by Paul Gilster | Jul 5, 2024 | Sail Concepts |
Browsing through the correspondence that makes up Freeman Dyson’s wonderful Maker of Patterns: An Autobiography Through Letters (Liveright, 2018), I came across this missive, describing to his parents in 1958 why space exploration occupied his time at General Atomic, where he was working on Orion, the nuclear pulse concept that would explode atomic devices behind huge pusher plates to produce thrust. Dyson had no doubts about the value of humanity moving ever outward as it matured:
I am something of a fanatic on this subject. You might as well ask Columbus why he wasted his time discovering America when he could have been improving the methods of Spanish sheep farming. I think the parallel is a close one… We shall know what we go to Mars for only after we get there. The study of whatever forms of life exist on Mars is likely to lead to better understanding of life in general. This may well be of more benefit to humanity than irrigating ten Saharas. But that is only one of many reasons for going. The main purpose is a general enlargement of human horizons.
But there’s the thing, the driver for the entire Centauri Dreams effort these past twenty years. Just how do we go? And I mean that not only in terms of propulsion, the nuts and bolts of engines as well as the theory that drives them, but how we move outward carrying the cultural and scientific values of our species, Dyson thought deeply about these matters, as did Shklovskii and Sagan in their Intelligent Life in the Universe (Holden Day, 1966), who mused that an advanced civilization might view interstellar travel as a driver for creativity and philosophical growth. Perhaps culture remakes itself with each new exploration.
Greg Matloff’s investigations of these matters in numerous papers and key books like Deep Space Probes (Springer, 2005) have laid out the propulsion options from Project Orion to beamed lightsails, but his new paper, written with the artist C Bangs, draws on Dyson’s Astrochicken concept, first published in 1985, one of the few times I’ve seen it discussed in the literature (although I gave it a look in my 2005 Centauri Dreams book). Astrochicken was to be a one-kilogram probe to Uranus, a genetically engineered device powered by artificial intelligence.
As Dyson describes it in Infinite in All Directions (Harper & Row, 1988), “The plant component has to provide a basic life-support system using closed-cycle biochemistry with sunlight as the energy source. The animal component has to provide sensors and nerves and muscles with which it can observe and orient itself and navigate to its destination. The electronic component has to receive instructions from Earth and transmit back the results of its observations.”
Integrating all of this is artificial intelligence, creating a probe “…as agile as a hummingbird with a brain weighing no more than a gram.” Some years after Dyson introduced Astrochicken, Matloff discussed such a living probe, flitting from world to world, in Deep Space Probes, seeing elegance in the idea of wedding biology to technology. There he imagines a spacecraft like this fully fleshed out in the interstellar context, with a harvesting capability in the destination star system. He describes it thus:
…a living Astrochicken with miniaturized propulsion subsystems, autonomous computerized navigation via pulsar signals, and a laser communications link with Earth. The craft would be a bioengineered organism. After an interstellar crossing, such a living Astrochicken would establish orbit around a habitable planet. The ship (or being) could grow an incubator nursery using resources of the target solar system, and breed the first generation of human colonists using human eggs and sperm in cryogenic storage.
We have in this symbiosis of plant, animal and electronic components the possibility of leaving the Solar System and conceivably creating a von Neumann probe that combines engineering with the genetic manipulation of plant and animal DNA. Our probes need not be robotic, or at least entirely robotic, even if humans are not aboard.
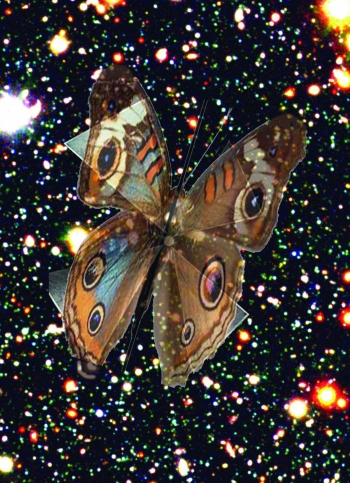
Out of this seed comes Space Butterfly, aptly named for its large, thin wings that can unfurl for a close stellar pass for acceleration and trajectory adjustments. In the new paper in JBIS, Matloff notes that this is a spacecraft with an affinity for starlight, using its wings as solar panels to power up its suite of scientific and communications equipment. Driven by its AI brain, it would be capable of mining resources found in exoplanetary systems, moving between stellar systems in passages of millennial length. Here I’m reminded of the oft-cited fact that only a small fraction of the Sun’s projected lifetime would serve for such space butterflies to explore and fill the galaxy, even moving at velocities that exceed Voyager by only a small amount. Quoting the paper:
Using hyper thin all-metallic sails and close perihelion passes, Space Butterfly could traverse the separation between neighboring stars in a few millennia. If it elects to come to rest temporarily within a planetary system, it can decelerate by electromagnetically reflecting encountered interstellar photons and pointing the fully unfurled sail towards the destination star…
If ET elects to construct Space Butterflies with very long lives, many spare AI ‘brains’ could be carried. This should produce no major problem since these units could have masses well under one gram. Spare parts could also be carried to replace non-biological portions of Space Butterfly.
Image: Flitting from star to star, the Space Butterfly concept is perhaps more like a Space Moth, with its affinity to starlight. Credit: C Bangs.
Plugging in the known characteristics of the interstellar object ‘Oumuamua, Matloff speculates on its characteristics if it were a Space Butterfly, exploring the kinematics – trajectory, velocity, acceleration – of this kind of probe. He uses a framework of mathematical tools that have evolved for the analysis of sail technologies, ranging from the lightness number of the sail (the ratio of radiation pressure force on the sail and solar gravitational force on the spacecraft), as well as radiation pressure from the Sun at perihelion, given what we know about sail materials and thickness.
If ‘Oumuamua were a sail, it would be a slow one, moving at an interstellar cruise velocity in the range of 26 kilometers per second, and thus requiring a solid 50,000 years for a crossing between the Sun and the Alpha Centauri stars, for example. A Space Butterfly should be able to do a good deal better than that, but we are still talking about crossings involving thousands of years. Civilizations interested in filling the galaxy with such probes clearly would have long lifetimes and attention spans.
