by Paul Gilster | Dec 31, 2013 | Communications and Navigation |
Best wishes for the New Year! I got a resigned chuckle — not a very mirthful one, to be sure — out of a recent email from Adam Crowl, who wrote: “Look at that date! Who imagined we’d still be stuck in LEO in 2014???” Indeed. It’s hard to imagine there really was a time when the ‘schedule’ set by 2001: A Space Odyssey seemed about right. Mars at some point in the 80’s, and Jupiter by the turn of the century, a steady progression outward that, of course, never happened. The interstellar community hopes eventually to reawaken those dreams.
Yesterday’s post on laser communications makes the point as well as any that incremental progress is being made, even if at an often frustrating pace. We need laser capabilities to take the burden off a highly overloaded Deep Space Network and drastically improve our data transfer and networking capabilities in space. The Lunar Laser Communication Demonstration (LLCD) equipment aboard the LADEE spacecraft transmitted data from lunar orbit to Earth at a 622 megabits per second (Mbps) rate in October, a download rate six times faster than any radio systems that had been flown to the Moon. It was an extremely encouraging outcome.
“These first results have far exceeded our expectation,” said Don Cornwell, LLCD manager. “Just imagine the ability to transmit huge amounts of data that would take days in a matter of minutes. We believe laser-based communications is the next paradigm shift in future space communications.”
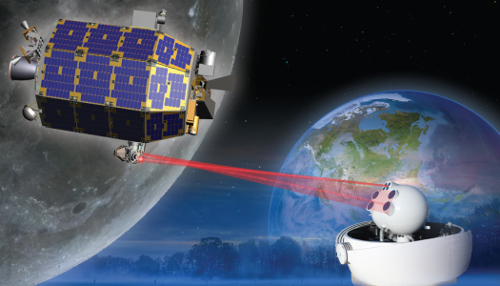
LLCD is actually an overall name for the ground- and space-based components of this laser experiment. What’s aboard the LADEE spacecraft is the Lunar Laser Space Terminal (LLST), which communicates with a Lunar Laser Ground Terminal (LLGT) located in White Sands, New Mexico, a joint project developed between MIT and NASA. There are also two secondary terminals, one at the European Space Agency’s La Teide Observatory (Tenerife), the other at JPL’s Table Mountain Facility in California, where previous laser experiments like GOLD — the Ground-to-Orbit Laser Communication Demonstration — have taken place.
The laser communication between LLCD and ground stations on Earth is the longest two-way laser communication ever demonstrated and a step in the direction of building the next generation of communications capability we’ll need as we explore the Solar System. Imagine data rates a hundred times faster than radio frequencies can provide operating at just half the power of radio and taking up far less space aboard the vehicle. Improvements in image resolution and true moving video would radically improve our view of planetary targets.
Laser methods are proving as workable as we had hoped. The LLCD demonstrated error-free communications during daylight, and could operate when the Moon was within three degrees of the Sun as seen from Earth. Communications were also possible when the Moon was less than four degrees from the horizon as seen from the ground station, and were successful even through thin layers of cloud, which NASA describes as ‘an unexpected bonus.’ A final plus: The demonstrated ability to hand off the laser connection from one ground station to another.
The scientific benefits of lasers are tangible but they’re matched by what could be a rise in public engagement with space if we can produce a networked infrastructure in which video plays a major role. Immersive gaming systems give way to the thought of rovers sending back high-resolution video from exotic places like Titan or Callisto — is this one way to rekindle the passion for exploration that sometimes seems to have died with Apollo? Robotic missions lack the immediacy and glamor of human crews but high bandwidth may help make up the slack, perhaps building momentum for later crewed missions to many of the same targets.
Up next is the Laser Communications Relay Demonstration (LCRD), which recently passed a preliminary design review. LRCD is to be a long-duration optical mission that will tweak optical relay services over a two-year period onboard a commercial satellite built by Space Systems Loral. We’re in the transitional period between demonstrators and reliable flight hardware. After its 2017 launch, LCRD will be positioned above the equator to carry that process forward.
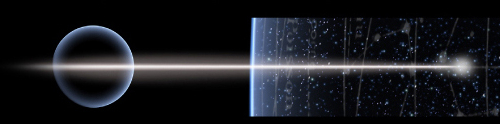
by Paul Gilster | Dec 30, 2013 | Communications and Navigation |
A recent email from Centauri Dreams regular Carl Keller reminded me about the laser communications tests conducted aboard a NASA satellite. The Lunar Atmosphere and Dust Environment Explorer satellite (LADEE) carried a laser package that demonstrated excellent download and upload rates and successful transmission of two simultaneous channels carrying high-definition video streams to and from the Moon. The fast transmission of large data files shows how useful laser methods will become.
