Some confusion has arisen about a possible circumplanetary disk in the system PDS 70, which I wrote about recently (see Exoplanet Moons in Formation?, from June 7). A team led by led by Valentin Christiaens at Monash (Australia) presented evidence for the kind of disk...
Search Results:
We Are the Music: Reflections on Galactic Immensity
While I’m immersed in the mechanics of exoplanet detection and speculation about the worlds uncovered by Kepler, TESS and soon, the Roman Space Telescope (not to mention what’s coming with Extremely Large Telescopes), I’m daunted by a single fact. We keep producing...
Habitability around F-class Stars
Some years back I read a science fiction story in which the planet where the action took place orbited an F-class star. That was sufficiently odd to get my attention, and I began to pay attention to these stars, which represent on the order of 3 percent of all stars...
Our Earliest Ancestor Appeared Soon After Earth Formed
Until we learn whether or not life exists on other planets, we extrapolate on the basis of our single living world. Just how long it took life to develop is a vital question, with implications that extend to other planetary systems. In today's essay, Alex Tolley...
Forbidden Worlds? Theory Clashes with Observation
Back before we knew for sure there were planets around other stars, the universe seemed likely to be ordered. If planet formation was common, then we'd see systems more or less like our own, with rocky inner worlds and gas giants in outer orbits. And if planet...
The “Habitability” of Worlds (Part I)
Dave Moore is a Centauri Dreams regular who has long pursued an interest in the observation and exploration of deep space. He was born and raised in New Zealand, spent time in Australia, and now runs a small business in Klamath Falls, Oregon. He counts Arthur C....
A Liquid Water Mechanism for Cold M-dwarf Planets
A search for liquid water on a planetary surface may be too confining when it comes to the wide range of possibilities for supporting life. We see that in our own Solar System. Consider the growing interest in icy moons like Europa and Enceladus, where there is no...
What We’re Learning about TRAPPIST-1
It’s no surprise that the James Webb Space Telescope’s General Observers program should target TRAPPIST-1 with eight different efforts slated for Webb’s first year of scientific observations. Where else do we find a planetary system that is not only laden with seven...
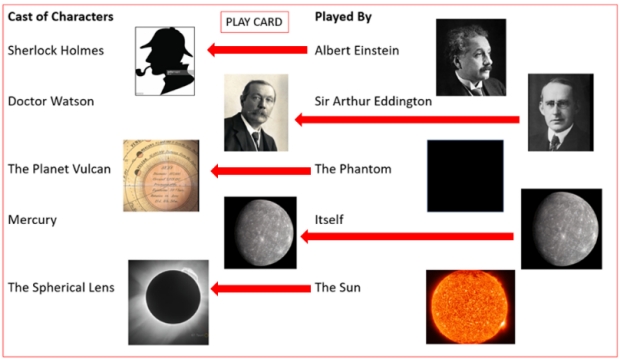
Part II: Sherlock Holmes and the Case of the Spherical Lens: Reflections on a Gravity Lens Telescope
Aerospace engineer Wes Kelly continues his investigations into gravitational lensing with a deep dive into what it will take to use the phenomenon to construct a close-up image of an exoplanet. For continuity, he leads off with the last few paragraphs of Part I, which...
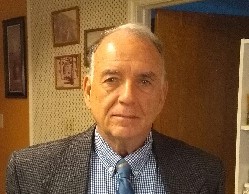
Sherlock Holmes and the Case of the Spherical Lens: Reflections on a Gravity Lens Telescope (Part I)
A growing interest in JPL's Solar Gravitational Lens mission here takes Wes Kelly on an odyssey into the past. A long-time Centauri Dreams contributor, Wes looks at the discovery of gravitational lensing, which takes us back not only to Einstein but to a putative...