Such timeframes challenge all our assumptions about a civilization’s survival and indeed the lifespan of the beings who operate within its strictures. Given that we know of no extraterrestrial civilizations, we can only speculate, and in my view the prospect of an advanced culture operating over millennial timeframes in waves of slow exploration is as likely as one patterned on the human model. A sentient probe carrying perhaps a post-biological consciousness not at the mercy of time’s dictates might find ranging the interstellar depths a matter of endless fascination. For such a being, the journey of discovery and contemplation may be of more value than any single arrival.
The paper is Matloff & Bangs, “Space Butterfly: Combining Artificial Intelligence and Genetic Engineering to Explore Multiple Stellar Systems,” Journal of the British Interplanetary Society Vol. 77 (2024), 16-19.
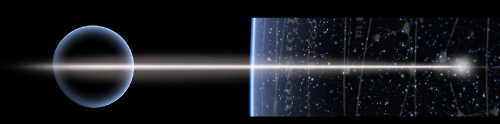
by Paul Gilster | Jul 3, 2024 | Sail Concepts |
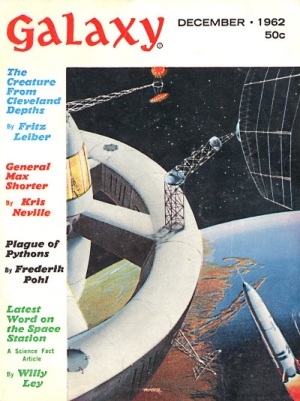
If you look at Galaxy’s December, 1962 issue, which I have in front of me from my collection of old SF magazines, you’ll find a name that appears only once in the annals of science fiction publishing: George Peterson Field. The article, “Pluto – Doorway to the Stars,” is actually by Robert Forward, who was at that time indulging in a time-honored practice, concealing an appearance in a science fiction venue so as not to raise any eyebrows with management at his day job at Hughes Aircraft Company.
Aeronautical engineer Carl Wiley had done the same thing with an article on solar sails in Astounding back in May of 1951, choosing the pseudonym Russell Saunders as cover for his work at Goodyear Aircraft Corporation (later Lockheed Martin). Both these articles were significant, as they introduced propulsion concepts for deep space to a popular audience outside the scientific journals. While solar sails had been discussed by the likes of J. D. Bernal and Konstantin Tsiolkovsky, the idea of sails in space now begins to filter into popular fiction available on any newsstand.
But despite being frequently referenced in the literature, Forward’s foray into Galaxy did not focus on sail technologies at all. Instead, it dwells on an entirely different concept, one that Forward called a ‘gravitational catapult.’ This is itself entertaining, so let’s talk about it for just a moment before pushing on to the actual first appearance of laser beaming to a sail, which Forward would produce in a different journal in the same year.
Forward is the master of gigantic engineering projects. Pluto had caught his attention because its eccentric orbit matched up with what Percival Lowell had predicted for a planet beyond Neptune, but its size was far too small to account for its supposed effects. Lowell had calculated that it would mass about six times Earth’s mass, a figure later corroborated by W. H. Pickering. But given Pluto’s actual size, Forward found that if it were the outer system perturber Lowell had predicted, it would have to have a density hundreds of times greater than water.
Remember, this was 1962, and in addition to being a physicist, Forward was a budding science fiction author playing with ideas in Galaxy, which had just passed from the editorship of H. L. Gold to that of Frederick Pohl, a man of lively imagination and serious SF chops himself. Why not play with the notion of Pluto as artifact? I think this was Forward’s first gigantic project. Thus:
…we can envision how such a gravitational catapult could be made. It would require a large, very dense body with a mass larger than the Earth, made of collapsed matter many times heavier than water. It would have to be whirling in space like a gigantic, fat smoke ring, constantly turning from inside out.
The forces it would exert on a nearby object, such as a spaceship, would tend to drag the ship around to one side, where it would be pulled right through the center of the ring under terrific acceleration and expelled from the other side. If the acceleration were of the order of 1000 g’s, then after a minute or so it would take to pass through, the velocity of the ship on the other side would be near that of light…
Forward imagined a network of such devices, each of them losing a bit of energy each time they accelerated a ship, but gaining it back when they decelerated an incoming ship. The Pluto reference is a playful speculation that what was then considered the ninth planet was actually one of these devices, which we would find waiting for us along with a note from the Galactic Federation welcoming us to use it. A sort of ‘coming out present’ to an emerging species. I can see the twinkle in his eye as he wrote this.
In any case, we have to change the history of beamed sails slightly to reflect the fact that the Galaxy appearance did not deal with sails, despite having a name similar to an article Forward published in the journal Missiles and Rockets in that same year. “Pluto – Gateway to the Stars” ran in the journal’s April, 1962 issue as part of a series by various authors on technologies for sending spacecraft to other planets. I had never seen the actual article until my friend Adam Crowl was kind enough to forward it the other day. Adam’s collection of interstellar memorabilia is formidable and has often fleshed out my set of early deep space papers.
Here what Forward latches onto is the most significant drawback to solar sailing, which relies on the momentum imparted by photons. This is the inverse square law, which tells us that the push we can get from solar photons decreases with the square of our distance from the Sun. Solar sails lose their punch somewhere around the orbit of Jupiter. What Carl Wiley first discussed in Astounding was the utility of sails for interplanetary exploration. Forward wanted to go a lot farther.
“Pluto – Gateway to the Stars” ran through the options for deep space available to the imagination in 1962, homing in on antimatter and concluding “it would be a solution if you were a science fiction writer,” which of course Forward would become. But he noted “there are a few engineering details.” The first of these would be the problem of antimatter production. The second is storage. With both of these remaining huge problems today, it’s intriguing that Forward actually spends more of this article on antimatter than on his innovative laser concept but runs aground on the problem of gamma radiation.
When the hydrogen-anti-hydrogen streams collide, the matter in the atoms will be transformed into pure energy, but the energy will be in the form of intense gamma radiation. We can stop the gamma rays in heavy lead shields and get our thrust this way, but the energy in the gamma rays will turn into heat energy in the shields and it will not be long before the whole rocket melts. What is needed is a gamma-ray reflector – and such a material does not exist. In fact, there are strong physical arguments against every finding any such material since the wavelengths of the gamma rays are smaller than the atomic structure of matter.
