by Paul Gilster | Jul 11, 2024 | Outer Solar System |
Looking for new Kuiper Belt targets for the New Horizons spacecraft pays off in multiple ways. While we can hope to find another Arrokoth for a flyby, the search also contributes to our understanding of the dynamics of the Kuiper Belt and the distribution of comets in the inner Oort Cloud. Looking at an object from Earth or near-Earth orbit is one thing, but when we can collect data on that same object with a spacecraft moving far from the Sun, we extend the range of discovery. And that includes learning new things about KBOs that are already cataloged, as a new paper on observations with the Subaru Telescope makes clear.
The paper, in the hands of lead author Fumi Yoshida (Chiba Institute of Technology) and colleagues, points to Quaoar and the use of New Horizons data in spawning further research. A key aspect of this work is the phase angle as the relative position of the object changes with different observing methods.
One of the unique perspectives of observing KBOs from a spacecraft flying through the Kuiper Belt is that they can be observed with a significantly larger solar phase angle, and from a much closer distance, compared with ground-based or Earth-based observation. For example, the New Horizons spacecraft observed the large classical KBO (50000) Quaoar at solar phase angles of 51°, 66°, 84°, and 94° (Verbiscer et al. 2022). Ground-based observation can only provide data at small solar phase angles (≲2°). The combination of observations at large and small solar phase angles provides us with knowledge of the surface reflectance of the object and enables us to infer information about a KBO’s surface properties in detail (e.g., Porter et al. 2016; Verbiscer et al. 2018, 2019, 2022).
In the new paper, the Subaru Telescope’s Hyper Suprime-Cam (HSC) is the source of data that is sharpening our view of the Kuiper Belt through wide and deep imaging observations Located at the telescope’s prime focus, HSC involves over 100 CCDs covering a 1.5 degree field of view. Early results from Yoshida’s work support the idea that we can think in terms of extending the Kuiper Belt, whose outer edge seems to end abruptly at around 50 AU. This adds weight to recent work with the New Horizons team’s Student Dust Counter (SDC), which has been measuring dust beyond Neptune and Pluto. The SDC results point to such an extension. See the citation below, and you might also want to check New Horizons: Mapping at System’s Edge in these pages.
Other planetary systems also raise the question of why our outer debris belt should be as limited as it has been thought to be. Says Yoshida:
“Looking outside of the Solar System, a typical planetary disk extends about 100 au from the host star (100 times the distance between the Earth and the Sun), and the Kuiper Belt, which is estimated to extend about 50 au, is very compact. Based on this comparison, we think that the primordial solar nebula, from which the Solar System was born, may have extended further out than the present-day Kuiper Belt.”
If it does turn out that our system’s early planetary disk was relatively small, this could be the result of outer objects like the much discussed (and still unknown) Planet 9. The distribution of objects in this region thus points to the evolution of the Solar System, with the implication that further discoveries will flesh out our view of the process.
The Subaru work was focused on two fields along New Horizon’s trajectory, an area of sky equivalent to about 18 full moons. Using these datasets, drawn from thirty half-nights of observations, the New Horizons science team has been able to find more than 240 objects. The new paper pushes these findings further by studying the same observations with different analytical tools, using JAXA software called the Moving Object Detection System, which normally is deployed for spotting near-Earth objects. Out of 84 KBO candidates, seven new objects have emerged whose orbits can be traced. Two of these have been assigned provisional designations by the Minor Planet Center of the International Astronomical Union.
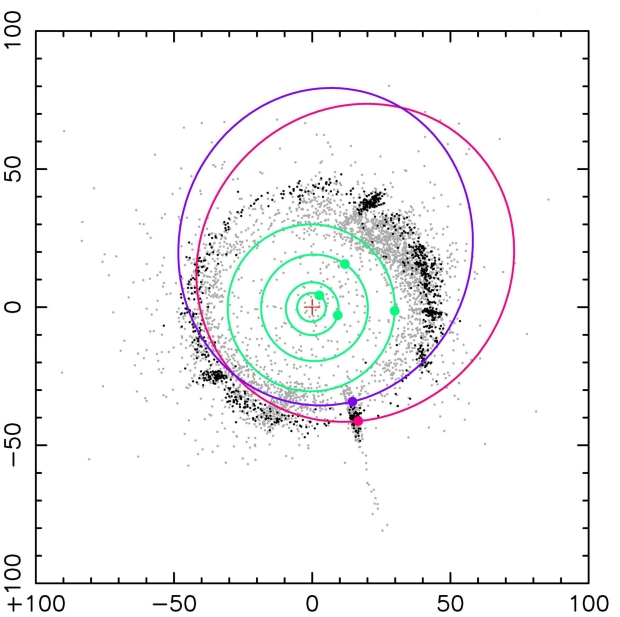
Image: Schematic diagram showing the orbits of the two discovered objects (red: 2020 KJ60, purple: 2020 KK60). The plus symbol represents the Sun, and the green lines represent the orbits of Jupiter, Saturn, Uranus, and Neptune, from the inside out. The numbers on the vertical and horizontal axes represent the distance from the Sun in astronomical units (au, one au corresponds to the distance between the Sun and the Earth). The black dots represent classical Kuiper Belt objects, which are thought to be a group of icy planetesimals that formed in situ in the early Solar System and are distributed near the ecliptic plane. The gray dots represent outer Solar System objects with a semi-major axis greater than 30 au. These include objects scattered by Neptune, so they extend far out, and many have orbits inclined with respect to the ecliptic plane. The circles and dots in the figure represent their positions on June 1, 2024. Credit: JAXA.
The semi-major axes of the two provisionally designated objects are greater than 50 AU, pointing to the possibility that as such observations continue, we will be able to extend the edge of the Kuiper Belt. Further work using the HSC is ongoing.
