by Paul Gilster | Jun 14, 2024 | Exoplanetary Science |
Great observatories work together to stretch the boundaries of what is possible for each. Data from the Chandra X-ray Observatory were used in tandem with the James Webb Space Telescope, for example, to observe the death of a star as it was consumed by a black hole. JWST’s infrared look at this Tidal Disruption Event (TDE) helped show the structure of stellar debris in the accretion disk of the black hole, while Chandra charted the high-energy processes at play in the cataclysmic event.
Or have a look at the image below, combining X-ray and infrared data from these two instruments along with the European Space Agency’s XMM-Newton, the Spitzer Space Telescope and optical data from Hubble and the European Southern Observatory’s New Technology Telescope to study a range of targets.
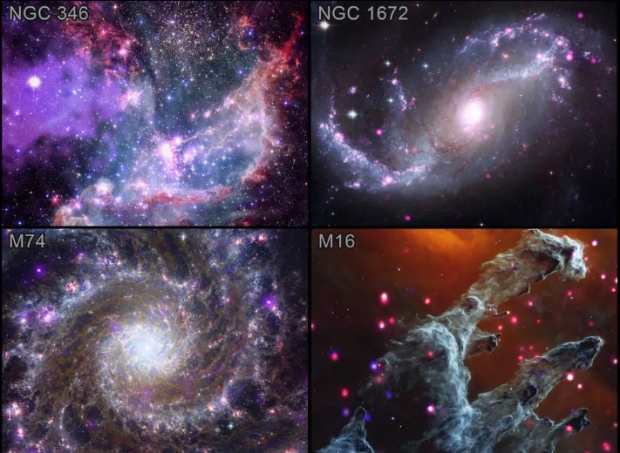
Image: Four composite images deliver dazzling views from NASA’s Chandra X-ray Observatory and James Webb Space Telescope of two galaxies, a nebula, and a star cluster. Each image combines Chandra’s X-rays — a form of high-energy light — with infrared data from previously released Webb images, both of which are invisible to the unaided eye. Data from NASA’s Hubble Space Telescope (optical light) and retired Spitzer Space Telescope (infrared), plus the European Space Agency’s XMM-Newton (X-ray) and the European Southern Observatory’s New Technology Telescope (optical) is also used. These cosmic wonders and details are made available by mapping the data to colors that humans can perceive. Credit: X-ray: Chandra: NASA/CXC/SAO, XMM: ESA/XMM-Newton; IR: JWST: NASA/ESA/CSA/STScI, Spitzer: NASA/JPL/CalTech; Optical: Hubble: NASA/ESA/STScI, ESO; Image Processing: L. Frattare, J. Major, and K. Arcand.
Working at multiple wavelengths obviously pays dividends, and with Chandra in the news because of proposed budget cuts, it’s worth noting that its observations have a role to play in the search for habitable worlds in our own stellar neighborhood. Currently the observatory is being used in conjunction with XMM-Newton in an ongoing exploration of habitability in terms of radiation. Which stars close enough to Earth for us to image planets in their habitable zones are also benign in terms of the radiation bath to which these same planets would be exposed?
High levels of ultraviolet and X-ray radiation can break chemical bonds and damage DNA in biological systems, not to mention their effect in stripping away planetary atmospheres. Thus ionizing radiation becomes a key factor in habitability in the kind of planets whose atmospheres we are first going to be able to study, those orbiting nearby M-dwarfs where the habitable zone is dauntingly close to the parent star.
The new study is led by Breanna Binder (California State Polytechnic University), who told the American Astronomical Society’s recent meeting in Madison, Wisconsin that of some 200 promising target stars, only a third have previously been examined by X-ray telescopes. Of these, many appeared quiet at visible wavelengths but quite active in X-ray emissions. Clearly we need to learn more about this critical variable.
With a generation of Extremely Large Telescopes soon to come online and future space observatories like the Habitable Worlds Observatory in the works, we will be able to take apart light from individual planets in search of biosignatures. Binder’s team will try to identify stars where the X-ray background is not dissimilar to Earth’s, so that the chances of life evolving within a protective atmosphere are enhanced. Ten days of Chandra observations along with 26 days of data from XMM-Newton were collected to home in on the X-ray behavior of some 57 nearby stars, ranging from 4.2 to 62 light years away.
This continuing effort is in a sense a shot in the dark, for while some of the stars are known to have planets, many are not. The necessary geometry of planetary transits means that many stars are likely orbited by habitable zone planets we can’t yet detect, and radial velocity methods are likewise not tuned for planets of this size. Edward Schwieterman (University of California Riverside) points to what comes next:
“We don’t know how many planets similar to Earth will be discovered in images with the next generation of telescopes, but we do know that observing time on them will be precious and extremely difficult to obtain. These X-ray data are helping to refine and prioritize the list of targets and may allow the first image of a planet similar to Earth to be obtained more quickly.”
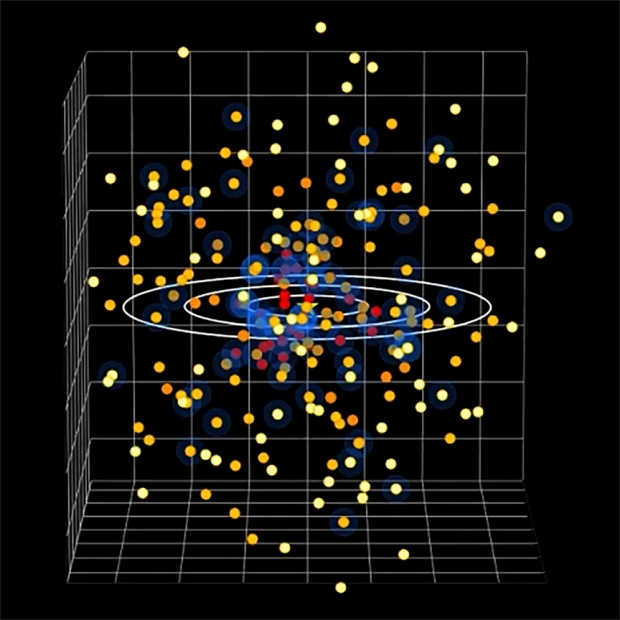
Image: A three-dimensional map of stars near the Sun that are close enough to Earth for planets in their habitable zones to be directly imaged using future telescopes. Planets in their stars’ habitable zones likely have liquid water on their surfaces. A study with Chandra and XMM-Newton of some of these stars (shown in blue haloes) indicates those that would most likely have habitable exoplanets around them based on a second condition — whether they receive lethal radiation from the stars they orbit. Credit: Cal Poly Pomona/B. Binder; Illustration: NASA/CXC/M.Weiss.
More on this continuing study as results become available. Meanwhile, note in relation to the budgetary woes Chandra is experiencing that a letter to NASA administrator Bill Nelson went out on June 6 from several U.S. senators and members of the House of Representatives urging that restrictions on its funding be rescinded. The letter bears all too few signatures, but it’s at least a step in the right direction. Quoting from it:
Premature termination of the Chandra mission would jeopardize this critical workforce, potentially driving talent to other countries. Chandra should serve as a bridge to a promising future in high energy astrophysics at NASA, including the development of its eventual flagship-scale successor, as recommended by the 2020 Decadal Survey in Astronomy and Astrophysics.
We strongly urge NASA to maintain full FY25 funding for the Chandra mission at the $68.7 million level, as outlined in NASA’s FY24 budget request, and to halt plans for significant reductions in FY25 until Congress determines Chandra’s appropriations. The proposed budget cuts would cause damage to U.S. leadership in high energy astrophysics and prematurely end the mission of a national treasure whose most significant discoveries may still be ahead.