But sails beckon, and here the innovation is clear: Leave the propellant behind. Forward made the case that standard reaction methods could not obtain speeds anywhere near the speed of light because their mass ratios would be appallingly high, not to mention thermal problems with any design carrying its own propellant plant and energy sources. For interstellar purposes, he mused, the energy source and the reaction mass would have to be external to the vehicle, as indeed they are in a solar sail. But to go interstellar, we have to get around the inverse square law. Hence the laser:
There is a way to extend the idea of solar driven sails to the problem of interstellar travel at large distances from the sun. This is to use very large Lasers in orbits close to the sun. They would convert the random solar energy into intense, coherent, very narrow light beams that can apply radiation pressure at distances of light years.
However, since the Laser would have to be over 10 kilometers in diameter, this particular method does not look feasible for interstellar travel and other methods of supplying propulsive energy from fixed power plants must be found.
The editors of Missiles and Rockets seem to have raised their eyebrows at this early instance of Forward’s engineering, as witness the end of their caption to the image that accompanied the text.
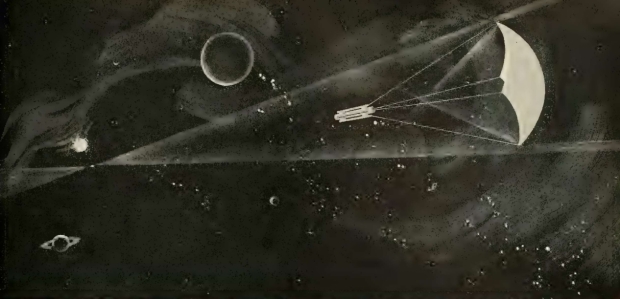
Image: This is the original image from the Missiles and Rockets article. Caption: Theoretical method for providing power for interstellar travel is use of a very large Laser in orbit close to sun. Laser would convert random solar energy into intense, very narrow light beams that would apply radiation pressure to solar sail carrying space cabin at distances of light years. Rearward beam from Laser would equalize light pressure. Author Forward observes, however, that the Laser would have to be over 10 kilometers in diameter. Therefore other means must be developed.
At this point in his career, Forward’s thinking leaned toward fusion to solve the interstellar conundrum, but as events would prove, he would increasingly return to beamed sails of kilometer scale, and power station and lensing structures that are far beyond our capabilities today. But if we ever do create smart assemblers at the nanotech level, the idea of megastructures of our own devising may not seem quite so preposterous. And this 1962 introduction to beamed sails is to my knowledge their first appearance in the literature. Today the concept continues to inspire research on beaming technologies at various wavelengths and using cutting edge sail materials.
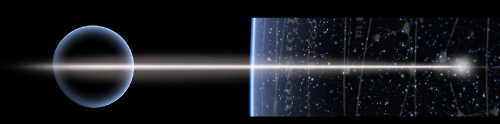
by Paul Gilster | Jun 7, 2024 | Sail Concepts |
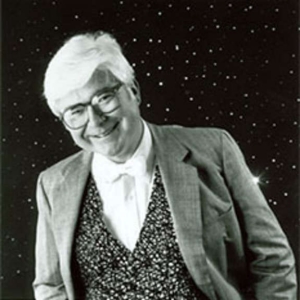
Although solar sails were making their way into the aerospace journals in the late 1950s, Robert Forward was the first scientist to consider using laser beams rather than sunlight to drive a space sail. That concept, which György Marx picked up on in his 1966 paper, opened the door to interstellar mission concepts. Late in life in an unpublished memoir, Forward recalled reading about Theodore Maiman’s work on lasers at Hughes Research Laboratories, and realizing that this was a way to create a starship. His 1962 article (citation below) laid out the idea for the journal Missiles and Rockets and was later reprinted in Science Digest. Marx surely knew the Forward article and his subsequent paper in Nature probed how to achieve this goal.
Image: One of the great figures of interstellar studies, Robert Forward among many other things introduced and explored the principles of beamed propulsion. Credit: UAH Library Robert L. Forward Collection.
Marx was at that time a professor of theoretical physics at Roland Eötvös University in Budapest. He was plugged into the difficulties of interstellar flight through Les Shepherd’s work in Britain, and he cites the latter’s Realities of Space Travel (1957) in the paper as one of many sources highlighting the depth of the problem. His paper “Interstellar Vehicle Propelled by Terrestrial Laser Beam” is a mere two pages built largely around equations, reporting on the “commonly accepted view that, apart from the technical difficulties involved, the laws of conservation of energy and momentum forbid the visiting of other planetary systems in the human lifespan.”
Marx had already explored energy issues for interstellar flight in a 1960 paper for Astronautica Acta (as it was then known) and a second for the same journal in 1963 (citations below). But the idea of laser beaming offered the physicist a glimmer of hope. In the 1966 paper, he cites the advantages of beamed sailing vs conventional rocket propulsion. The paper argues that “a vehicle can be accelerated almost to the speed of light if an emitter on the Earth can accurately project light onto its mirror.”
The ideal focusing mechanism would be the laser, and it is here that he runs into trouble. For Marx worried about the size of the transmitter aperture, which determines the size and initial beam diameter that will emerge. Remember we’re in the Robert Forward era of maxed out engineering, when the idea was simply to establish what was possible even if it required building capabilities far beyond those of the present day. So here’s what Marx comes up with, the best concept he thought feasible:
…the technical conditions are extremely challenging. A range of operation of 0.1 light year would require a coherently radiating surface of the order of 1 km2 which emits hard X-rays, and the vehicle would need an X-ray mirror with an effective cross-sectional area of several km2.