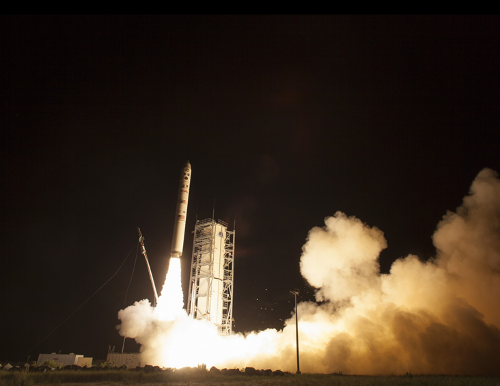
Image: NASA’s Lunar Atmosphere and Dust Environment Explorer (LADEE) observatory launches aboard the Minotaur V rocket from the Mid-Atlantic Regional Spaceport (MARS) at NASA’s Wallops Flight Facility, Friday, Sept. 6, 2013, in Virginia. Image Credit: NASA/Clara Cioffi.
All this is heartening because we need better communications as we begin to build a true infrastructure in the Solar System, while the demands of interstellar communication we’ll eventually need for probes of other stars are even more immense. The easy comparison is sitting right on our desktops in the form of the PCs we use everyday to communicate with the Net. Cable connections make website loading relatively painless, but most of us remember the frustration of early graphics coming in over painfully slow modem connections. Can lasers put the same kind of zip into communications from spacecraft at the edge of the Solar System?
Let’s hope so. I’m remembering the overloads that plague the Deep Space Network, extending decades back. In 1993, the Galileo spacecraft had a chance to take a close look at the asteroid 243 Ida, well worth viewing because the cratered rock was orbited by a ‘moon’. But the DSN also had to handle the load from controllers trying to revive the Mars Observer probe, so that important traffic that would have supported Galileo’s asteroid flyby was diverted. Galileo snapped a photo of Ida anyway, but the problem of overcrowded resources has only gotten worse.
In any case, when we’re talking truly long-distance communications, we have to reckon with the fact that our radio signals drop in intensity with the square of their distance, so a spacecraft ten times farther out than its twin sends a signal that’s fully one hundred times weaker. The numbers on actual missions staggered me when I first ran into them: The signal received from Voyager’s 23 watts was twenty billion times weaker than the power needed to operate a digital wristwatch when the Neptune encounter occurred back in 1989. Put that same signal around Alpha Centauri and it would arrive 81 million times weaker still, as I learned from James Lesh at JPL.
No wonder early starship designers leaned on massive dishes — consider the 40-meter second stage engine bell which, when burned out, the Daedalus craft would employ as a massive communications dish. And in order to process the signals from the starship, the British Interplanetary Society team assumed an Earth-based asset called Project Cyclops, one that would have been armed with a thousand 64-meter antennae. Like Robert Forward, the Daedalus designers as well as the SETI community was thinking big back in the 1970s.
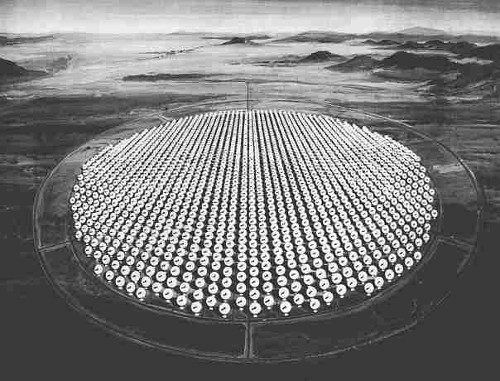
Image: What might have been. The gigantic Cyclops antenna array as envisioned in the 1970s. Credit: Columbus Optical SETI Observatory.
But Daedalus also was conceived as having laser capability that would be used while the craft was under power, and so was the US Navy student project called Project Longshot, which the class that came up with it equipped with six 250-kilowatt lasers, three for communications during the acceleration of the vehicle, and three for communications as Longshot arrived in the Alpha Centauri system. Lasers change the dynamic, but the point is we’re only now testing out the systems that will eventually make them commonplace in space communications.