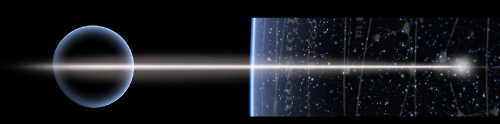
by Paul Gilster | Mar 23, 2024 | Exoplanetary Science |
Europa Clipper is scheduled to launch on October 10, with arrival at Jupiter in 2030. That will keep subsurface oceans on our minds as we tangle with the problems of analyzing water locked under kilometers of ice. Some moons, of course, help us out. Enceladus spews watery materials into space through cracks in its crust, making flybys through its geysers a possibility for snagging samples. Europa Clipper may find further evidence of the much less dramatic plume activity that has been spotted on Europa. Clipper’s SUrface Dust Analyzer (SUDA) would prove vital in such analysis.
If cellular material is found in an ice grain snared from an orbital pass, would we be able to detect it? The answer may be found in laboratory work with a common bacteria that thrives in the waters off Alaska. As explained in new work out of the University of Washington and the Freie Universität Berlin, the bacterium Sphingopyxis alaskensis is made to order for such studies. It is smaller than Escherichia coli (frequently used in experimental work), thrives in cold conditions and needs little by way of nutrients to stay alive. If you want to approximate an icy moon’s ocean environment and experiment here with how life might operate there, Sphingopyxis alaskensis is just the ticket.
Under the direction of lead author Fabian Klenner (University of Washington), a research team has been pondering how material ejected from a moon like Enceladus might be detected by a spacecraft passing through a plume. The benefits of analyzing materials from subsurface oceans without landing are obvious, although Enceladus presents a much more benign environment for surface investigation than Europa, given the vents in its south polar crust and its much lower radiation exposure. And even if you find a way to shield a Europa lander, how to drill through all that ice?
The challenge the team has overcome is how to simulate ice grains impacting a scientific instrument at between four and six kilometers per second. The proposed solution: Fire a beam of liquid water into a vacuum. As it transforms into droplets, deploy a laser beam to excite the droplets and subject the result to mass spectral analysis, which identifies chemical and isotopic compositions. Europa Clipper’s SUrface Dust Analyzer will be able to detect ions with negative charges, even fatty acids and lipids. Thus the prospect of flying through a Europan plume looms as an exciting astrobiological possibility.
The genesis of this work comes from the authors’ analysis of Enceladus, in which they examined the evidence for phosphates in its ocean. The moon appears to contain the organic materials and the energy resources needed to support some form of life. Indeed, that work, developed in a 2023 paper, found that phosphate is present in the Enceladus ocean at levels at least 100 times higher, and perhaps much higher still, than in Earth’s oceans. Phosphates are vital to life on Earth, and this work was the first report of direct evidence of phosphorus on an icy moon. As Klenner noted then:
“By determining such high phosphate concentrations readily available in Enceladus’ ocean, we have now satisfied what is generally considered one of the strictest requirements in establishing whether celestial bodies are habitable.”
So we have an ocean laden with recently discovered dissolved phosphates, one with previously known dissolved carbonates and a variety of other carbon-containing compounds and salts. Bacterial cells found here, if encased in a liquid membrane, might well rise through cracks in the ice shell even as exposure to the vacuum would cause the waters directly below to boil. Gas bubbles bursting at the surface would allow cellular material to become incorporated into ice grains being blown outward by the plume. That’s a scenario that makes for possible life discovery.
As the paper notes:
Although an extraterrestrial biosphere might use different biochemistry, it is logical to assume an aqueous-based ecosystem with access to molecular building blocks common in our Solar System [e.g., amino acids, aliphatic hydrocarbons, sugars, nitrogen heterocycles, and others commonly found in meteorites (69)] would likely use and modify the concentrations of those molecules in ways that would deviate from an abiotic system (57, 70).
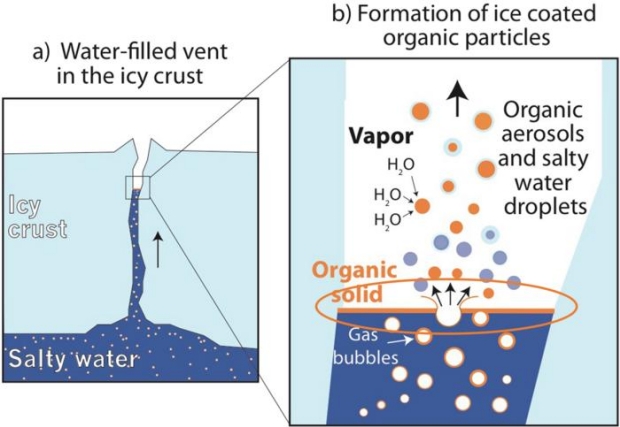
Image: The left panel shows the kilometers-thick icy crust believed to encapsulate Saturn’s moon Enceladus. Filling the crack is salty water with a proposed thin layer (shown orange) at its surface. The right panel shows that as gas bubbles rise and pop, they combine with organic material and get lofted into the spray. Credit: Postberg et al. (2018)/Nature.
The experimental results in the recent lab work on Sphingopyxis alaskensis are encouraging. The above mechanism would produce enough material in individual ice grains for cellular matter to be detected by instruments like those aboard Europa Clipper and future missions to icy moons. Indeed, a single ice grain would do the trick. Says Klenner:
“For the first time we have shown that even a tiny fraction of cellular material could be identified by a mass spectrometer onboard a spacecraft. Our results give us more confidence that using upcoming instruments, we will be able to detect lifeforms similar to those on Earth, which we increasingly believe could be present on ocean-bearing moons.”
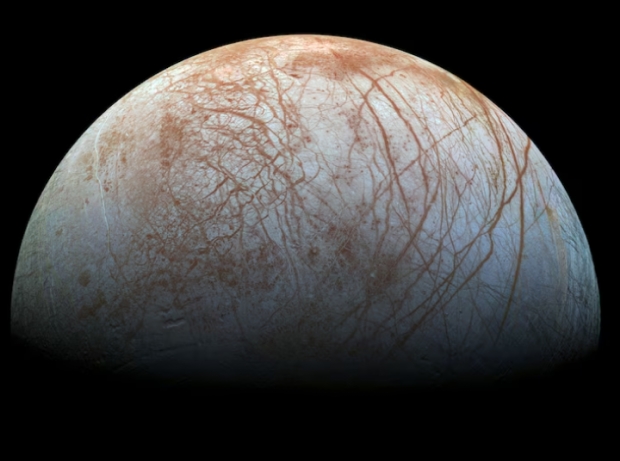
Image: Won’t it be great when we can move past the old Galileo imagery of Europa for fresh images taken by Europa Clipper? This Galileo image shows red streaks across the surface of this smallest of Jupiter’s four large moons. New research shows that one of the instruments destined for the Clipper mission could find traces of a single cell in a single ice grain ejected from the moon’s interior. Credit: NASA/JPL/Galileo.
The paper is Klenner et al., “How to identify cell material in a single ice grain emitted from Enceladus or Europa,” Science Advances, Vol 10, Issue 12 (22 March 2024). Abstract. The paper on phosphates on Enceladus is Postberg et al., “Detection of phosphates originating from Enceladus’s ocean,” Nature 618 (14 June 2023) 489-493 (abstract).