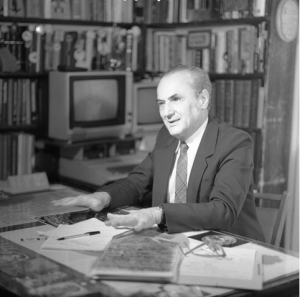
Marx talks about the absorbed energy of his sail ‘mirror’ radiating out isotropically into space, and here we run into serious problems. I took my questions to Jim Benford, CEO of Microwave Sciences and author of High Power Microwaves, a standard text which is about to go into its fourth edition at CRC Press. Jim is also a regular contributor to Centauri Dreams. And he was quick to point out that X-rays reflect from conducting surfaces in ways that defeat Marx’s purpose.
Image: György Marx in his office. Credit: REAL-I, the Image File Collection of the Academic Library.
As Jim told me, incoming X-rays reflect only at very low grazing angles. The efficiency of energy transfer is at stake here. Here’s a bit more of what he said, using one of the most important formulae in all of modern physics:
X-ray photons have far more energy than visible light or microwaves. Remember the relation E=hν, where E is the energy of the photon, h is Planck’s constant, ν the frequency. X-ray photons have energies about a thousand times that of visible light, a million times that of microwaves. If they come in normal to the surface [i.e., striking the sail head on], they ionize atoms, damaging the lattice of the material.
X-ray telescopes, as a matter of fact, work through a series of grazing incidence reflectors. In other words, we can’t direct Marx’s fantastic X-ray beam toward our sail without seriously damaging it, not unless we are willing to bring the beam to it at such a low angle that the intrinsic power of the beam is largely lost. Benford again:
There’s no way to accelerate a sail with X-rays. The cross-section of the sail must be at a slight angle to the beam, not perpendicular to it, for the X-rays to reflect. That’s hugely inefficient. Grazing incidence means that only the slight transverse component of the photon velocity vector is reversed, leaving the far larger axial component almost unchanged. Little energy is transferred to the inclined sail, and that drives it sideways to the beam, not antiparallel to it, as reflected photons do when they incident normally. So the sail is accelerated very little in the direction of the X-ray beam.
This is the coup de grâce for the X-ray sail. It’s interesting to see what Robert Forward thought of Marx’s idea. Here he is, writing in a 1984 paper called “Roundtrip Interstellar Travel Using Laser-Pushed Lightsails,” which is one of the classics of the field:
The concept of laser-pushed interstellar lightsails was reinvented by Marx in 1966. Since Marx was unwilling to consider a laser aperture greater than 1 km2, he was forced to assume the use of hard x-rays in order to obtain the operational ranges needed for interstellar flight. The impossibility of constructing both an x-ray laser and a lightweight sail to reflect those x-rays led to Marx’s highly pessimistic conclusion about the feasibility of the concept. If Marx had been willing to consider a larger transmitter aperture, then his laser frequencies and sail requirements would have been much easier.
J. L. Redding, then at Bishop’s University in Quebec, saw Marx’s paper and responded to it in the same year, offering corrections to Marx’s equations without challenging the X-ray concept. His telling remark that “…one does not need to consider the difficulties of arranging suitable deceleration and landing facilities” refers to what he saw as the overwhelming problems in making a beamed energy propulsion system work at all. Marx had commented on the deceleration problem and Forward would go on to offer a potential solution in his 1984 paper, one so baroque that it deserves a future post of its own.
I should also mention a little referenced paper by W. E. Moeckel, “Propulsion by Impinging Laser Beams,” which ran in the Journal of Spacecraft and Rockets in 1972. Moeckel (working at what was then NASA’s Lewis Research Center in Cleveland) analyzed laser beaming to 100 ton relativistic flyby probes, each of which would require 1012 watts of X-ray energy. Making specific reference to Marx, Moeckel found X-ray beaming promising but did not know if it was feasible. His conclusion would have warmed the hearts of science fiction writers of the time:
…some future generations of mankind, with a somewhat different ordering of priorities than ours and much more available power, could conceivably explore other stars and other solar systems with highly sophisticated unmanned spacecraft capable of relaying information in elapsed times of the order of decades.
If only it worked! Fortunately, we’re not restricted to X-rays when it comes to beamed propulsion.
References
The early Forward paper is “Pluto-Gateway to the Stars,” Missiles and Rockets 10, 26 ff. (2 April 1962); reprinted in Science Digest 52, 70-75 (August 1962). Forward’s “Roundtrip Interstellar Travel Using Laser-Pushed Lightsails” appeared in the Journal of Spacecraft and Rockets 21 (1984), pp. 187-195 (abstract).
György Marx’s paper on X-ray beaming is “Interstellar Vehicle Propelled by Terrestrial Laser Beam,” which ran in Nature on July 2, 1966 (abstract). His two other interstellar papers are “The mechanical efficiency of interstellar vehicles,” Astronautica Acta 9 (1963) 131–139, and “Über Energieprobleme der Interstellaren Raumfahrt,” Astronautica Acta 6 (1960) 366–372.
The Redding paper in response to Marx has the same title, “Interstellar Vehicle Propelled by Terrestrial Laser Beam,” Nature February 11, 1967 (abstract). W. E. Moeckel’s paper “Propulsion by Impinging Laser Beams” appeared in the Journal of Spacecraft and Rockets Vol. 9 (1972), 942-944 (abstract).
My thanks to Jim Benford, Greg Matloff and Al Jackson for invaluable references and commentary.
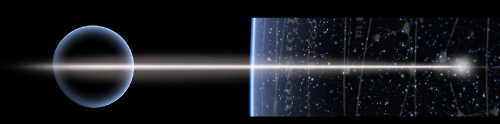
by Paul Gilster | May 31, 2024 | Sail Concepts |
In search of ever-higher velocities leaving the Solar System, we need to keep in mind the options offered by the solar wind. This stream of charged plasma particles flowing outward from the Sun carves out the protective bubble of the heliosphere, and in doing so can generate ‘winds’ of more than 500 kilometers per second. Not bad if we’re thinking in terms of harnessing the effect, perhaps by a magnetic sail that can create the field needed to interact with the wind, or an electric sail whose myriad tethers, held taut by rotation, create an electric field that repels protons and produces thrust.