Radio beams, after all, spread out at a diffraction rate determined by the wavelength of the signal divided by the diameter of the antenna. When we start pushing into higher and higher frequencies, the resulting signal becomes much more narrow. The advantages in reducing spectrum-crowding are supplemented by the laser signal’s ability to carry much more data, as the recent tests aboard LADEE demonstrate. Moreover, the optical telescopes needed aboard a spacecraft can be significantly smaller than the large radio dishes in use today.
Extend all those ideas into the far future and you wind up with an optical installation about the size of the Hubble Space Telescope capable of beaming useful data back to Earth from Alpha Centauri. That’s the 20-watt laser signal that would be beamed back to space-based telescopes in the Solar System, according to JPL’s Lesh in a well-known paper in JBIS. Remember that Voyager signal — it’s now puffed up to well over a thousand times the diameter of the Earth because of beam diffraction. The tight beam of the Centauri laser would get the message through. Of course, a way to propel a communications system as big as Hubble to another star has to be discovered first.
Can we get around all this with gravitational lensing and much smaller equipment? Conceivably, and I’ll have some interesting news about Claudio Maccone’s FOCAL mission to the Sun’s gravitational lens in the next few weeks. I also want to talk a bit more about the LADEE experiments. I’ve mentioned the Lesh paper in these pages before, but here’s the reference again: Lesh, C. J. Ruggier, and R. J. Cesarone, “Space Communications Technologies for Interstellar Missions,” Journal of the British Interplanetary Society 49 (1996): 7-14.
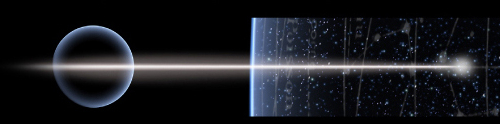
by Paul Gilster | Dec 27, 2013 | Exoplanetary Science |
‘Exomoons’ — moons around planets around other stars — are another of those new frontiers of modern astronomy. It’s astonishing to reflect that 51 Pegasi b, the first exoplanet orbiting a main-sequence star, was discovered as recently as 1995, a time when we could only suspect that planets might be common and were only then working out the best ways to find them. Now we have thousands of planet candidates, the search is on for true Earth analogues, and the idea that we might make as fine-grained a discovery as an exomoon is an exhilarating prospect.
So is the recent paper from David Bennett (University of Notre Dame) and colleagues the breakthrough we’ve been waiting for? The answer is no because we have no way of knowing whether this suggestive find is a true moon around a planet or perhaps two larger objects in gravitational synch at a much further distance. But either case is intriguing. Here are the possibilities for the 2011 event called MOA-2011-BLG-262, detected by gravitational microlensing:
- A gas giant of three to four times Jupiter’s mass at a distance of 1800 light years, the moon being, at about half the mass of Earth, absolutely gigantic in comparison to moons in our Solar System and orbiting its host world at a distance of 0.13 AU, quite a large separation. This is what Bennett and team call the ‘fast model,’ ; or
- A much more distant brown dwarf orbited by a Neptune-mass planet. This is the ‘slow model’ (see the paper for details on the use of these terms).
Now brown dwarfs with planets are interesting enough, so I would find either of these scenarios fascinating. But what makes the exomoon picture still more riveting is that these objects are evidently out there on their own, with no associated star. If we really are looking at an exomoon and a planet, then the question of how they came to be where they are emerges. New Scientist quotes exomoon hunter David Kipping (Harvard University) as saying “It almost begs the question as to whether we can really call these objects ‘moons’ or whether some other name is more apt.” What that other name might be is left to the imagination of the reader.
So unusual would a free-floating planet with a moon of its own be that the paper on this work leans preferentially to the brown dwarf solution. From the preprint:
…an apparently free-floating planet with a half Earth-mass moon would be a new class of system that was not previously known to exist. Such a new discovery would require strong evidence, so our favored model for this event is that it is a low-mass star or brown dwarf orbited by a planet of about Neptune’s mass.
Or as the abstract puts it: “The data are well fit by this exomoon model, but an alternate star+planet model fits the data almost as well.”