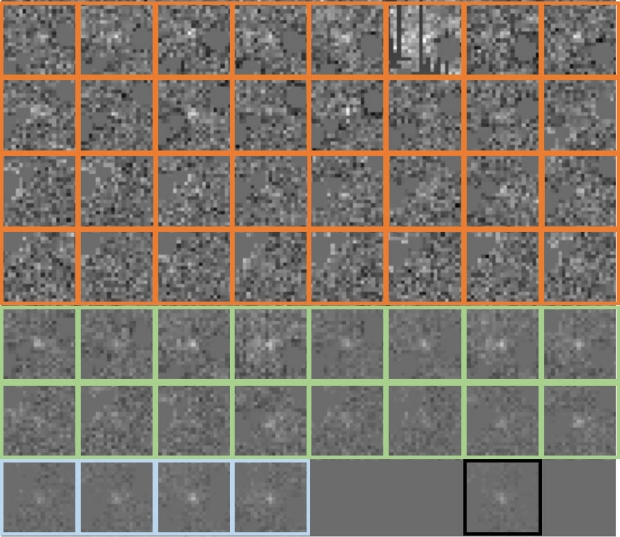
Image: An example of detection by JAXA’s Moving Object Detection System. Moving objects are detected from 32 images of the same field taken at regular time intervals (the images in the orange frames in the above figure). Assuming the velocity range of Kuiper Belt objects, each image is shifted slightly in any direction and then stacked. The green, light blue, and black framed images are the result of stacking 2 images each, 8 images each, and 32 images, respectively. If there is a light source in the center of a single image as well as each of the overlapping images, it is considered a real object. (Credit: JAXA)
Observations of tiny objects at these distances are fraught with challenges at any time, but the authors take note of the fact that while their datasets cover the period between May 2020 and June 2021 (the most recently released datasets), their particular focus is on the June 2020 and June 2021 data. This marks the time when the observation field was close to opposition; i.e., directly opposite the Sun as seen from Earth. At opposition the objects should be brightest and their motion across the sky most accurately measured.
Additional datasets are in the pipeline and will be analyzed when they are publicly released. The authors intend to adjust their software in a computationally intensive way that will bring more accurate results near field stars that can otherwise confuse a detection. They also plan to deploy a machine-learning framework as the effort continues. Meanwhile, New Horizons presses on, raising the question of its successor. Right now we have exactly one spacecraft in the Kuiper Belt. How and when will we build the probe that continues its work?
The paper is Yoshida et al., “A deep analysis for New Horizons’ KBO search images,” Publications of the Astronomical Society of Japan (May 29, 2024). Full text. The SDC paper is Doner et al., “New Horizons Venetia Burney Student Dust Counter Observes Higher than Expected Fluxes Approaching 60 au,” The Astrophysical Journal Letters Vol. 961, No. 2 (24 January 2024), L38 (abstract).
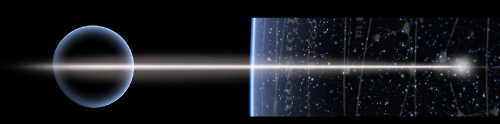
by Paul Gilster | May 24, 2024 | Outer Solar System |
What a pleasure to see new images from JunoCam, the visible-light camera aboard the Juno spacecraft that has now imaged in its peregrinations around Jupiter the surface of its most interesting moon. Our probing of Europa’s secrets has depended heavily upon the imagery returned by the Galileo spacecraft. That mission made its last flyby in 2000, and we have another wait while ESA’s Juice mission and Europa Clipper make the journey, the former enroute, the latter scheduled for an October launch.
Juno’s 2022 flyby thus gave us a helpful visual update, one that is complemented by an informative snapshot taken by the spacecraft’s Stellar Reference Unit (SRU) star camera. While we have five high resolution images to work with, the Stellar Reference Unit’s black-and-white image has produced the most detail. The image is intriguing because of its method, for bear in mind that the SRU is designed to track stars for navigation purposes. That makes it a dim light instrument, one that must be handled carefully to avoid washing out the image. The Juno team used it on Europa’s nightside, where the ambient light was sunlight reflected off Jupiter itself and the Sun was safely hidden.
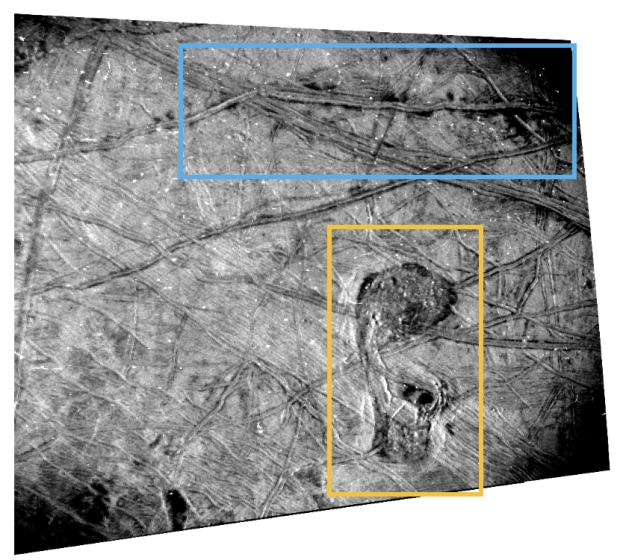
Image: From Juno’s SRU, this image shows the location of a double ridge running east-west (blue box) with possible plume stains and the chaos feature the team calls ‘the Platypus” (orange box). These features hint at current surface activity and the presence of subsurface liquid water on the icy Jovian moon. Credit: NASA/JPL-Caltech/SwRI.
What emerges is a jumble of chaotic terrain cut by ridges and laden with a reddish-brown material familiar from Galileo imagery of the moon. These dark stains have been hypothesized to be the deposits of cryovolcanic plumes. Amidst this terrain, a new feature emerges that interrupts different forms of terrain. The Juno team has christened it the Platypus. Here the ridge topography breaks down as it encounters what is clearly younger material laden with ice blocks, a disrupted area that is some 37 kilometers by 67 kilometers in size. A double ridge line north of the Platypus is also apparent, the complex terrain suggesting the kind of surface change that researchers believe may allow ocean water to come close to the surface in isolated pockets.
The mention of plumes is intriguing because of the possibility of one day collecting samples from a spacecraft during a flyby, although no plumes are evident in the Juno imagery. Both the Platypus and the double ridges suggest recent activity. On the possibility of plumes, the SRU paper notes:
Diffuse discontinuous low-albedo deposits flank double ridges ∼50 km north of the “Platypus” chaos margin, extending radially outward from the lineaments. The morphology of these deposits is similar to features observed elsewhere on Europa that have been associated with hypothesized plume activity, the discontinuous low-albedo spots flanking Rhadamanthys Linea being a prominent example (Quick & Hedman, 2020). Quick and Hedman (2020) surmise that 1–10 m thick deposits can be emplaced by sufficiently compact plumes and detected by high-resolution visible wavelength cameras. The radii of the deposits observed by the SRU are ∼2–5 km, which Quick & Hedman’s models associate with <10 km high plumes.