But like the winds that drove the great age of sail on Earth, the solar version is treacherous, as likely to becalm the ship as to cause its sails to billow. It’s a gusty, turbulent medium, one where those velocities of 500 kilometers and more per second can as likely fall well below that figure. Exactly how it produces squalls in the form of coronal mass ejections or calmer flows is a topic under active study, which is where missions like Solar Orbiter come into play. Studying the solar surface to pin down the origin of the wind and the mechanism that drives it is at the heart of the mission.
Launched in 2020, Solar Orbiter carries a panoply of instruments, ten in all, for the analysis, including an Electron Analyzer System (EAS), a Proton-Alpha Sensor (PAS) for measuring the speed of the wind, and a Heavy Ion Sensor (HIS) designed to measure the heavy ion flow. Critical to the analysis of this paper is the Spectral Imaging of the Coronal Environment (SPICE) instrument, as we’ll see below. Steph Yardley (Northumbria University) is lead author of the paper on this work, which has just appeared on Nature Astronomy:
“The variability of solar wind streams measured in situ at a spacecraft close to the Sun provide us with a lot of information on their sources, and although past studies have traced the origins of the solar wind, this was done much closer to Earth, by which time this variability is lost. Because Solar Orbiter travels so close to the Sun, we can capture the complex nature of the solar wind to get a much clearer picture of its origins and how this complexity is driven by the changes in different source regions.”
What the work is analyzing is a theory that the process of magnetic field lines breaking and reconnecting is critical to producing the slower solar wind. Different areas of the Sun’s corona are implicated in the origin of both the fast and slow winds, with the ‘open corona’ being those regions where magnetic field lines extend from the Sun into space, tethered to it at one end only and creating the pathway for solar material to flow out in the form of the fast solar wind. Closed coronal regions, on the other hand, are those where the magnetic field lines connect to the surface at both ends, forming loops.
As you would imagine, the process is wildly turbulent and marked by the frequent breakage of these closed magnetic loops and their subsequent reconnection. The researchers have probed the theory that the slow solar wind originates in the closed corona during these periods of breakage and reconnection by studying the composition of solar wind streams, for the heavy ions emitted vary depending on their origins in either the closed or open corona. Solar Orbiter’s Heavy Ion Sensor (HIS) is able to take the needed measurements to relate the effects of this activity on the surrounding plasma.
The image below is from the Solar Dynamics Observatory spacecraft rather than Solar Orbiter, reminding us of the different views we are gaining by our various missions to our star. The comparison of key datasets tells the story.
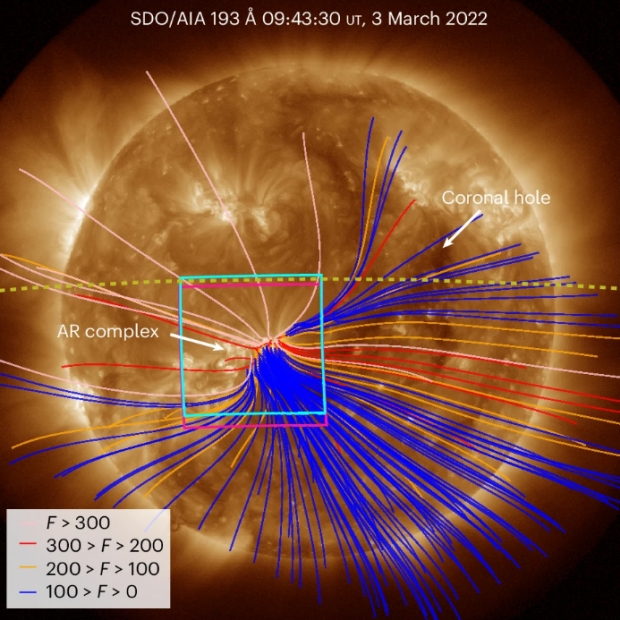
Image: This is part of Figure 1 from the paper. The caption reads: SDO/AIA [Solar Dynamics Observatory data using its Atmospheric Imaging Assembly] 193 Å image showing the source region from the perspective of an Earth observer. Open magnetic field lines that are constructed from the coronal potential field model are overplotted, coloured by their associated expansion factor F. The large equatorial CH [Coronal Hole] and AR [Active Region] complex are labeled in white. The FOVs [fields of view] of SO EUI/HRI and PHI/HRT [references to instruments aboard Solar Orbiter] are shown in cyan and pink, respectively. The back-projected trajectory of SO [Solar Orbiter] from 1 March 2022 until 9 March 2022 is shown by the olive dotted line (from right to left).
So because we have Solar Orbiter, we can now combine observations of the Sun from various sources including other space missions, like the Solar Dynamics Observatory, with the measurements of the solar wind actually flowing past the spacecraft. Susan Lepri (University of Michigan) is deputy principal investigator on the HIS system:
“Each region of the Sun can have a unique combination of heavy ions, which determines the chemical composition of a stream of solar wind. Because the chemical composition of the solar wind remains constant as it travels out into the solar system, we can use these ions as a fingerprint to determine the origin of a specific stream of the solar wind in the lower part of the Sun’s atmosphere.”
The results have been productive. The analysis gives us a precise breakdown of just what Solar Orbiter has encountered during the period studied. This is a thorny quotation but it includes a key finding. From the paper:
Combining the SO [Solar Orbiter] trajectory, coronal field model, magnetic connectivity tool, the SPICE composition analysis of the AR [Active Region] complex, and the in situ plasma and magnetic field parameters, we suggest that SO was immersed in three fast wind streams… originating from the three linked sections of the large equatorial CH [Coronal Hole]… These were followed by two slower streams associated with the negative polarities of the AR complex… The decrease of the solar wind speed can be explained by the expansion of the open magnetic field associated with the CH-AR complex, as the connectivity of SO transitioned across these regions. Credit: Yardley et al.
The findings described here are significant. We learn from this work just how complex the solar wind flow is, in this case involving three fast streams and two slower ones, all involving changes in magnetic field connectivity. Matching the composition of the solar wind streams to different areas on the corona gives us new insights into the turbulent mix found where the open and closed corona meet. The slow solar wind’s ‘breakout’ from closed magnetic field lines is demonstrated. The phenomenon of magnetic reconnection proves critical to the wind’s variability.