If that seems to take the sizzle out of this story, please reconsider. This interesting duo was identified by gravitational microlensing, in which the passage of an object in front of a more distant star bends the light from that star in ways that can be quantified. In the case of MOA-2011-BLG-262, the light of the distant star was magnified about seventy times beyond the norm. About an hour later the second, smaller increase in brightness occurred, suggesting the passage of two objects in front of the star. Because microlensing events don’t repeat, we may never be able to untangle the true story of MOA-2011-BLG-262. But the work of Bennett and colleagues points to future microlensing breakthroughs:
…it should be possible to definitely distinguish similar models for future events if they are observed with high cadence from multiple sites. Very high cadence observations on 1-2m class telescopes are able to measure the light curves precisely enough to distinguish similar models (Gould et al. 2006), such as the fast and slow models for MOA-2011-BLG-262. If these high cadence observations are taken from observatories separated by thousands of kilometers, then the terrestrial parallax effect can be used to measure the lens masses (Gould & Yee 2013).
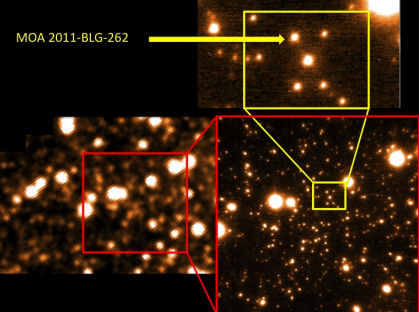
Image: The left panel shows a K-band (infrared) image from the VISTA 4m telescope from the VVV (Vista Variables in the Via Lactea) survey. The field observed by the Keck-2 telescope in K and a zoom of this field are shown to the right. The arrow indicates the microlensing source star, separated by 0.51 arcsec from its nearest neighbor. Credit: David Bennett.
‘Cadence’ as used in the passage above refers to how long it takes to re-observe the same target. High cadence can be combined with other techniques, and the paper notes that observatories separated by thousands of kilometers can take advantage of terrestrial parallax to increase and refine the microlensing detection rate. Ultimately, we’ll need new instruments:
…the development of large robotic networks of 1m class telescopes, such as the Las Cumbres Robotic Telescope Network (Brown et al. 2013), will substantially improve the rate of terrestrial microlensing parallax mass measurements. Thus, if systems resembling the planetary-mass host models for MOA-2011-BLG-262 are common, the combination of high cadence microlensing surveys, rapid realtime event detection by these surveys, and high cadence follow-up observations should enable the definitive discovery of rogue exoplanets with moons of nearly an Earth mass within a few years.
Recall the ground-breaking work of David Kipping and colleagues on using transit timing (TTV) and transit duration variations (TDV) to spot an exomoon signal in transit data, and the ongoing Hunt for Exomoons with Kepler project that Kipping runs. Add in this recent microlensing story and the possibility of future microlensing discoveries of the kind described above. It’s clear that we have exmoon detections in our future, and who knows what we may learn about the characteristics of rogue objects that, if MOA-2011-BLG-262 is a true indication, may not be traveling alone.
The paper is Bennett et al., “A Sub-Earth-Mass Moon Orbiting a Gas Giant Primary or a High Velocity Planetary System in the Galactic Bulge” (preprint).
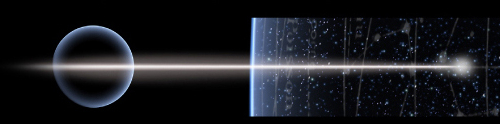
by Paul Gilster | Dec 24, 2013 | Exoplanetary Science |
Let me offer best wishes for the holidays to all Centauri Dreams readers, with thanks for the numerous comments and suggestions over the course of the past year. The schedule this week is abbreviated but I’ll have a new post up on Friday. I’m about to set out to gather the materials I need for a family dinner tonight, but I have time this morning to talk about interesting new work on figuring out the mass of an exoplanet. As you might guess, this is a key measurement, and a tough one to make. The work out of MIT offers an elegant solution.
I yield to no one in my admiration for the tough-minded Sara Seager (MIT), whose career in astrophysics is so movingly described in Lee Billings Five Billion Years of Solitude: The Search for Life Among the Stars
. A number of readers pointed out Seager’s most recent study, which develops this new technique for our exoplanet toolkit. Julien de Wit, a MIT grad student who is lead author on the paper just published in Science, describes his work with Seager as a way of determining the mass of an exoplanet using nothing more than the signature of its transit.
Now this is interesting stuff because learning something of a planet’s mass can help us make the call on whether it is a rocky world capable of supporting life. Radial velocity methods work well when larger planets are at play, or smaller worlds that orbit extremely close to their parent star. But radial velocity is trickier when we’re dealing with small planets orbiting further out. A planet like the Earth, for example, would be hard to analyze using radial velocity alone. We do, however, have the ability to study planetary atmospheres as planets transit their stars, and ingenious analysis may make it possible to extract from this a reading of a planet’s mass.