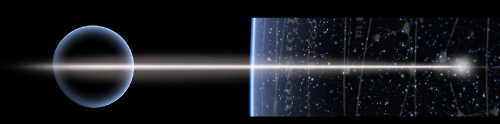
by Paul Gilster | Mar 15, 2024 | Exoplanetary Science |
Just a few weeks ago I wrote about stellar interactions, taking note of a concept advanced by scientists including Ben Zuckerman and Greg Matloff that such stars would make for easier interstellar travel. After all, if a star in its rotation around the Milky Way closes to within half a light year of the Sun, it’s a more feasible destination than Alpha Centauri. Of course, you have to wait for the star to come around, and that takes time. Zuckerman (UCLA), working with Bradley Hansen, has written about the possibility that close encounters are when a civilization will attempt such voyages.
I have a further idea along the lines of motion through the galaxy and its advantages to explorers, and it’s one that may not require tens of thousands of years of waiting. We’d like to get to another star system because we’re interested in the planets there, so what if an interstellar planet nudges into nearby space? I’ll ignore Oort Cloud perturbations and the rest to focus on a ‘rogue’ or ‘free-floating’ planet as the target of a probe, and ask whether we may not already have some of these in nearby space.
After all, finding free-floating planets – and I’m now going to start calling them FFPs, because that’s what appears in scientific papers on the matter – are hard to find. There being no reflected starlight to look for, the most productive way is to pick them out by their infrared signature, which means finding them when they’re relatively young. This is what Núria Miret Roig (University of Vienna) and team did a couple of years ago, working with data from the Very Large Telescope and other sources. Lo and behold, over one hundred FFPs turned up, all of them infants and still warm.
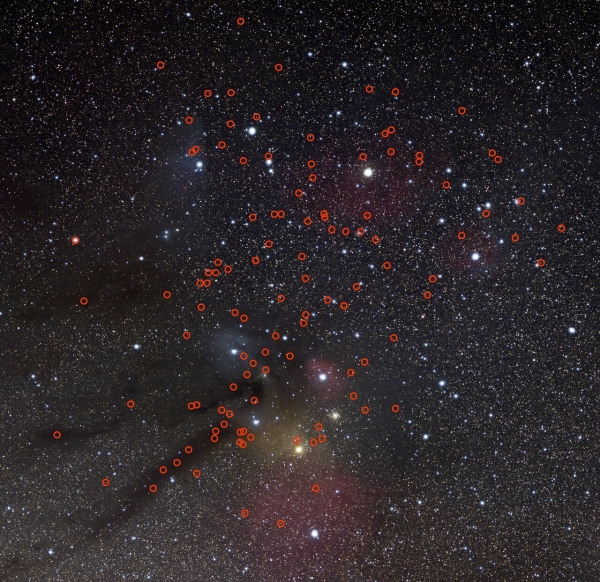
Image: The locations of 115 potential FFPs [free-floating planets] in the direction of the Upper Scorpius and Ophiuchus constellations, highlighted with red circles. The exact number of rogue planets found by the team is between 70 and 170, depending on the age assumed for the study region. This image was created assuming an intermediate age, resulting in a number of planet candidates in between the two extremes of the study. Credit: ESO/N. Risinger (skysurvey.org).
But young FFPs are most likely to be found in star-forming regions, two of which (in Scorpius and Ophiuchus) were subjected to Miret Roig and team’s searches. What’s likely to amble along in our rather more sedate region is an FFP with enough years on it to have cooled down. The WISE survey (Wide-Field Infrared Survey Explorer) showed how difficult it is to pin down red dwarfs in the neighborhood, although it can be done. But even there, when you get down to L- and T-class brown dwarfs, uncertainty persists about whether you can find them. With planets the challenge is even greater.
Sometimes FFPs are found through microlensing toward the galactic core, but I don’t think we can rely on that method for finding a population of such worlds within, say, half a light year. Nonetheless, Miret Roig is not alone in pointing out that “there could be several billions of these free-floating planets roaming freely in the Milky Way without a host star.” Indeed, that number could be on the low side given what we’re learning about how these objects form. Given the excitement over ‘Oumuamua and other interstellar interlopers that may appear, I’m surprised that there hasn’t been more attention paid to how we might detect planet-sized objects near our system.
The ongoing search for Planet 9 demonstrates how difficult finding a planet outside the ecliptic can be right here at home. While pondering the best way to proceed, I’ll divert the discussion to rogue planet formation, which has always been central to the debate. Are the processes rare or common, and if the latter, do most stellar systems including our own, have the potential for ejecting planets? The last two decades of study have been productive, as we have refined our methods for modeling this process.
Recent work on the Trapezium Cluster in the Orion Nebula shows us how the catalog of FFPs is growing. The Trapezium Cluster is helpfully located out of the galactic plane, and there is a molecular cloud behind it that reduces the problems posed by field stars. I was startled to learn about this study (conducted at the European Space Agency’s ESTEC facility in the Netherlands by Samuel Pearson and Mark J McCaughrean) because of the sheer number of FFPs it turned up. Some 540 FFP candidates are identified here, ranging in mass from 0.6 to 13 Jupiter masses, although the range is an estimate based on the age of the cluster and our current models of gas giant evolution.
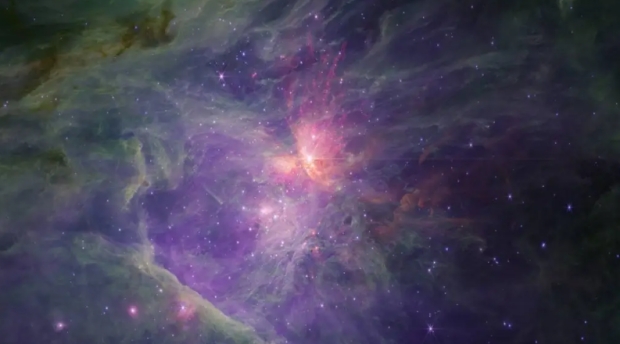
Image: A total of 712 individual images from the Near Infrared Camera on the James Webb Space Telescope were combined to make this composite view of the Orion Nebula and the Trapezium Cluster. Credit: NASA, ESA, CSA/Science leads and image processing: M. McCaughrean, S. Pearson, CC BY-SA 3.0 IGO.
What stopped me cold about this work is that among the 540 candidate FFPs, 40 are binaries. Two free-floating planets moving together without a star, and enough of them that we have to learn a new term: JuMBOs, for Jupiter-mass binary objects. How does that happen? There are even two triple systems in the data. Digging into the paper:
…we can compare their statistical properties…with higher-mass systems. The JuMBOs span the full mass range of our PMO [planetary-mass object] candidates, from 13 MJup down to 0.7 MJup. They have evenly distributed separations between ∼25–390 au, which is significantly wider than the average separation of brown dwarf-brown dwarf binaries which peaks at ∼ 4 au [42, 43]. However, as our imaging survey is only sensitive to visual binaries with separations > 25 au, we can not rule out an additional population of JuMBOs with closer orbits. For this reason we take 9% as a lower bound for the PMO multiplicity fraction. The average mass ratio of the JuMBOs is q = 0.66. While there are a significant number of roughly equal-mass JuMBOs, only 40% of them have q ≥ 0.8. This is much lower than the typical mass ratios for brown dwarfs, which very strongly favour equal masses.