We can also compare the Juno imagery with that of Galileo, as the JunoCam paper does:
The number of documented craters larger than 1 km on Europa has gone from 41 to 40 craters. Careful comparisons of the JunoCam images with overlapping images from Galileo show no surface changes due to plume deposits or ongoing geologic activity over time intervals of 23–26 yr, though admittedly the images are not well matched in resolution, viewing geometry, and wavelength. No active eruptions were detected. Finally, from the Europa data set taken on 2022 February 24, we can say that the north polar cap of Europa at this image scale looks similar to lower latitudes.
It’s worth adding here that a recent search using the Atacama Large Millimeter/submillimeter Array (ALMA) collected data over four days to examine the moon’s entire surface, coming up with no evidence of plume activity. We’re clearly not dealing with a geyser phenomenon anywhere as active as what we find at Enceladus, and thus far evidence from the Hubble instrument has been the most compelling, but even the data from its 2013 observations remain at the edge of detection. Clearly the search for active plumes will continue given their exciting implications.
Meanwhile, evidence for surface activity of other kinds on Europa continues to emerge, presenting new targets for Europa Clipper as well as Juice. Juice (Jupiter Icy Moons Explorer) launched on April 14, 2023 and will arrive in July of 2031, while Europa Clipper is scheduled to reach the giant planet in April of 2030. The new imagery suggests that Europa’s outer ice shell moves freely over the ocean (“true polar wander”), capturing steep depressions up to 50 kilometers wide near the equator. These ovoid features are similar to those found in other parts of Europa. Candy Hansen, who leads JunoCam planning at the Planetary Science Institute in Tucson, AZ, notes their relevance:
“True polar wander occurs if Europa’s icy shell is decoupled from its rocky interior, resulting in high stress levels on the shell, which lead to predictable fracture patterns. This is the first time that these fracture patterns have been mapped in the southern hemisphere, suggesting that true polar wander’s effect on Europa’s surface geology is more extensive than previously identified.”
The landscape of ice blocks and troughs near Europa’s equator broken by depressions tells a tale that must be interpreted in terms of light and shadow. The feature called Gwern, for example, an apparent impact crater found in Galileo imagery, turns out under different lighting to be nothing more than an oval shadow caused by the intersection of prominent ridges. Cross-cut ridges and the dark stains that may mark the residue from ancient (or recent) plumes offer a compelling landscape. New features like the Platypus will get a particularly hard look from our incoming spacecraft.
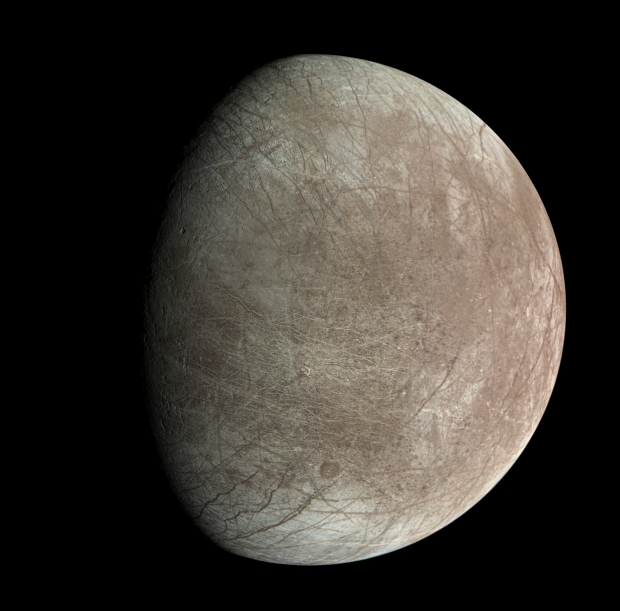
Image: Jupiter’s moon Europa was captured by the JunoCam instrument aboard NASA’s Juno spacecraft during the mission’s close flyby on Sept. 29, 2022. The images show the fractures, ridges, and bands that crisscross the moon’s surface. Credit: Image data: NASA/JPL-Caltech/SwRI/MSSS. Image processing: Björn Jónsson (CC BY 3.0).
The SRU paper is Becker et al., “A Complex Region of Europa’s Surface With Hints of Recent Activity Revealed by Juno’s Stellar Reference Unit,” JGR Planets 22 December 2023 (full text). The paper on the JunoCam imagery is Hansen, “Juno’s JunoCam Images of Europa,” Planetary Science Journal Vol. 5, No. 3 (21 March 2024), 76. Full text. The paper on the ALMA observations is Cordiner et al., “ALMA Spectroscopy of Europa: A Search for Active Plumes,” submitted to IAU Symposium 383 conference proceedings (preprint).
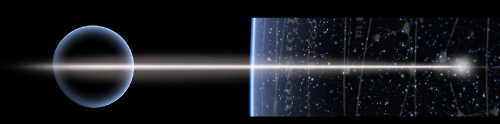
by Paul Gilster | May 21, 2024 | Outer Solar System |
Dust between the stars usually factors into our discussions on Centauri Dreams when we’re considering its effect on fast-moving spacecraft. Although it only accounts for 1 percent of the mass in the interstellar medium (the other 99 percent being gas) its particles and ices have to be accounted for when moving at a substantial fraction of the speed of light. As you would expect, regions of star formation are particularly heavy in dust, but we also have to account for its presence if we’re modeling deceleration into a planetary system, where the dust levels will far exceed the levels found along a star probe’s journey.
Clearly, dust distribution is something we need to learn more about when we’re going out from as well as into a planetary system, an effort that extends all the way back to Pioneers 10 and 11, which included instruments to measure interplanetary dust. Voyager 1 and 2 carry dust detecting instruments, and so did Galileo and Cassini, the latter with its Cosmic Dust Analyzer (CDA).
And I’m reminded by a recent news release from the New Horizons team that the Student Dust Counter (SDC) aboard New Horizons is making a significant contribution as it moves ever deeper into the Kuiper Belt. You’ll recall that the SDC played the major role in identifying what may be an extended Kuiper Belt in findings published in January (citation below). Alex Doner (University of Colorado Boulder) is lead author of the paper on that work. He serves as SDC lead:
“New Horizons is making the first direct measurements of interplanetary dust far beyond Neptune and Pluto, so every observation could lead to a discovery. The idea that we might have detected an extended Kuiper Belt — with a whole new population of objects colliding and producing more dust – offers another clue in solving the mysteries of the solar system’s most distant regions.”