Demonstrating these linkages means that we can now use the findings to probe further into the origins of the solar wind. But this is a variability that is in no way predictable, making the prospect of riding the solar wind via electric or magnetic sail a daunting one. We’ll continue to learn more, though, as we bring in data from missions like the Parker Solar Probe. It will be fascinating to see one day how we use the solar wind to test out possible spacecraft designs in search of a faster route to the outer Solar System.
Addendum: In an earlier draft, I mistakenly criticized the authors for not initially clarifying some of the acronyms in this paper. I’ve removed that comment because a later reading showed I was mistaken about the two examples I cited.
The paper is Yardley et al., “Multi-source connectivity as the driver of solar wind variability in the heliosphere,” Nature Astronomy 28 May 2024 (full text).
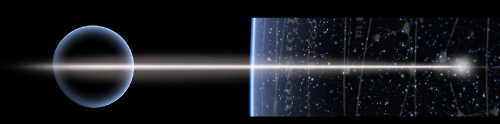
by Paul Gilster | Feb 16, 2024 | Sail Concepts |
How long before we can send humans to another star system? Ask people active in the interstellar community and you’ll get answers ranging from ‘at least a century’ to ‘never.’ I’m inclined toward a view nudging into the ‘never’ camp but not quite getting there. In other words, I think the advantages of highly intelligent instrumented payloads will always be apparent for missions of this duration, but I know human nature well enough to believe that somehow, sometime, a few hardy adventurers will find a way to make the journey. I do doubt that it will ever become commonplace.
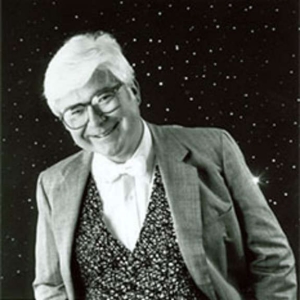
You may well disagree, and I hope you’re right, as the scenarios open to humans with a galaxy stuffed with planets to experience are stunning. Having come into the field steeped in the papers and books of Robert Forward, I’ve always been partial to sail technologies and love the brazen, crazy extrapolation of Forward’s “Flight of the Dragonfly,” which appeared in Analog in 1982 and which would later be turned into the novel Rocheworld (Baen, 1990). This is the novel where Forward not only finds a bizarre way to keep a human crew sane through a multi-decade journey but also posits a segmented lightsail to get the crew home.
Image: The extraordinary Robert Forward, whose first edition of Flight of the Dragonfly was expanded a bit from the magazine serial and offered in book form in 1984. The book would later be revised and expanded further into the 1990 Baen title Rocheworld. The publishing history of this volume is almost as complex as the methods Forward used to get his crew back from Barnard’s Star!
Forward was a treasure. Like Freeman Dyson, his imagination was boundless. Whether we would ever choose to build the vast Fresnel lens he posited in the outer Solar System as a way of collimating a laser beam from near-Sol orbit, and whether we could ever use that beam to reflect off detached segments of the sail upon arrival to slow it down are matters that challenge all boundaries of engineering. I can hear Forward chuckling. Here’s the basic idea, as drawn from his original paper on the concept.
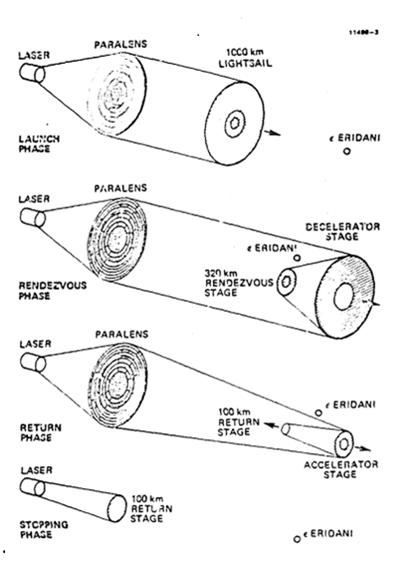
Image: Forward’s separable sail concept used for deceleration, from his paper “Roundtrip Interstellar Travel Using Laser-Pushed Lightsails,” Journal of Spacecraft and Rockets 21 (1984), pp. 187-195. The ‘paralens’ in the image is a huge Fresnel lens made of concentric rings of lightweight, transparent material, with free space between the rings and spars to hold the vast structure together, all of this located between the orbits of Saturn and Uranus. Study the diagram and you’ll see that the sail has three ring segments, each of them separating to provide a separate source of braking or acceleration for the arrival, respectively, and departure of the crew. Imagine the laser targeting this would require. Credit: Robert Forward.
I tend to think that Les Johnson is right about sails as they fit into the interstellar picture. In a recent interview with a publication called The National, Johnson (NASA MSFC) made the case that we might well reach another star with a sail driven by a laser. Breakthrough Starshot, indeed, continues to study exactly that concept, using a robotic payload miniaturized for the journey and sent in swarms of relatively small sails driven by an Earth-based laser. But when it comes to human missions to even nearby stars, Johnson is more circumspect. Let me quote him on this from the article:
“As for humans, that’s a lot more complicated because it takes a lot of mass to keep a group of humans alive for a decade-to centuries-long space journey and that means a massive ship. For a human crewed ship, we will need fusion propulsion at a minimum and antimatter as the ideal. While we know these are physically possible, the technology level needed for interstellar travel seems very far away – perhaps 100 to 200 years in the future.”
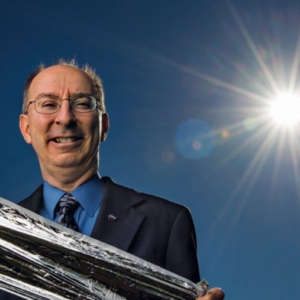
Johnson’s background in sail technologies for both near and deep space at Marshall Space Flight Center is extensive. Indeed, there was a time when his business card described him as ‘Manager of Interstellar Propulsion Technology Research’ (he once told me it was “the coolest business card ever”). He has also authored (with Gregory Matloff and Giovanni Vulpetti), books like Solar Sails: A Novel Approach to Interstellar Travel (Springer, 2014) and A Traveler’s Guide to the Stars (Princeton University Press, 2022), as well as editing the recent Interstellar Travel: Purpose and Motivations (Elsevier, 2023). In addition to that, his science fiction novels have explored numerous deep space scenarios.