That last line is interesting. Our FFP binary systems tend to have planets of distinctly different masses, which implies, according to the authors, that if the JuMBOs formed through core collapse and fragmentation – like a star – “then there must be some fundamental extra ingredient involved at these very low masses.” But the binary systems here go well below the mass where this formation method was thought to work. That opens up the ‘ejection’ hypothesis, with the planets forming in a circumstellar disk only to be ejected by gravitational interactions. So note this:
In either case, however, how pairs of young planets can be ejected simultaneously and remain bound, albeit weakly at relatively wide separations, remains quite unclear. The ensemble of PMOs and JuMBOs that we see in the Trapezium Cluster might arise from a mix of both of these “classical” scenarios, even if both have significant caveats, or perhaps a new, quite separate formation mechanism, such as a fragmentation of a star-less disk is required.
Ejection is a rational thing to look at considering that gravitational scattering is a well-studied process and may well have occurred in the early days of our own system. On the other hand, in star-forming regions like Trapezium the nascent systems are so young that this scenario may be less likely than the core-collapse model, in which the process is similar to star formation as a molecular cloud collapses and fragments. The open question is whether a scenario like this, which seems to work for brown dwarfs, is also applicable to considerably smaller FFPs in the Jupiter-mass range.
In any case, it seems unlikely that binary planets could survive ejection from a host system. As co-author Pearson puts it, “Nine percent is massively more than what you’d expect for the planetary-mass regime. You’d really struggle to explain that from a star formation perspective…. That’s really quite puzzling.”
All of which triggered a new paper from Fangyuan Yu (Shanghai Jiao Tong University) and Dong Lai (Cornell University), which takes an entirely different tack when it comes to formation of binary FFPs:
The claimed detection of a large fraction (9 percent) of JuMBOs among FFPs (Pearson & McCaughrean 2023) seems to suggest that core collapse and fragmentation (i.e. scaled-down star formation) channel plays an important role in producing FFPs down to Jupiter masses, since we do not expect the ejection channel to produce binary planets. On the other hand, (Miret-Roig et al. 2022) suggested that the observed abundance of FFPs in young star clusters significantly exceeds the core collapse model predictions, indicating that ejections of giant planets must be frequent within the first 10 Myr of a planetary system’s life.
Yu and Lai look at close stellar flybys as a contributing factor to FFP binary formation. If we’re talking about dense young star clusters, encounters between stars should be frequent, and there has been at least one study advancing the idea that bound binary planets could be the result of such flybys. Yu and Lai model two-planet systems to study the effects of a flyby on single and double-planet systems. Will an FFP result from a close flyby? A binary FFP? Or will the flyby star contribute a planet to the system it encounters?
These numerical experiments yield interesting results: The production rate of binary pairs of FFPs caused by stellar flybys is always less than 1 percent in their modeling, even when parameters are adjusted to make for tightly packed stellar systems. Directly addressing the JWST work in Trapezium and the large number of JuMBOs found there, Yu and Lai deduce that they cannot be caused by flybys, and because ejection scenarios are so unlikely, they see “a scaled-down version of star formation” at work “via fragmentation of molecular cloud cores or weakly-bound disks or pseudo-disks in the early stages of star formation.”
The matter remains unresolved, producing much fodder for future observations and debate. And while we figure out how to detect free-floating planets that may already be far closer than Proxima Centauri, we can create science fictional scenarios of journeys not just to a single rogue planet, but to a binary or even a triple system cohering despite the absence of a central star. I can only imagine how much Robert Forward, the man who gave us Rocheworld, would have enjoyed working with that.
The paper is Pearson & McCaughrean, “Jupiter Mass Binary Objects in the Trapezium Cluster” (preprint). The Miret-Roig paper is “A rich population of free-floating planets in the Upper Scorpius young stellar association,” published online at Nature Astronomy 22 December 2021 (abstract). The Fangyuan Yu & Dong Lai paper is, “Free-Floating Planets, Survivor Planets, Captured Planets and Binary Planets from Stellar Flybys,” submitted to The Astrophysical Journal (preprint).
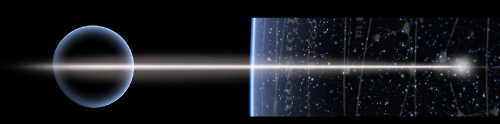
by Paul Gilster | Mar 6, 2024 | Exoplanetary Science |
Planet formation is a fascinating subtopic of the exoplanet hunt, and it may just have produced the first exoplanet detection in data that go back as far back as 1981, though the event in question has never been confirmed as being caused by a planet. I learned this through a paper sent me recently by Jean Schneider (Observatoire de Paris), who along with colleague Danielle Briot wrote about the early days of transit searches in a chapter for the Handbook of Exoplanets (Springer, 2018).
I want to dig deeper into that chapter in a later post, but for now, I note that the planet Beta Pictoris b, discovered in 2008 and orbiting an infant star 63 light years from Earth, may have transited in 1981, according to subsequent papers on the matter. The debris disk around the primary has long fascinated astronomers and it has been investigated for the possible presence of comet-like bodies and subjected to direct imaging searches, which revealed Beta Pictoris b and confirmed it in 2009. But the 1981 data show light variations that could be interpreted as a transit.
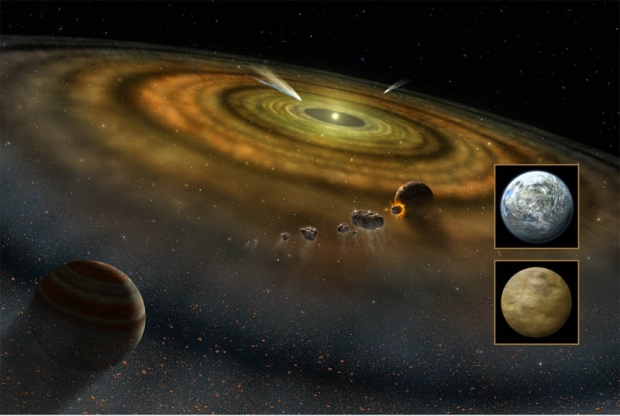
Image: Various planet formation processes, including exocomets and other planetesimals, around Beta Pictoris, a very young type A V star. Credit: NASA/FUSE/Lynette Cook.
Testing the matter involved a nano-satellite called PicSat designed by astronomers at the Paris-Meudon observatory to look for a possible 2018 transit. Unfortunately, the mission failed when communications were lost. As Schneider and Briot write:
If the transit is confirmed in the near future, we could obtain better observations of the following transit in 2053, when the period will be known more accurately and when we could use very large and extraordinary outstanding future instruments. So, will Beta Pictoris b win the title of the first detected exoplanet?
The massive debris disk at Beta Pictoris is asymmetric (likely the result of perturbations by another star), and seen edge-on from Earth. But the beauty of the circumstellar disks out of which planets form is that if we peer into planet-forming regions, we can find them oriented in all kinds of ways, as we learn in a new series of images from the European Southern Observatory’s Very Large Telescope. They’re part of a survey of how planetary systems form, and they mark a transition between the intense study of individual star systems to a broad swath of planets and stars. As Christian Ginski (University of Galway, Ireland) puts it, “We’ve gone from the intense study of individual star systems to this huge overview of entire star-forming regions.”
Let’s home in on a single system to begin with to ponder how much we can learn from these disks.