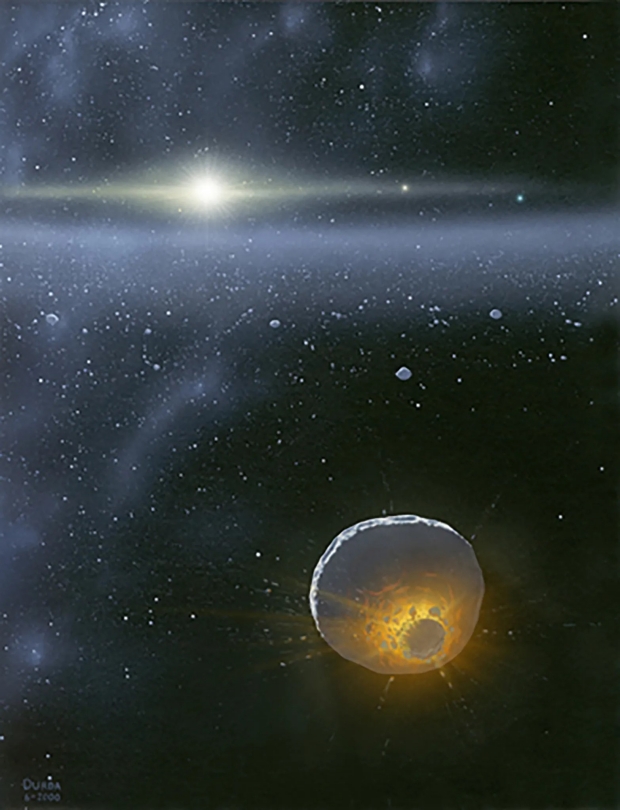
Image: Artist’s impression of a collision between two objects in the distant Kuiper Belt. Such collisions are a major source of dust in the belt, along with particles kicked up from Kuiper Belt objects being peppered by microscopic dust impactors from outside of the solar system. Credit: Dan Durda, FIAAA.
We have to account for the variable distribution and composition of dust not only in terms of spacecraft design but also for fine-tuning our astronomical observations. Scattering and absorbing starlight, dust produces what astronomers refer to as extinction, dimming and reddening the light in significant ways. It’s a part of the cosmic optical background, which on the largest scale includes light from extragalactic sources as well as our own Milky Way. This background can tell us about galactic evolution and even dark matter if we know how to adjust for its effects.
Joel Parker (SwRI) is a New Horizons project scientist who notes that even as the craft continues to make observations within the Kuiper Belt (and the search for potential flyby targets continues), its instruments are also returning data with implications for astrophysics at large:
“New Horizons is uniquely positioned to make astrophysical observations that are difficult or impossible to make here on Earth or even from orbit. Many things can obscure observations, but one of the biggest problems is the dust in the inner solar system. It may not be obvious when you look up into a clear night sky, but there is a lot of dust in the inner part of the solar system. There is also a great deal of obscuration at certain ultraviolet wavelengths at closer distances due to the hydrogen that pervades our planetary system, but which is much reduced out in the Kuiper Belt and the outer heliosphere.”
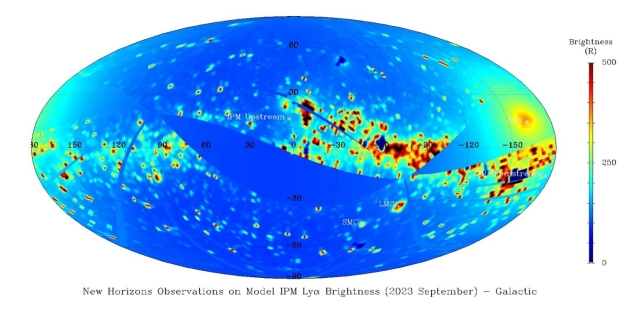
Image: New Horizons mission scientists and external colleagues are taking advantage of the New Horizons spacecraft’s position in the distant Kuiper Belt to make unique astrophysical and heliospheric observations. Alice, the ultraviolet spectrograph on the spacecraft, performed 75 great circle scans of the sky in September 2023, for a total of 150 hours of observations. These data focus on the light from hydrogen atoms in the ultraviolet Lyman-alpha wavelength across the sky as seen from New Horizons’ vantage point in the distant solar system. This map shows the data from the scans overlaid on a smoothed model of the expected Lyman-alpha background. (Credit: NASA/Johns Hopkins APL/SwRI).
We’ve recently talked about hydrogen-alpha transitions, which are a factor in astronomical observations (we saw this in the Project Hephaistos Dyson sphere papers). The Lyman-alpha transitions referred to above produce different emissions as electrons change energy levels within the atom, and are primarily useful for studies of the interstellar and intergalactic medium. So New Horizons is on point for helping us clarify how local dust levels may affect our observations of these distant sources.
Parker puts it this way:
“It’s like driving through a thick fog, and when you go over a hill, it’s clear. Suddenly, you can see things that were obscured. When you’re trying to look for a very faint light far outside our solar system or beyond our galaxy, that obscuration creates a challenge. If we measure how the ‘fog’ changes as we move farther out, we can make better models for our observations from Earth. With more accurate models, we can more easily subtract the effects of light and dust contamination.”
New Horizons records the cosmic ultraviolet background and maps hydrogen distribution as it moves through the outer regions of the heliosphere and eventually through the heliopause and into the local interstellar medium. This is going to be useful in telling astronomers something about the evolution of galaxies by yielding data on star formation rates as we learn how to remove the contaminating signature of interplanetary dust from our observations.
It’s fascinating to see how a single spacecraft can, as have the Voyagers, function as a kind of Swiss army knife with tools useful well beyond a single target. Successors to New Horizons will one day extend these observations as we learn more about dust distribution all the way out to the Oort Cloud.
The paper on a possible extended Kuiper Belt is Doner et al., “New Horizons Venetia Burney Student Dust Counter Observes Higher than Expected Fluxes Approaching 60 au,” The Astrophysical Journal Letters Vol. 961, No. 2 (24 January 2024), L38 (abstract).