Thus de Wit’s description of what the duo have been up to:
“With this method, we realized the planetary mass — a key parameter that, if missing, could have prevented us from assessing the habitability of the first potentially habitable Earth-sized planet in the next decade — will actually be accessible, together with its atmospheric properties.”
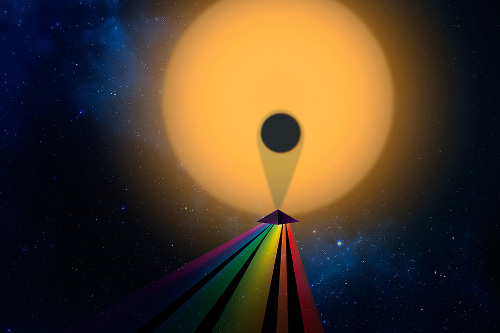
Image: Artistic rendering of a planet’s transmission spectrum. Credit: MIT.
How? Our old friend HD 189733b comes into play, a transiting ‘hot Jupiter’ some 63 light years away that has been a testbed for the technique called transmission spectroscopy, where scientists analyze the light that passes through the atmosphere to determine properties like temperature and the density of atmospheric molecules. Because we can study an atmosphere, we can study the effect of mass on that atmosphere. The method described in the de Wit/Seager paper works with a standard equation that describes the effect of three factors — temperature, gravitational force and atmospheric density — on the planet’s atmospheric pressure profile, which is a measure of how pressure changes throughout the atmosphere.
Believing that each of these factors could be derived independently from a transmission spectrum, de Wit studied the effects of each using the 18th Century mathematical constant called the Euler-Mascheroni constant. This MIT news release describes the constant as an ‘encryption key’ that helped decode the ways the properties of a planet’s atmosphere are embedded in its transmission spectrum. Turned on HD 189733b, the analysis yielded the same mass measurement that other scientists had obtained by radial velocity methods. Future space-based platforms should be able to turn these methods to much smaller worlds.
My particular interest in red dwarfs is piqued by this study, because radial velocity methods are not well suited for small planets orbiting faint stars. Extending the range of mass measurement through this new transit technique would make the mass of planets transiting red dwarfs that much more discoverable, offering another way of characterizing possibly habitable worlds. The technique is not, of course, limited to red dwarfs, and we can assume that Earth-sized planets orbiting stars not so different from the Sun will be studied using the same methods, once we have space-based instruments like the James Webb Space Telescope operational.
The paper is de Wit and Seager, “Constraining Exoplanet Mass from Transmission Spectroscopy,” Science 20 December 2013 (abstract).
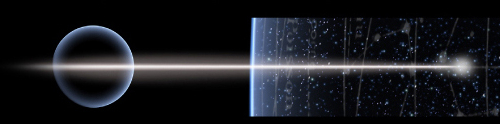
by Paul Gilster | Dec 23, 2013 | Missions |
I will admit to an obsession with small, dim stars, one that goes far enough to take in those not-quite stars called brown dwarfs, objects too small to ignite hydrogen fusion. The WISE mission showed us that, at least in our Sun’s neighborhood, brown dwarfs aren’t as common as we once thought, with perhaps one of them for every six main sequence stars. For their part, red dwarfs are the prime currency of the galaxy, accounting for 75 to 80 percent of all stars, so between the two we have a host of venues for planets and, possibly, life. But so much needs to be done before we’ll know if either brown or red dwarfs could really be candidates for astrobiology around them.
These thoughts are triggered by more news from WISE, now in its reactivated incarnation as NEOWISE (Near-Earth Object Wide-field Infrared Survey Explorer). The spacecraft came back to life in September after 31 months of hibernation and is now working to help us identify potentially hazardous near-Earth objects, a reminder that brown dwarfs were only a part of a much larger mission to characterize the infrared sky. But before I leave brown dwarfs today, let me run an image Greg Benford passed along, a Jon Lomberg conception of a brown dwarf.