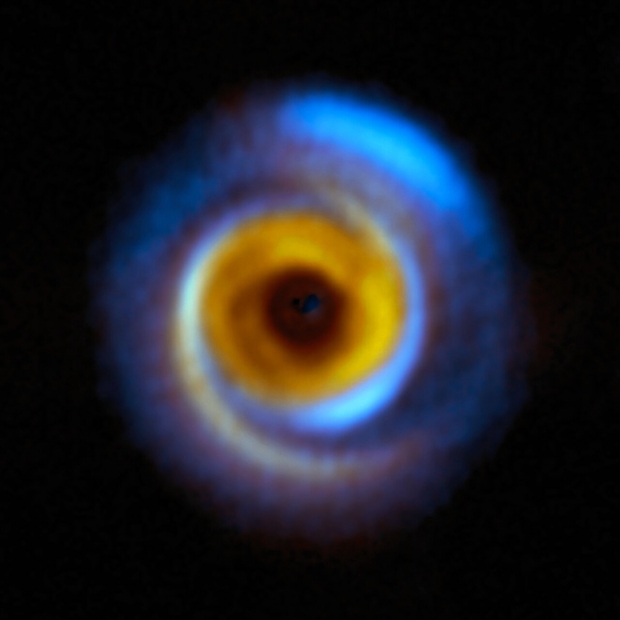
Image: This composite image shows the MWC 758 planet-forming disc, located about 500 light-years away in the Taurus region, as seen with two different facilities. The yellow colour represents infrared observations obtained with the Spectro-Polarimetric High-contrast Exoplanet REsearch (SPHERE) instrument on ESO’s Very Large Telescope (VLT). The blue regions on the other hand correspond to observations performed with the Atacama Large Millimeter/submillimeter Array (ALMA), in which ESO is a partner. These facilities allow astronomers to map how dust is distributed around this and other stars in different but complementary ways. SPHERE captures light from the host star that has been scattered by the dust around it, whereas ALMA registers radiation directly emitted by the dust itself. These observations combined help astronomers understand how planets may form in the dusty discs surrounding young stars. Credit: ESO/A. Garufi et al.; R. Dong et al.; ALMA (ESO/NAOJ/NRAO).
The new ESO imagery takes in numerous young stars like this one. What leaps out here is the wide range of formation scenarios. Working with the star-forming regions at Orion, Taurus and Chamaeleon I, an international team of astronomers investigated 86 stars using SPHERE, the Spectro-Polarimetric High-contrast Exoplanet REsearch instrument mounted on the VLT. The quality of the imagery is attributable to SPHERE’s adaptive optics, which allows disks to be imaged around stars down to about half the Sun’s mass while correcting for the distortions produced by looking through the Earth’s atmosphere. The use of ALMA, the Atacama Large Millimeter/submillimeter Array, helped quantify the amount of dust in these systems.
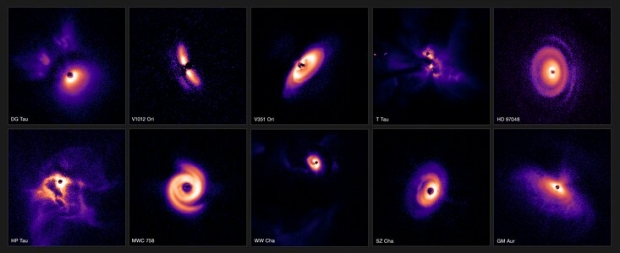
Image: This research brings together observations of more than 80 young stars that might have planets forming around them in spectacular discs. This small selection from the survey shows 10 discs from the three regions of our galaxy observed in the papers. V351 Ori and V1012 Ori are located in the most distant of the three regions, the gas-rich cloud of Orion, some 1600 light-years from Earth. DG Tau, T Tau, HP Tau, MWC758 and GM Aur are located in the Taurus region, while HD 97048, WW Cha and SZ Cha can be found in Chamaeleon I, all of which are about 600 light-years from Earth. The discs have been scaled to appear roughly the same size in this composition. Credit: ESO/C. Ginski, A. Garufi, P.-G. Valegård et al.
The imagery is stunning. Per-Gunnar Valegård (University of Amsterdam), who led the work on Orion, noted “It is almost poetic that the processes that mark the start of the journey towards forming planets and ultimately life in our own Solar System should be so beautiful.” I think we can drop that word ‘almost’ as we are reminded that the sheer wonder of celestial vistas at ever increasing scales is what drew many of us into astronomy at an early age. We’re a long way from those grainy Palomar images of Jupiter and Saturn that I acquired decades ago at Adler Planetarium in Chicago.
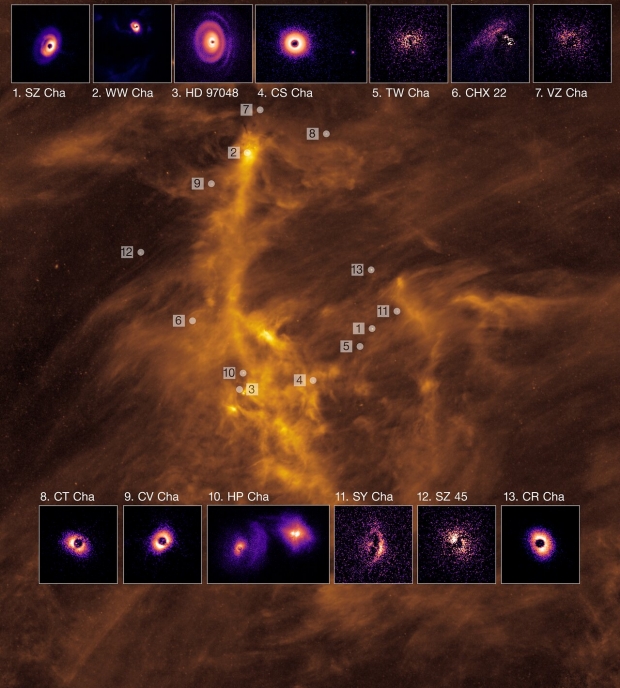
Image: Planet-forming discs around young stars and their location within the gas-rich cloud of Chamaeleon I, roughly 600 light-years from Earth. The team observed 20 stars in the Chamaeleon I region, detecting discs around 13. The background image shows an infrared view of Chamaeleon I captured by the Herschel Space Observatory. Credit: ESO/C. Ginski et al.; ESA/Herschel.
Meanwhile, in a separate investigation of the young star T Chamaeleontis, we gain insights into planet formation in its latter stages. Here we have the James Webb Space Telescope to thank. Naman Bajaj (University of Arizona) and Uma Gorti (SETI Institute) have been able to extract data on the four lines of the noble gases neon (Ne) and argon (Ar), with the neon detection showing processes that are occurring over an extended area. The observations show dispersing gasses from a planet-forming disk that is in its latter stages, completing the formation process. These ‘winds’ seem to be driven by stellar photons or, according to Bajaj, by the magnetic field within the disk itself.
The find is significant, says Richard Alexander (University of Leicester):
“We first used neon to study planet-forming discs more than a decade ago, testing our computational simulations against data from Spitzer, and new observations we obtained with the ESO VLT. We learned a lot, but those observations didn’t allow us to measure how much mass the discs were losing. The new JWST data are spectacular, and being able to resolve disc winds in images is something I never thought would be possible. With more observations like this still to come, JWST will enable us to understand young planetary systems as never before.”
The paper on the history of transit studies is Briot & Schneider, “Prehistory of Transit Searches,” in Handbook of Exoplanets, 2nd edition.
The papers on the SPHERE work are Ginski et al., “The SPHERE view of the Chamaeleon I star-forming region: The full census of planet-forming disks with GTO and DESTINYS programs”, Astronomy & Astrophysics (abstract).