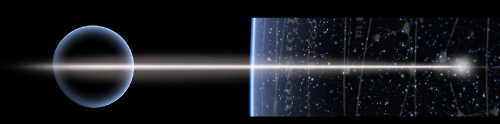
by Paul Gilster | Mar 13, 2024 | Outer Solar System |
We’re a species that likes to leave evidence of itself in new places. In Greenland, for example, the Kingittorsuaq runestone, dating from the 14th Century, offers inscriptions that help chart Norse exploration of the region. The oldest inscription at New Mexico’s El Morro dates from 1605, though many explorers left their names and stories on the cliffs there. Apollo 11’s plaque, with its “We came in peace for all mankind” is justly famous, as are the Golden Records of the two Voyagers and the Pioneer plaques, even if the latter were dogged with controversy at the time of their unveiling.
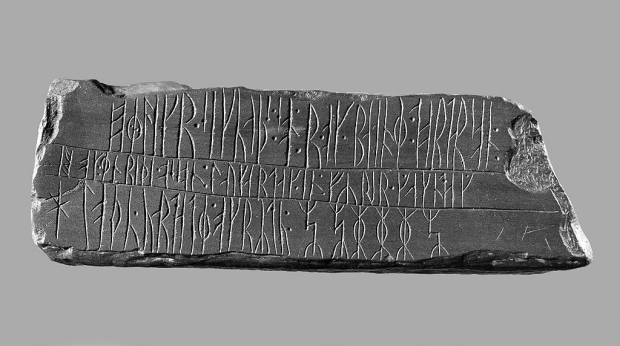
Image: The Kingittorsuaq runestone. Credit: Ukendt /Nationalmuseet, Danmark, CC BY-SA 2.5 DK , via Wikimedia Commons.
Clearly the Solar System is wide open for future plaques and markers, so that NASA’s inclusion of a plaque aboard Europa Clipper comes as no surprise. The poem it carries focuses, of course, on that intriguing moon, and I rather like poet Ada Limón’s “In Praise of Mystery: A Poem for Europa” except for its first stanza. I snag on the word ‘expansiveness,’ and the notion of a sky inky with it. The word ‘expanse’ is itself so liminal, especially as applied to an inky night sky, that it carries its own freight of awe.
To this jaded ear, ‘expansiveness’ is bloated. I can’t imagine saying ‘the sky is certainly inky with expansiveness tonight.’ So I’ll pass on stanza 1, but go for the rest of the poem, which is a deft evocation of water’s place in our evolution and our explorations:
Arching under the night sky inky
with black expansiveness, we point
to the planets we know, we
pin quick wishes on stars. From earth,
we read the sky as if it is an unerring book
of the universe, expert and evident.
Still, there are mysteries below our sky:
the whale song, the songbird singing
its call in the bough of a wind-shaken tree.
We are creatures of constant awe,
curious at beauty, at leaf and blossom,
at grief and pleasure, sun and shadow.
And it is not darkness that unites us,
not the cold distance of space, but
the offering of water, each drop of rain,
each rivulet, each pulse, each vein.
O second moon, we, too, are made
of water, of vast and beckoning seas.
We, too, are made of wonders, of great
and ordinary loves, of small invisible worlds,
of a need to call out through the dark.
Those last three stanzas form a fine conclusion; the poem ends with a satisfying click describing its mission, which is to fly aboard Europa Clipper and wind up hurtling past the target world again and again, battered by radiation as it hunts for information about an alien sea. What a good thing it is to put human artifacts on spacecraft. There is scant likelihood, of course, that the Europa poem, flying along with a microchip containing 2.6 million names submitted by the public, will one day be read by anyone, but the impulse is to commemorate and inspire ourselves. It’s something we humans do.
Image: The lower half of Europa Clipper’s vault plate, showing the poem by U.S. poet laureate Ada Limón (lower right), a drawing representing the Jovian system that will host the names of 2.6 million people flying with the mission on a microchip (top right), a tribute to planetary scientist Ron Greely (bottom left), and the radio emission lines known as the ‘Water Hole’ (center). Credit: NASA/JPL-Caltech.
As a collector and user of vintage fountain pens, I am particularly pleased to see that the poem is inscribed in the author’s handwriting, a nice touch in an era increasingly learning that writing by hand, though rarely taught these days, is actually a powerful way to explore and retain ideas. The vault plate, which you can explore here, likewise contains Frank Drake’s handwriting. Drake (1930-2022), among much else in a magnificent career, contributed the first SETI search, at Green Bank in West Virginia, and the seminal Drake Equation, which estimates the probability of finding life elsewhere in the cosmos and describes the factors critical to the discussion.
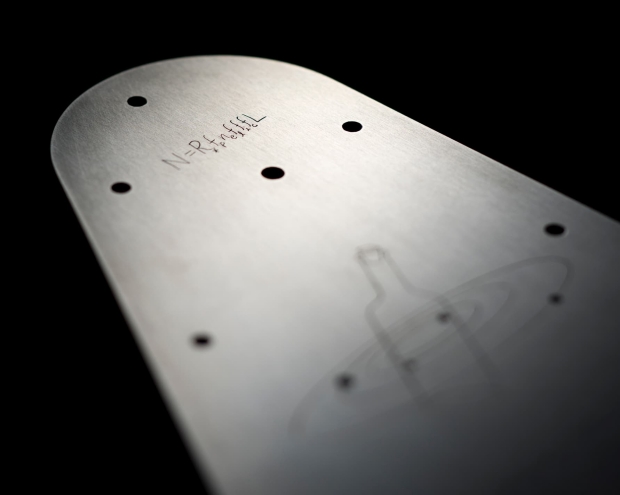
Image: The upper half of Europa Clipper’s vault plate, showing the Drake Equation in Frank Drake’s own handwriting. Credit: NASA/JPL-Caltech
The Europa Clipper vault plate is small, measuring 1 millimeter in thickness and 18 X 28 centimeters. What I’ve described so far is the inner-facing plate. The outer side contains a visual representation of the word for ‘water’ spoken in 103 languages, with the central symbol the sign for water in American Sign Language. Water, after all, is why we are probing Europa. Audio renditions of these words are contained as visual waveforms representing each sound. They look a bit runic to me.