Image: NASA’s Les Johnson, a prolific author and specialist in sail technologies. Credit: NASA.
So there’s a much more optimistic take on the human interstellar guideline than the one I gave in my first paragraph, and of course I hope it’s on target. We’re probably not going to be going to what is sometimes called an “Earth 2.0,” in Johnson’s view, because he doubts there are any such reasonably close to us. That’s something we’ll be learning a great deal more about as future space instrumentation comes online, but we can bear in mind that the explorers who tackled the Pacific in the great era of sail didn’t set out thinking they were going to find another Europe, either. The point is to explore and to learn what you can, with all the unexpected benefits that brings.
Johnson’s early interest in sails, by the way, was fired not so much by Forward’s Rocheworld as by Larry Niven and Jerry Pournelle’s novel The Mote in God’s Eye (Simon & Schuster, 1974), where an incoming laser sail from another civilization is detected. The realization that unusual astronomical observations point to a technology, and a laser-beaming one at that, is an exciting part of the book. Here the authors’ human starship crew describes the detection of a strange light emanating from a smaller star (the Mote) in front of a much larger supergiant (the Eye):
“…I checked with Commander Sinclair. He says his grandfather told him the Mote was once brighter than Murcheson’s Eye, and bright green. And the way Gavin’s describing that holo – well, sir, stars don’t radiate all one color. So -”
“All the more reason to think the holo was retouched. But it is funny, with that intruder coming straight out of the Mote…”
“Light,” Potter said firmly.
“Light sail!” Rod shouted in sudden realization…”
For more on all this, see my Our View of a Decelerating Magsail in these pages. It’s not surprising that Niven and Pournelle ran their lightsail concept past Robert Forward at a time when the idea was just gaining traction. We all have career-changing literary experiences. I can remember how a childhood reading of Poul Anderson’s The Enemy Stars (J.B. Lippincott, 1959) utterly fired my imagination toward the idea of leaving the Solar System entirely. It was a finalist for the Hugo Award that year following serialization in Astounding, though I didn’t encounter it until later.
Johnson’s work at Marshall Space Flight Center takes in the deployment of a large solar sail quadrant for the Solar Cruiser mission that was first unfurled in 2022 to demonstrate TRL 5 capability, and has just been deployed at contractor Redwire Corp.’s facility in Longmont, Colorado to demonstrate TRL 6. In NASA’s terms, that means going from “Component or breadboard validation in relevant environment” (TRL 5) to “System or subsystem model or prototype demonstration in a relevant environment (ground or space).” In other words, this is progress. At TRL 6, a system is considered “a fully functional prototype or representational model.” Says Johnson in a recent email:
“25 years ago, when I first met Dr. Forward, he inspired me to plan a development program for solar sails that would eventually lead us to the stars. With Bob’s help, I laid out a milestone driven roadmap that began with the space flight of a 10 m² solar sail, which we did in 2010 with NanoSail-D.
“Next on the plan was the development of something an order of magnitude larger. This was achieved with the development and launch of the 86 m² Near Earth Asteroid Scout solar sail in 2022 and the soon to be launched ACS-3 sail. The Solar Cruiser sail is an order of magnitude larger still at 1653 Square meters. The next step is 10,000!”
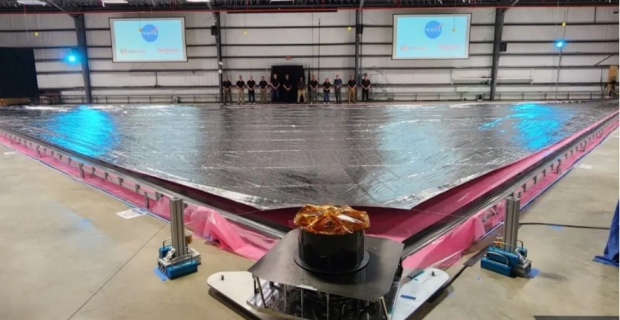
Image: NASA and industry partners used two 100-foot lightweight composite booms to unfurl the 4,300-square-foot sail quadrant for the first time Oct. 13, 2022, at Marshall Space Flight Center, making it the largest solar sail quadrant ever deployed at the time. On Jan. 30, 2024, NASA cleared a key technology milestone, demonstrating TRL6 capability at Redwire’s new facility in Longmont, Colorado, with the successful deployment of one of four identical solar sail quadrants. Credit: NASA (although I’ve edited the caption slightly to reflect the TRL level reached).
Solar sails are becoming viable choices for space missions, and the Breakthrough Starshot investigations remind us that sails driven not just by sunlight but by lasers are within the bounds of physics. A key question that will be informed by our experience with solar sails is how laser-driven techniques scale. Theoretically, they seem to scale quite well. Are the huge structures Forward once wrote about remotely feasible (perhaps via nanotech construction methods), or is Johnson right that fusion and one day antimatter may be necessary for craft large enough (and fast enough) to carry human crews?
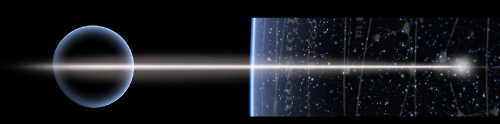
by Paul Gilster | Dec 22, 2023 | Sail Concepts |
Reaching ‘Oumuamua through some kind of statite technology, an idea we’ve been kicking around recently, brings up the interesting work of Richard Linares at MIT, who has been working on a “dynamic orbital slingshot” for rendezvous with future objects from the interstellar depths (ISOs). Linares received a Phase I grant from the NASA Innovative Advanced Concepts (NIAC) Program to pursue the idea of a network of statites on sentry duty, any one of which could release the stored energy of the sail to enter a trajectory that would take it to a flyby of an object entering our system on a hyperbolic orbit.
The concept is simplicity itself, once you realize that a statite balances the pressure of solar photons against the Sun’s gravitational pull, and essentially hovers in place. As I mentioned when covering Greg Matloff and Les Johnson’s paper on using statites to achieve fast rectilinear trajectories to reach interstellar interlopers, Robert Forward was the one who came up with the idea and practical uses for it. He could envision, for example, communications satellites in polar position to cover high latitudes on Earth.