And two other papers in the same issue: Garufi et al., “The SPHERE view of the Taurus star-forming region: The full census of planet-forming disks with GTO and DESTINYS programs,” (abstract) and Valegard et al., “Disk Evolution Study Through Imaging of Nearby Young Stars (DESTINYS): The SPHERE view of the Orion star-forming region” (abstract).
The paper on T Chamaeleontis is Bajaj et al., “JWST MIRI MRS Observations of T Cha: Discovery of a Spatially Resolved Disk Wind,” The Astronomical Journal Vol. 167, No. 3 (4 March 2024), 127 (abstract).
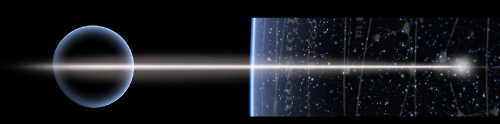
by Paul Gilster | Feb 23, 2024 | Exoplanetary Science |
I’m going to start in the Kuiper Belt this morning before going further out, because the news that the Belt may extend much further than expected reminds us of the nature of exploration. The New Horizons spacecraft, well beyond Pluto’s orbit and approaching 60 AU from the Sun, is finding more dust than expected. Our theoretical models didn’t see that coming. In fact, the dust produced by collisions between Kuiper belt objects was thought to decline as we approached the Belt’s outer edge.
So just where is that outer edge? It had been pegged around 50 AU but now looks more like 80 AU, if not further out, a finding corroborated by the fact that New Horizons scientists have used Earth-based resources like the Subaru Telescope in Hawaii to find numerous KBOs beyond the assumed boundary. Is this a new population of Solar System objects, or are we actually seeing something more mundane, such as radiation pressure pushing inner belt dust further out than would be expected? It takes patient observation to decide, and to re-shape our notions according to hard data.
Which gets me into exoplanet territory, and specifically our understanding of red dwarf stars. Theory is always malleable and yields to observation which, in turn, re-energizes theory. Michaël Gillon (University of Liége), who is among other things the discoverer of the TRAPPIST-1 system, made this point in a recent email exchange. He was responding to my article What We Know Now about TRAPPIST-1 (and what we don’t) with a much needed note of caution. The question of whether rocky planets orbiting M-dwarf stars can retain atmospheres is one of the hottest controversies going. Observations, says Gillon, will tell the tale, not theory, no matter how elegant the latter. After all, we now have JWST, and a new generation of telescopes already under construction to help.
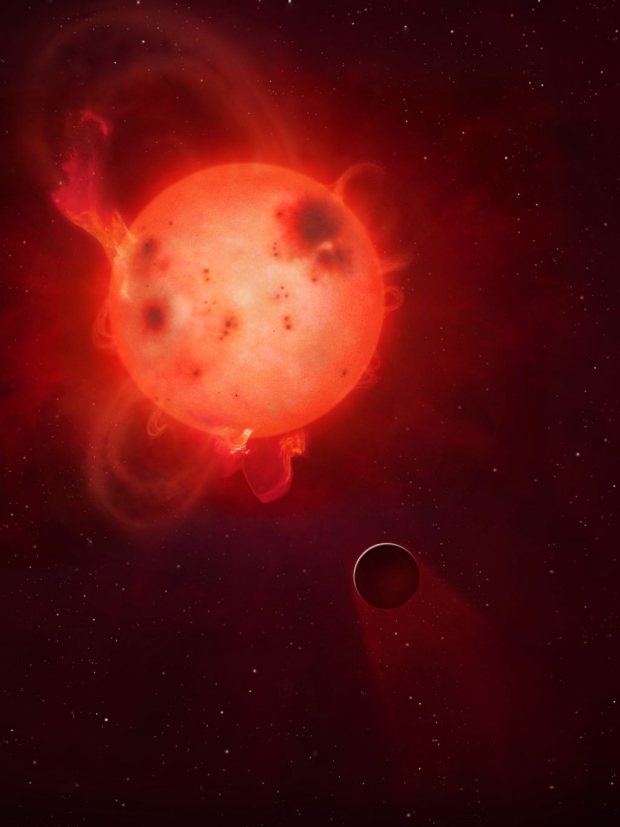
Image: An artist’s concept of Kepler-438b, shown here in front of its violent parent star, a red dwarf. It is regularly irradiated by huge flares of radiation, which could render the planet uninhabitable and possibly strip it of its atmosphere entirely. A variety of mechanisms for depleting the atmosphere of such a planet are now under discussion, using a wide range of models. Image credit: Mark A Garlick / University of Warwick.
On that score, it’s useful to look at a paper that Gillon suggests in his email, a study by Ignasi Ribas and collaborators that appeared in Astronomy & Astrophysics in 2016. The paper does a deep dive into the question of the habitability of Proxima Centauri b, that tantalizingly close Earth-mass planet in the star’s habitable zone. A key issue is whether the extreme dosing of X-ray and ultraviolet radiation such a planet would suffer would compromise a young atmosphere or prevent its existence at all.
There is a lot going on in the Ribas paper, but Gillon pointed me to its discussion of what we might learn from the lesson of our own Earth. Here the essential fuzziness of theory manifests itself, as Ribas and team point out how many uncertainties exist in our estimates of volatile loss, including the telling line “…they rely on complex models that were never confronted to actual observations of massive escape.” Even more telling is the lack of sufficient information about what might prevent such escape:
None of the available models include all the mechanisms controlling the loss rate, for example, the photochemistry of the upper atmosphere and its detailed interaction with the wavelength-dependent stellar emission, non-LTE [Non-Local Thermodynamic Equilibrium] cooling processes, and an accurate description of the outflow beyond the exobase where hydrodynamics no longer apply. Some key data are not known, like the intrinsic planetary magnetic moment, now and in the past, the detailed evolution of the atmospheric composition, of the high-energy spectrum and of stellar wind properties.
Nor do we have hard data on these things even now. Note the term ‘non-LTE cooling’ above. An LTE system is one in thermal equilibrium, maintaining a single temperature. Poking around in the literature, I learn that the lack of thermal equilibrium involves processes that have to be carefully measured to build an accurate profile of an atmosphere, with ramifications for any discussion of its long-term survival. In the absence of such data, it’s telling that other factors remain unknown, including local magnetic conditions and the actual properties of the stellar wind affecting the planet. And we have no good modeling for how volatiles may be distributed between the atmosphere of an M-dwarf planet and the internal processes that can replenish it.
Thus the Ribas paper, although eight years old, remains pertinent to this ongoing discussion, as scientists attack volatile retention in such systems. The authors point out that the protoplanets that built up the early Earth were exposed to a young Sun that was blasting our planet with X-rays, ultraviolet and stellar wind conditions that may equal, and perhaps surpass, what occurred at Proxima b. Note this (italics mine):
The XUV irradiation and stellar wind on the proto-Earth was therefore comparable, and possibly higher, than that of Proxima b. Proxima b spent 100–200 Myr in runaway before entering the HZ, which is longer than the runaway phases experienced by the proto-Earth by a small factor only (<10). Models predict that early Earth suffered massive volatile losses: hydrodynamic escape of hydrogen dragging away heavier species and non thermal losses under strong stellar wind exposure and CMEs (Lammer et al. 2012). Nonetheless, no clear imprint of these losses is found in the present volatile inventory.
The authors point out, then, that geochemical evidence alone shows us no signs of significant depletion of Earth’s inventory of volatiles, which can lead to the possibility that volatile loss was extremely limited under conditions that some of our models would suggest should deplete them radically. If this analysis is correct, then the idea that the planets of red dwarf stars will likely be barren rock stripped of atmospheres is questionable. I come back to Gillon’s point. We’re only going to know from observation, just as we can only know about the extent of the Kuiper Belt through hard data.