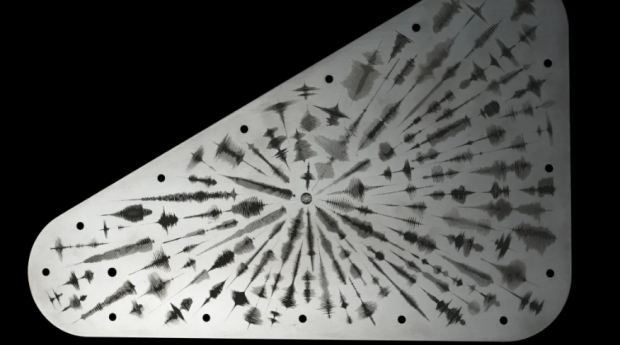
Image: The art on this side of the plate, which will seal an opening of the vault on NASA’s Europa Clipper, features waveforms that are visual representations of the sound waves formed by the word “water” in 103 languages. At center is a symbol representing the American Sign Language sign for “water.” Credit: NASA/JPL-Caltech.
We’re going to get 49 close passes of Europa if all goes well when the spacecraft arrives in 2030. The commemorative plate seals an opening in the metal vault which will protect the craft’s sensitive electronics from the sleet of particles produced by interactions between the planet and its magnetic fields. We can hope to learn a good deal more about the thickness of the moon’s icy crust, its interactions with the ocean below, the composition of that ocean, and the geology of the surface. We’re getting close to Europa Clipper’s launch, slated for October at Kennedy Space Center.
Jupiter’s radiation belts, we’ve recently learned, may play a role in what goes on in the ocean below. As a paper in Nature Astronomy explains, the bombardment of ionized particles can split any water molecules encountered on the surface, producing oxygen that could find its way into the ocean. Thus lead author Jamey Szalay (Princeton University):
“Europa is like an ice ball slowly losing its water in a flowing stream. Except, in this case, the stream is a fluid of ionized particles swept around Jupiter by its extraordinary magnetic field. When these ionized particles impact Europa, they break up the water-ice molecule by molecule on the surface to produce hydrogen and oxygen. In a way, the entire ice shell is being continuously eroded by waves of charged particles washing up upon it.”
The Juno spacecraft’s Jovian Auroral Distributions Experiment (JADE) instrument flew within 354 kilometers of the surface in September of 2022, measuring the hydrogen and oxygen ions created by the particle bombardment. The work allowed a calculation of the rate of oxygen being produced at Europa, which turns out to be about 12 kilograms per second (previous estimates have reached as high as 1000 kilograms per second). “[W]hat we didn’t realize,” adds Szalay, “is that Juno’s observations would give us such a tight constraint on the amount of oxygen produced in Europa’s icy surface.” How much of this oxygen, if any, works its way into the ocean to provide potential metabolic energy is something Europa Clipper should help us understand.
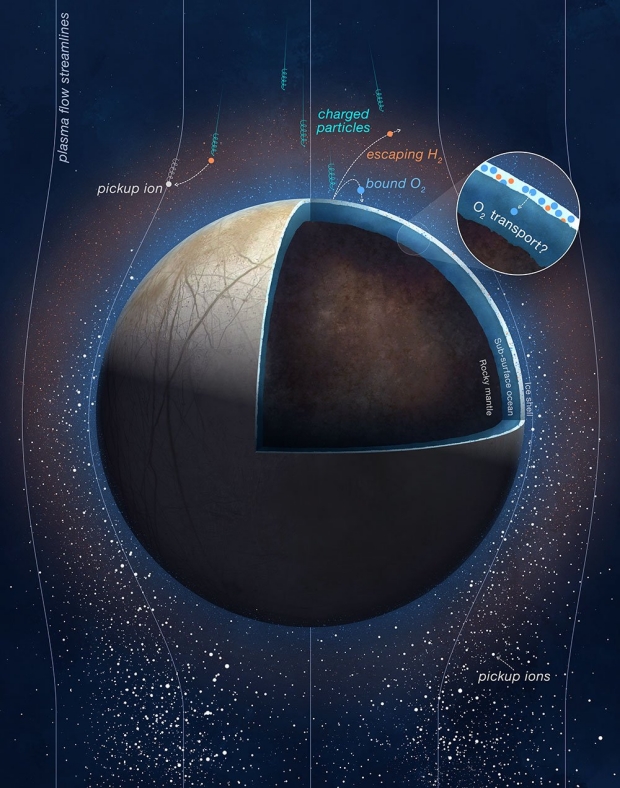
Image: This illustration shows charged particles from Jupiter impacting Europa’s surface, splitting frozen water molecules into oxygen and hydrogen molecules. Scientists believe some of these newly created oxygen gases could migrate toward the moon’s subsurface ocean, as depicted in the inset image. Credit: NASA/JPL-Caltech/SWRI/PU.
The paper is Szalay et al., “Oxygen production from dissociation of Europa’s water-ice surface,” Nature Astronomy 04 March 2024 (full text).
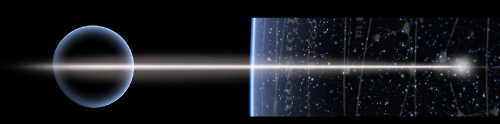
by Paul Gilster | Aug 31, 2023 | Outer Solar System |
The idea that we might take an active, working spacecraft in the Kuiper Belt and not only repurpose it for a different task (heliophysics) but also dismiss the team that is now running it is patently absurd. Yet this appears to be a possibility when it comes to New Horizons, the remarkable explorer of Pluto/Charon, Arrokoth, and the myriad objects of the Kuiper Belt. NASA’s Science Mission Directorate, responding to a 2022 Senior Review panel which had praised New Horizons, is behind the controversy, about which you can read more in NASA’s New Horizons Mission Still Threatened.
So absurd is the notion that I’m going to assume this radical step, apparently aimed at ending the Kuiper Belt mission New Horizons was designed for on September 30 of 2024, will not happen, heartened by a recent letter of protest from some figures central to the space community, as listed in the above article from Universe Today. These are, among a total of 25 planetary scientists, past Planetary Society board chair Jim Bell, Lori Garver (past Deputy Administrator of NASA), Jim Green (Past Director of NASA’s Planetary Science Division), Candice Hansen-Koharcheck (Past chair of the American Astronomical Society’s Division of Planetary Sciences and Past Chair of NASA’s Outer Planets Assessment Group), author Homer Hickham, Wesley T. Huntress (Past Director of NASA’s solar system Exploration Group), astrophysicist Sir Brian May, and Melissa McGrath (past NASA official and AAS Chair of DPS).