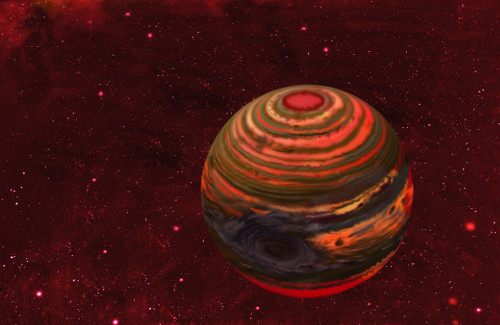
Image: Could a brown dwarf like this form planets that support life? Credit: Jon Lomberg.
If memory serves, I ran this image a long time ago in the course of another brown dwarf discussion, and Greg and I talked about it at the first 100 Year Starship Symposium in Orlando. The Lomberg artwork was on Greg’s mind when he finished his recent story “The Man Who Sold the Stars” (in Starship Century) with a scene set around a dim brown dwarf that was only discovered after a renewed search of old data, including that of the WISE mission. Greg describes a surreal landscape on a planet orbiting the brown dwarf called Redstar:
Stars shone in pale gray here against the inky black. The huge hull of Redstar hung as a burgundy disk cut off by the sea. Here and there across the long panorama of perpetual twilight, slanting rays of a deep Indian red showed floating plants, lapping on the waves in a somber sprawl. Everything glowed with infernal incandescence…. Down from the desolate slope to his left came an echoing cry, long and slow. In the thick air a thing like a huge orange gossamer butterfly fluttered on a thin wind. It swooped across a sky peppered with amber clouds and vanished with deliberate, long flaps of its enormous wings, vanishing behind a low eroded hill.
As we learn more about dim stars and much dimmer brown dwarfs, we have to answer key questions that impinge upon their chances for such life. About red dwarfs, we have to learn whether tidal lock offers stable meteorology anywhere on the surface (various papers have argued that it can), and whether the flare activity particularly in young stars would sterilize the planet or serve as a spur to evolutionary change. About brown dwarfs we have to learn whether these continually cooling objects produce rocky planets in close orbits that could stay warm long enough for life to thrive.
NEOWISE Surveys the Nearby Sky
We won’t have the answers to these questions any time soon. For this morning, then, back to NEOWISE. The image below shows a view from the spacecraft after its recent reactivation. If you’ll study the center of the image, you’ll see the track of an asteroid as captured in a series of exposures. This image shows an area in the constellation Pisces; the asteroid is (872) Holda. A red streak at the top of the image is the track of an Earth-orbiting satellite.
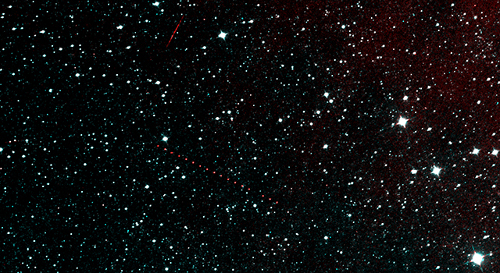
Image: NEOWISE originated as WISE (Wide-field Infrared Survey Explorer), which was put into hibernation in 2011 upon completing its goal of surveying the entire sky in infrared light. WISE cataloged three quarters of a billion objects, including asteroids, stars and galaxies. In August 2013, NASA decided to reinstate the spacecraft on a mission to find and characterize more asteroids. Credit: NASA/JPL.
From 2010 to early 2011, NEOWISE discovered more than 34,000 asteroids and characterized 158,000 throughout the Solar System. Amy Mainzer, principal investigator for NEOWISE at JPL, looks at the future of the mission:
“NEOWISE not only gives us a better understanding of the asteroids and comets we study directly, but it will help us refine our concepts and mission operation plans for future, space-based near-Earth object cataloging missions. The spacecraft is in excellent health, and the new images look just as good as they were before hibernation. Over the next weeks and months we will be gearing up our ground-based data processing and expect to get back into the asteroid hunting business, and acquire our first previously undiscovered space rock, in the next few months.”
NEOWISE uses a 40-centimeter telescope and infrared cameras in going about its work studying asteroid size, albedo, reflectivity and other properties. The original WISE mission captured more than 2.7 million images in various infrared wavelengths and catalogued well over 700 million objects. The primary goal of NEOWISE is to study asteroids and comets that approach within 45 million kilometers. It’s exciting and necessary work, but I do wish we had a second WISE working on brown dwarfs.
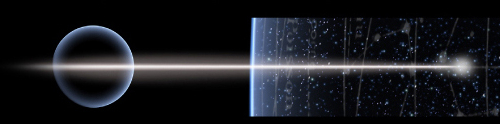