Now comes a new paper from Ofer Cohen (University of Massachusetts) and colleagues. Writing in The Astrophysical Journal, the authors again address the TRAPPIST-1 question, this time with a new twist. They’re looking at electric currents that would be produced in the ionosphere of TRAPPIST-1e, a planet that may be in the star’s habitable zone. The question is whether such currents would produce atmospheric heating that would contribute to dissipating the atmosphere entirely.
So this is another stripping mechanism to consider, one produced by the planet’s upper atmosphere encountering the star’s changing magnetic field as the planet proceeds along its orbit. The operative term is ‘Joule heating.’ I only received the paper this morning, so I won’t go too deeply into it. But my early reading suggests that the results from the models used in it point to serious atmospheric loss. This adds to earlier modeling involving the stellar wind and ionized upper atmosphere, some of this conducted by the same authors. The conclusion draws naturally from the modeling:
The JH [Joule heating] is the result of a dissipation of electric current, which is driven by the rapidly varying magnetic field along the planetary orbit. We estimate the JH energy flux on the exoplanet Trappist-1e as well as similar planets orbiting the Sun in close-in orbits. We find that the JH energy flux is larger than the anticipated EUV energy flux at the planet, and it may reach a few percent of the stellar constant energy flux. Such an intense heating could drive a strong atmospheric escape and could lead to a rapid loss of the atmosphere. Thus, the rapid orbital motion of short-orbit exoplanets may exhaust a significant portion of their atmospheres over time.
Again we find useful theories painting a landscape of possibilities. But it’s also true that we lack observational data on the properties of the stellar wind, its evolution over time, and the magnetic fields affecting the planet. The authors call attention to this fact:
VDJH [voltage-driven Joule heating] depends on the variations of the interplanetary magnetic field (IMF) strength along the planetary orbit. Such detailed IMF data are not available for exoplanets (some observations were made for the stellar wind interaction with the interstellar medium; e.g., Wood et al. 2021), nor it is available for short orbits around the Sun (limited data at specific locations are available from the Parker Solar Probe; Raouafi et al. 2023). Due to the lack of observational constraints, we must rely on models to estimate the relevant stellar wind conditions.
Thus energy output, stellar wind and magnetic field changes all factor into a model that suggests atmospheric escape and, like other models, is in need of confirmation with future instrumentation. We can only turn to such observation to begin to understand how diverse theories mesh. I think all the scientists involved in the study of planetary atmospheres around M-dwarfs would agree with this. And headlines in the popular media announcing barren rocks at TRAPPIST-1 are making ongoing investigations into settled science.
Getting too comfortable with theory can mislead us. Recently we saw that the astronomer Otto Struve proposed detecting Jupiter-class worlds in tight orbits around their host stars, only to have the suggestion ignored for decades because ‘hot Jupiters’ simply didn’t fit into then current thinking.
For that matter, nobody thought ‘super-Earths’ were likely, especially in the kind of numbers we’ve found them, nor ‘mini-Neptunes,’ and I doubt many were expecting tiny compact systems of numerous planets, like those Gillon identified at TRAPPIST-1. All in all, I appreciate Gillon’s reminder that patience and data gathering are needed as we explore the question of life around small red stars, an issue that is under deep study but has been by no means resolved. Perhaps the Habitable Worlds Observatory (Habex) will allow a definitive answer for TRAPPIST-1 in the not so distant future.
The Ribas paper is “The habitability of Proxima Centauri b I. Irradiation, rotation and volatile inventory from formation to the present,” Astronomy & Astrophysics 506 (2016), A111 (full text). The Cohen paper is “Heating of the Atmospheres of Short-orbit Exoplanets by Their Rapid Orbital Motion through an Extreme Space Environment,” The Astrophysical Journal 962 (16 February 2020), 157 (full text).
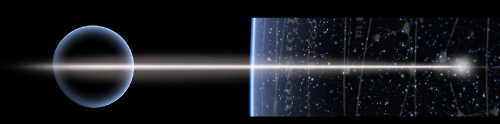
by Paul Gilster | Feb 14, 2024 | Exoplanetary Science |
Some things just run in families. If you look into the life of Otto Struve, you’ll find that the Russian-born astronomer was the great grandson of Friedrich Georg Wilhelm von Struve, who was himself an astronomer known for his work on binary stars in the 19th Century. Otto’s father was an astronomer as well, as was his grandfather. That’s a lot of familial energy packed into the study of the stars, and the Struve of most recent fame (Otto died in 1963) drew on that energy to produce hundreds of scientific papers. Interestingly, the man who was director at Yerkes and the NRAO observatories was also an early SETI advocate who thought intelligence was rife in the Milky Way.
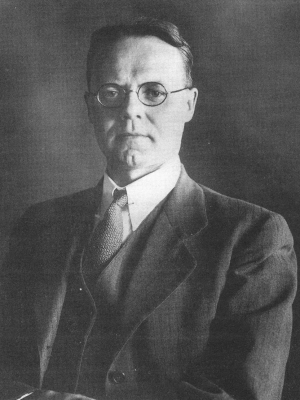
Of Baltic-German descent, Otto Struve might well have become the first person to discover an exoplanet, and therein hangs a tale. Poking around in the history of these matters, I ran into a paper that ran in 1952 in a publication called The Observatory titled “Proposal for a Project of High-Resolution Stellar Radial Velocity Work.” Then at UC Berkeley, Struve had written his PhD thesis on the spectroscopy of double star systems at the University of Chicago, so his paper might have carried more clout than it did. On the other hand, Struve was truly pushing the limits.
Image: Astronomer Otto Struve (1897-1963). Credit: Institute of Astronomy, Kharkiv National University.
For Struve was arguing that Doppler measurements – measuring the wavelength of light as a star moves toward and then away from the observer – might detect exoplanets, if they existed, a subject that was wildly speculative in that era. He was also saying that the kind of planet that could be detected this way would be as massive as Jupiter but in a tight orbit. I can’t call this a prediction of the existence of ‘hot Jupiters’ as much as a recognition that only that kind of planet would be available to the apparatus of the time. And in 1952, the idea of a Jupiter-class planet in that kind of orbit must have seemed like pure science fiction. And yet here was Struve:
…our hypothetical planet would have a velocity of roughly 200 km/sec. If the mass of this planet were equal to that of Jupiter, it would cause the observed radial velocity of the parent star to oscillate with a range of ± 0.2 km/sec—a quantity that might be just detectable with the most powerful Coudé spectrographs in existence. A planet ten times the mass of Jupiter would be very easy to detect, since it would cause the observed radial velocity of the star to oscillate with ± 2 km/sec. This is correct only for those orbits whose inclinations are 90°. But even for more moderate inclinations it should be possible, without much difficulty, to discover planets of 10 times the mass of Jupiter by the Doppler effect.
Struve suggested that binary stars would be a fertile hunting ground, for the radial velocity of the companion star would provide a “reliable standard of velocity.”
Imagine what would have happened if the discovery of 51 Pegasi (the work of Michel Mayor and Didier Queloz in 1995) had occurred in the early 1960s, when it was surely technically possible. Joshua Winn (Princeton University) speculates about this in his book The Little Book of Exoplanets (Princeton University Press, 2023). And if you start going down that road, you quickly run into another name that I only recently discovered, that of Kaj Aage Gunnar Strand (1907-2000). Working at Sproul Observatory (Swarthmore College) Strand announced that he had actually discovered a planet orbiting 61 Cygni in 1943. Struve considered this a confirmed exoplanet.