Needless to say, we’ll keep a wary eye on the matter (and I’ve just signed the petition to save the original mission), but let’s talk this morning about what New Horizons is doing right now, as the science remains deeply productive. I like the way science team member Tod Lauer spoke of the spacecraft’s current position in a recent post on the team’s website, a place where “It’s still convenient to think of neat north and south hemispheres to organize our vista of the sky, but the equator is now defined by the band of the galaxy, not our spinning Earth, lost to us in the glare of the fading Sun.”
Places like that evoke the poet in all of us. Lauer points out that the New Horizons main telescope is no more powerful than what amateur stargazers willing to open their checkbooks might use in their backyards. Part of the beauty of the situation, though, is that New Horizons moves in a very dark place indeed. While I have vivid memories of seeing a glorious Milky Way bisecting the sky aboard a small boat one summer night on Lake George in the Adirondacks, how much more striking is the vantage out here in the Kuiper Belt where the nearest city lights are billions of kilometers away?
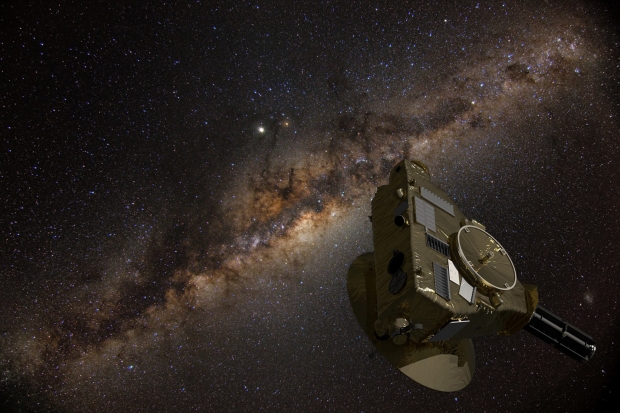
Image: A Kuiper Belt explorer looks into the galaxy. Credit: Serge Brunier/Marc Postman/Dan Durda
The science remains robust out here as well. Consider what Lauer notes in his article. Looking for Kuiper Belt objects using older images, the New Horizons team found that in all cases, even objects far from the Milky Way’s band appeared against a background brighter than scientists can explain, even factoring in the billions of galaxies that fill the visible cosmos. The puzzle isn’t readily solved. Says Lauer:
…we then tried observations of a test field carefully selected to be far away from the Milky Way, any bright stars, clouds of dust – or anything, anything that we could think of that would wash out the fragile darkness of the universe. The total background was much lower than that in our repurposed images of Kuiper Belt objects – but by exactly the amount we expected, given our care at pointing the spacecraft at just about the darkest part of the sky we could find. The mysterious glow is still there, and more undeniable, given the care we took to exclude anything that would compete with the darkness of the universe, itself. You’re in an empty house, far out in the country, on a clear moonless night. You turn off all the lights everywhere, but it’s still not completely dark. The billions of galaxies beyond our Milky Way are still there, but what we measure is twice as bright as all the light they’ve put out over all time since the Big Bang. There is something unknown shining light into our camera. If it’s the universe, then it’s just as strong, just as bright, as all the galaxies that ever were.
Only New Horizons can do this kind of work. How far are we from Earth? Take a look at the animated image below, which is about as vivid a reminder as I can find.
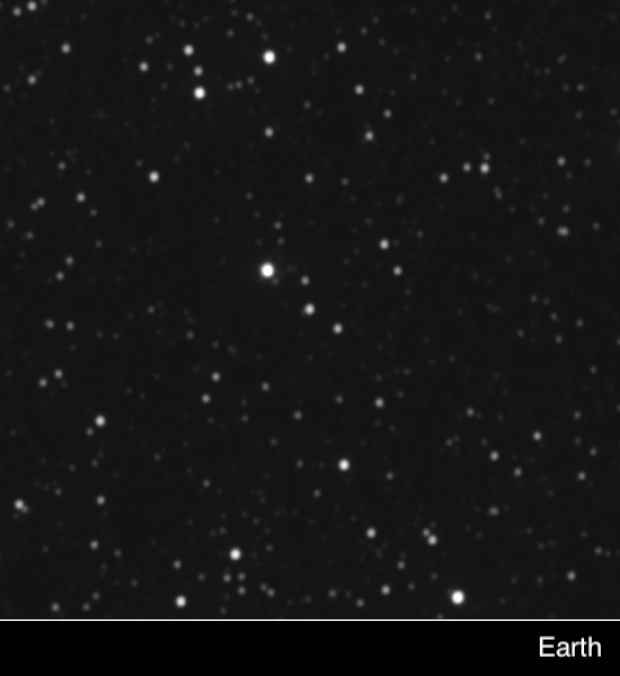
Image: Two images of Proxima Centauri, one taken by NASA’s New Horizons probe and the other by ground-based telescopes. The images show how the spacecraft’s view, from a distance of 7 billion kilometers, is very different from what we see on Earth, illustrating the parallax effect. Credit: NASA/Johns Hopkins Applied Physics Laboratory/Southwest Research Institute/Las Cumbres Observatory/Siding Spring Observatory.
With 15 more dark fields slated for examination this summer, New Horizons remains active on this and many other fronts. In his latest PI’s Perspective, Alan Stern notes the eight-week period of observations taking place now and through September. include continued observations of KBOs, imaging of the ice giants, dust impact measurements, mapping of hydrogen gas in the outer heliosphere, analysis using the ultraviolet spectrometer to look for structures in the interstellar medium, and spatial variations in the visible wavelength background. Six months of data return will be needed to download the full set for the planetary, heliospheric and astrophysics communities.
According to Stern, new fault protection software will allow New Horizons to operate out to 100 AU. That assumes we’ll still be taking Kuiper Belt data. Given the utterly unique nature of this kind of dataset and the fact that this spacecraft is engaged in doing exactly what it was designed to do, it is beyond comprehension that its current mission might be threatened with a shakeup to the core team or a reframing of its investigations. I’ve come across the National Space Society’s petition to save the New Horizons Kuiper Belt mission late, but please take this chance to sign it.
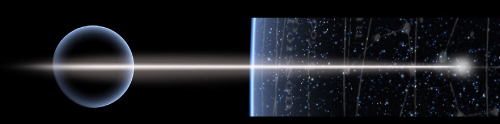
by Paul Gilster | Aug 29, 2023 | Outer Solar System |
Trans-Neptunian Objects, or TNOs, sound simple enough, the term being descriptive of objects moving beyond the orbit of Neptune, which means objects with a semimajor axis greater than 30 AU. It makes sense that such objects would be out there as remnants of planet formation, but they’re highly useful today in telling us about what the outer system consists of. Part of the reason for that is that TNOs come in a variety of types, and the motions of these objects can point to things we have yet to discover.