Here’s what Forward said about the statite concept in his wonderful essay collection Indistinguishable from Magic (1995):
…I have the patent on it — U.S. Patent 5,183,225 “Statite: Spacecraft That Utilizes Light Pressure and Method of Use”… The unique concept described in the patent is to attach a television broadcast or weather surveillance spacecraft to a large highly reflective lightsail, and place the spacecraft over the polar regions of the Earth with the sail tilted so the light pressure from the sunlight reflecting off the lightsail is exactly equal and opposite to the gravity pull of the Earth.
You can see why we need a new term here. If you deploy a sail in the configuration Forward describes, it essentially sits over the polar region while the Earth rotates below it. In other words, technically it is not a satellite. ‘Statite’ is a Forward coinage to describe such a hovering object in space. He wrote of a statite he dubbed the ‘Hovering Hawke’ in one of his short stories. It would be placed too far from the surface to be effective as a communications satellite, but could offer direct broadcasting to places on Earth that are without that capability. Weather surveillance is another use.
Polesitters become interesting when we consider the nature of a geostationary orbit. Put a satellite directly over the equator at 35,786 kilometers altitude and it will appear stationary over the Earth, a useful trait for communications. But the satellite must be positioned directly above the equator, matching Earth’s rotation, to maintain its position relative to the surface.
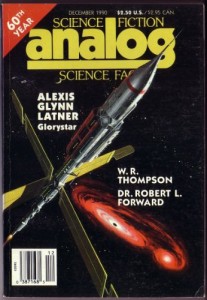
If we put our satellite at an angle relative to the equator, its apparent motion on Earth will be a figure eight, in what is called an inclined geosynchronous (not geostationary) orbit. That’s useful for areas not covered by geostationary satellites but not good enough for continuous coverage of a specific area, especially the more latitudinally challenged regions like the poles, and that’s why the polesitter is attractive. It can give us continuous coverage even when the region it sits above is far from the equator.
Image: Analog‘s December, 1990 issue contained an article by Robert Forward describing the ‘polesitter’ concept, one of many innovative ideas the scientist introduced to a broad audience. Credit: Condé Nast.
There’s always a catch, and here’s the catch with polesitters, as Forward explained it in his article. When the summer months arrive and the polar regions are in sunlight, keeping the statite precisely balanced (to maintain the hover) becomes quite tricky. He saw that such seasonal instability demanded that a statite be relatively far from Earth, and calculated that it cannot get any closer than 250 Earth radii to the surface.
But Linares and team are not thinking about statites supplying services to Earth. The NIAC work explores using statites to set up an early warning system for interstellar objects, one that will allow fast intercepts before these interlopers blow through our system and return to interstellar space. Consider what happens when we ‘turn off’ the statite capability on our satellite (as from rotating the sail to an edge-on position, for example, or simply releasing a CubeSat). At this point, the released object has no forces impinging upon it but gravity. Let me quote Linares from a white paper on the subject:
…a statite at 1 AU has a free-fall trajectory of about 64 days. This fast response time to a potential ISO can be thought of as a slingshot effect, since the solar sail is used to “store energy” that is released when desired. Additionally, to achieve a flyby some Delta-V is required to adjust from the free-fall path to a flyby trajectory. The proposed mission for the statite concept is to utilize a constellation of such devices to achieve wider coverage over a spherical region of 1 AU for potential ISO missions. Additionally, the orbital plane can be adjusted with relatively low Delta-V.
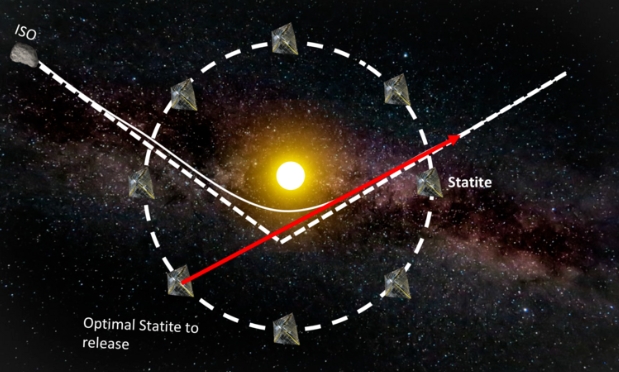
Image; A constellation of statites as envisioned by Richard Linares for intercepting a future interstellar interloper. Credit: MIT/Richard Linares.
The levitating sail has an inertial velocity of zero, and when released from ‘hover,’ it enters a Keplerian orbit. So as Linares points out, we can turn any one of the statites in our constellation of statites into a ‘sundiver,’ hurtling toward the Sun before its trajectory is adjusted by use of the sail (or perhaps other propulsion). Which statite is deployed simply depends upon the optimum trajectory to the incoming ISO.
We are now on a fast track toward reaching the interstellar object with at least a flyby. Linares calls this a “dynamic orbital slingshot for rendezvous with interstellar objects.” And the idea is to have a constellation of these statites always at the ready for the next ‘Oumuamua. Or, considering how odd ‘Oumuamua seems to be, perhaps I should say “the next Borisov.” Even so, with this net, who knows what we might catch?
The paper makes the case that a statite free-falling toward the Sun from an initial position at 1 AU and then deploying its sail away from the Sun at perihelion can achieve speeds of up to 25 AU/year, making it possible to deliver payloads to the outer Solar System. Now we’re in Matloff/Johnson ‘sundiver’ territory. Voyager 1 has reached 3.6 AU per year by comparison, making the statite concept attractive beyond its value as a station-keeper for quick response missions to interstellar comets/asteroids.
For more on Richard Linares’ work, see “Rendezvous Mission for Interstellar Objects Using a Solar Sail-based Statite Concept,” a white paper available on arXiv.
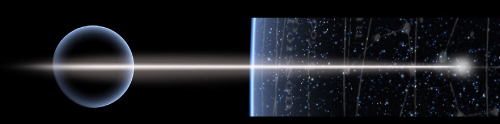