Now we’re getting deep into the weeds. Strand was using photometry, as reported in his paper “61 Cygni as a Triple System.” In other words, he was comparing the positions of the stars in the 61 Cygni binary system to demonstrate that they were changing over time in a cycle that showed the presence of an unseen companion. Here I’m dipping into the excellent Pipettepen site at the University of North Carolina, where Mackenna Wood has written up Strand’s work. And as Wood notes, Strand was limited to using glass photographic plates and a ruler to make measurements between the stars. Here’s the illustration Wood ran showing how tricky this would have been:
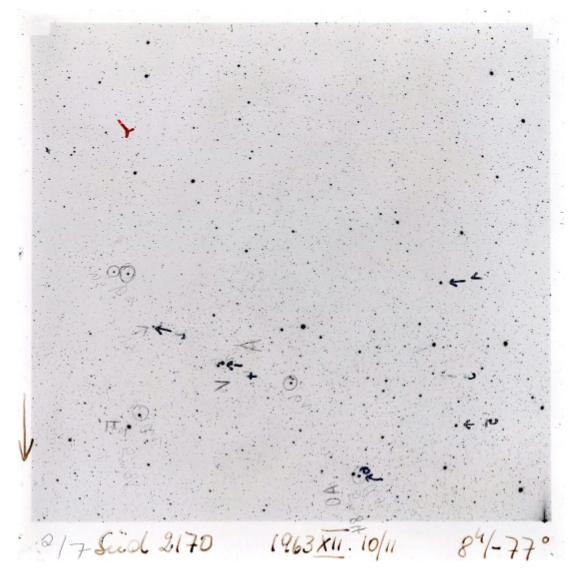
Image: An example of a photographic plate from one of the telescopes used in the 1943 61 Cygni study. The plate is a negative, showing stars as black dots, and empty space in white. Brighter stars appear as larger dots. Written at the bottom of the plate are notes indicating when the image was taken (Nov. 10, 1963), and what part of the sky it shows. Credit: Mackenna Wood.
Strand’s detection is no longer considered valid because more recent papers using more precise astrometry have found no evidence for a companion in this system. And that was a disappointment for readers of Arthur C. Clarke, who in his hugely exciting The Challenge of the Spaceship (1946) had made this statement in reference to Strand: “The first discovery of planets revolving around other suns, which was made in the United States in 1942, has changed all ideas of the plurality of worlds.”
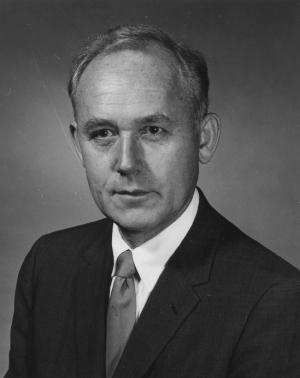
Can you imagine the thrill that would have run up the spine of a science fiction fan in the late 1940s when he or she read that? Someone steeped in Heinlein, Asimov and van Vogt, with copies of Astounding available every month on the newsstand and the great 1950s era of science fiction about to begin, now reading about an actual planet around another star? I have a lot of issues of Astounding from the late 1930s in my collection though few from the late ‘40s, but I plan to check on Strand’s work to see if it appeared in any fashion in John Campbell’s great magazine in the following decade. Surely there would have been a buzz at least in the letter columns.
Image: Kaj Aage Gunnar Strand (1907-2000) was director of the U.S. Naval Observatory from 1963 to 1977. He specialized in astrometry, especially work on double stars and stellar distances. Credit: Wikimedia Commons / US Navy.
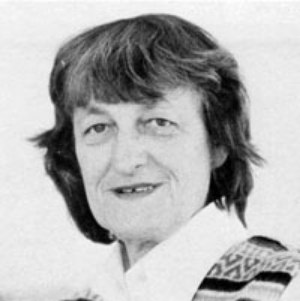
We’re not through with early exoplanet detection yet, though, and we’re staying at the same Sproul Observatory where Strand did the 61 Cygni work. It was in 1960 that another Sproul astronomer, Sarah Lippincott, published work arguing that Lalande 21185 (Gliese 411) had an unseen companion, a gas giant of ten Jupiter masses. A red dwarf at 8.3 light years out, this star is actually bright enough to be seen with even a small telescope. And in fact it does have two known planets and another candidate world, the innermost orbiting in a scant twelve days with a mass close to three times that of Earth, and the second on a 2800-day orbit and a mass fourteen times that of Earth. The candidate planet, if confirmed, would orbit between these two.
Image: Swarthmore College’s Sarah Lippincott, whose work on astrometry is highly regarded, although her exoplanet finds were compromised by faulty equipment. Credit: Swarthmore College.
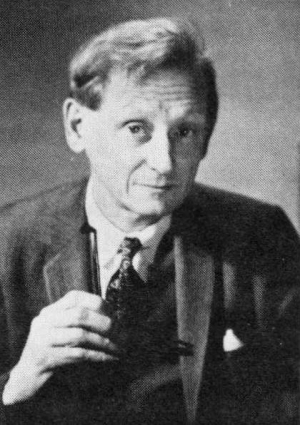
The work on Lalande 21185 in exoplanet terms goes back to Peter van de Kamp, who proposed a massive gas giant there in 1945. Lippincott was actually one of van de Kamp’s students, and the duo used astrometrical techniques to study photographic plates taken at Sproul. It turns out that Sproul photographic plates taken at the same time as those Lippincott used in her later paper on the star were later used by van de Kamp in his claim of a planetary system at Barnard’s Star. It was demonstrated later that the photographic plates deployed in both studies were flawed. Systematic errors in the calibration of the telescope were the culprit in the mistaken identifications.
Image: Astronomer Peter van de Kamp (1901-1995). Credit: Rochester Institute of Technology newsletter.
We always knew that exoplanet hunting would push us to the limits, and today’s bounty of thousands of new worlds should remind us of how the landscape looked 75 years ago when Otto Struve delved into detection techniques using the Doppler method. At that time, as far as he knew, there was only one detected exoplanet, and that was Strand’s detection, which as we saw turned out to be false. But Struve had the method down if hot Jupiters existed, and of course they do. He also reminded us of something else, that a large enough planet seen at the right angle to its star should throw a signal:
There would, of course, also be eclipses. Assuming that the mean density of the planet is five times that of the star (which may be optimistic for such a large planet) the projected eclipsed area is about 1/50th of that of the star, and the loss of light in stellar magnitudes is about 0.02. This, too, should be ascertainable by modern photoelectric methods, though the spectrographic test would probably be more accurate. The advantage of the photometric procedure would be its fainter limiting magnitude compared to that of the high-dispersion spectrographic technique.
There, of course, is the transit method which has proven so critical in fleshing out our catalogs of exoplanets. Both radial velocity and transit techniques would prove far more amenable to early exoplanet detection than astrometry of the sort that van de Kamp and Lippincott used, though astrometry definitely has its place in the modern pantheon of detection methods. Back in 1963, when van de Kamp announced the discovery of what he thought were planets at Barnard’s Star, he relied on almost half a century of telescope observations to build his case. No one could fault his effort, and what a shame it is that the astronomer died just months before the discovery of 51 Pegasi b. It would be fascinating to have his take on all that has happened since.