Thus the cottage industry in finding a ninth planet in the Solar System, with all the intrigue that provides. The current ‘Planet 9 Model’ points to a super-Earth five to ten times as massive as our planet located beyond 400 AU. It’s a topic we’ve discussed often in these pages. I can recall the feeling I had long ago when I first learned that little Pluto really didn’t explain everything we were discovering about the system beyond Neptune. It simply wasn’t big enough. That pointed to something else, but what? New planets are exciting stuff, especially when they are nearby, as the possibility of flybys and landings in a foreseeable future is real.
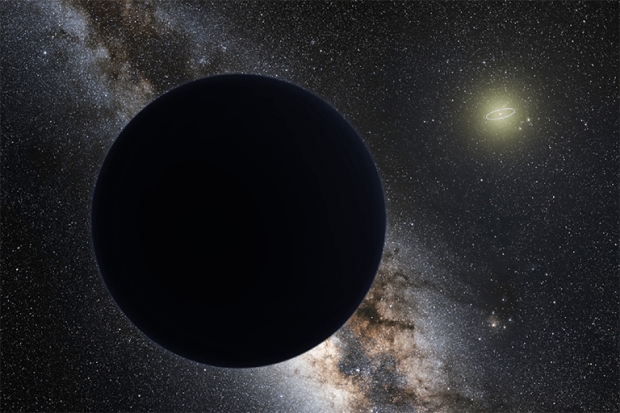
Image: An artist’s illustration of a possible ninth planet in our solar system, hovering at the edge of our solar system. Neptune’s orbit is show as a bright ring around the Sun. Credit: ESO/Tom Ruen/nagualdesign.
But a different solution for a trans-Neptunian planet is possible, as found in the paper under discussion today. Among the active researchers into the more than 1000 known TNOs and their movements are Patryk Sofia Lykawka (Kobe University) and Takashi Ito (National Astronomical Observatory of Japan), who lay out the basics about these objects in a new paper that has just appeared in The Astronomical Journal. The astronomers point out that we can use them to learn about the formation and evolution of the giant planets, including possible migrations and the makeup of the protoplanetary disk that spawned them. But it’s noteworthy that no single evolutionary model exists that would explain all known TNO orbits in a unified way.
So the paper goes to work on such a model, and while concentrating on the Kuiper Belt and objects with semimajor axes between 50 and 1500 AU, the authors pin down four populations, and thus four constraints that any successful model of the population must explain. The first of these are detached TNOs, meaning objects that are outside the gravitational influence of Neptune and thus not locked into any mean motion resonance with it. The second is a population the authors consider statistically significant, TNOs with orbital inclinations to the ecliptic above 45 degrees. This population is not predicted by existing models of early Neptune migration.
The paper is generous with details about these and the following two populations, but here I’ll just give the basics. The third group consists of TNOs considered to be on extreme orbits. Here we fall back on the useful Sedna and other objects with large values for perihelion of 60 AU or more. These demand that we find a cause for perturbations beyond the four giant planets, possibly even a rogue planet’s passing. Any model of the outer system must be able to explain the orbits of these extreme objects, an open question because interactions with a migrating Neptune do not suffice.
Finally, we have a population of TNOs that are stable in various mean motion resonances with Neptune over billions of years – the authors call these “stable resonance TNOs” – and it’s interesting to note that most of them are locked in a resonance of approximately 4 billion years, which points to their early origin. The point is that we have to be able to explain all four of these populations as we evolve a theory on what kind of objects could produce the observable result.
What a wild place the outer Solar System would have been during the period of planet formation. The authors believe it likely that several thousand dwarf planets with mass in the range of Pluto and “several tens” of sub-Earth and Earth-class planets would have formed in this era, most of them lost through gravitational scattering or collisions. We’ve been looking at trans-Neptunian planet explanations for today’s Kuiper Belt intensely for several decades, but of the various suggested possibilities, none explained the orbital structure of the Kuiper Belt to the satisfaction of Lykawka and Ito. The existing Planet 9 model here gives way to a somewhat closer, somewhat smaller world deduced from computer simulations examining the dynamical evolution of TNOs.
What the authors introduce, then, is the possibility of a super-Earth perhaps as little as 1.5 times Earth’s mass with a semimajor axis in the range of 250 to 500 AU. The closest perihelion works out to 195 au in these calculations, with an orbit that is inclined 30 degrees to the ecliptic. If we plug such a world into this paper’s simulations, we find that it explains TNOs that are decoupled from Neptune and also many of the high-inclination TNOs, while being compatible with resonant orbit TNOs stable for billions of years. The model thus broadly fits observed TNO populations.
But there is a useful addition. In the passage below, the italics are mine:
…the results of the KBP scenario support the existence of a yet-undiscovered planet in the far outer solar system. Furthermore, this scenario also predicts the existence of new TNO populations located beyond 150 au generated by the KBP’s perturbations that can serve as observationally testable signatures of the existence of this planet. More detailed knowledge of the orbital structure in the distant Kuiper Belt can reveal or rule out the existence of any hypothetical planet in the outer solar system. The existence of a KBP may also offer new constraints on planet formation and dynamical evolution in the trans-Jovian region.
A testable signature is the gold standard for a credible hypothesis, which is not to say that we will necessarily find it. But if we do locate such a world, or a planet corresponding to the more conventional Planet 9 scenarios, we will have ramped up our incentive to explore beyond the Kuiper Belt, an incentive already given further impetus by our growing knowledge of the heliosphere and its interactions with the interstellar medium. But to the general public, interstellar dust is theoretical. An actual planet – a place that can be photographed and one day landed upon – awakens curiosity and the innate human drive to explore with a target that pushes all our current limits.
The paper is Lykawka & Ito, “Is There an Earth-like Planet in the Distant Kuiper Belt?,” Astronomical Journal Vol. 166, No. 3 (25 August 2023) 118 (full text).
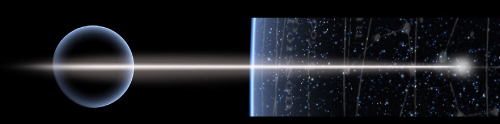