by Paul Gilster | May 8, 2024 | Culture and Society |
Larry Klaes began developing a following for his deep dives into science fiction cinema long ago on Centauri Dreams, through memorable looks at films like The Thing from Another World, Forbidden Planet and The Day the Earth Stood Still. Although he delves into recent films as well, Larry’s frequent focus on the 1950s always intrigues me, as he places these movies in the context of our developing, rapidly changing ideas about the spacefaring future. How did our views of space travel change over time as we went from Sputnik to Apollo, and where are they heading today? All of that is a subtext in today’s look at Conquest of Space, an odd and irritating take on interplanetary travel with an unusual pedigree and cultural echoes that persist.
by Larry Klaes
Imagine if the iconic ground (and space) breaking science fiction film 2001: A Space Odyssey, first released into theaters in early 1968, had actually been put together over one decade earlier – in the mid-1950s, to be more precise.
Now contemplate that film’s famous and brilliant producer, Stanley Kubrick (1928-1999), being continually badgered and overruled by Hollywood studio executives who – while being neither terribly aware of nor interested in the genre, to say nothing of science in general – want this pioneering cinematic work to follow the more standard action and melodrama formulas of the day to ensure a paying audience. To top it off, these same meddlers want to be stingy with the film’s budget, one which needs to be relatively robust in order to pull off the impressive sets and special effects planned for this production.
Had this speculative scenario on Hollywood tampering with 2001 succeeded in our reality, the results would probably have resembled Conquest of Space, a science fiction film which premiered in April of 1955, courtesy of legendary producer George Pal (1908-1980) and Paramount Pictures Corporation.
Inspired by a landmark 1949 book of the same title, Conquest of Space (or, for brevity, just Conquest) is not terribly well known or popular outside of space and science fiction aficionado circles today, unlike 2001 and other certain films of its genre. This list includes SF cinematic pieces released right around the same time as Conquest such as This Island Earth (1955) and Forbidden Planet (1956).
Why is this? Well, to sum it up in one review comment I read while conducting research for this essay, Conquest of Space is “a B-movie with A-movie values.”
Unlike the other films and documentaries I have reviewed and discussed in the past, Conquest is the first film in this collection I have not seen multiple times before now. This came about in no small part as Conquest is a flawed work when it comes to just about every aspect a film can possess. Conquest even has trouble falling neatly into the category of “so bad it is good” that is given much more easily to many low-grade science fiction and horror films of its era.
Producer Pal strove mightily to make a good film on the level of his earlier masterpiece, Destination Moon (1950), and his efforts do shine in certain places in Conquest. However, the aforementioned interference from Hollywood executives turned Conquest into an awkward hybrid that was neither cheesy enough to entertain audiences at a base level nor polished enough to influence most viewers in a positive direction towards supporting real space exploration.
When I did finally view Conquest over a decade ago now, there was little motivation beyond a combination of curiosity, being a fan of science fiction in its multiple forms, a deep interest in pre-Space Age depictions of humanity in the Final Frontier, and a sense of completion.
Well before the end credits appeared, I felt little compulsion to see Conquest again any time soon. I was less than enchanted with most of the characters, some of whom I came to actively dislike: This state was made even worse by the fact that no one in the film was meant to be an outright villain. Their dialogue often ranged from dull to awful to ridiculous. Most of the attempts at humor felt shoehorned at best, when not being downright juvenile. There was also an air of melancholy throughout Conquest that the makers seem to have mistaken for drama, which only increased as the plot went along. By the time Conquest approached its conclusion, I no more wanted to be on that deep space expedition than the men who did take that fictional journey.
My initial reactions to Conquest were mirrored by those who were its first audiences nearly seven decades ago: Film critics were not much enchanted by Conquest outside of its special effects. Initial box office returns were well under the film’s budget of 1.5 million U.S. dollars (over seventeen million in 2024 U.S. dollars), an unforgivable sin in Hollywood both then and now. All this and more are what kept Conquest from joining its more illustrious and popular fellow genre films in the higher echelons of the science fiction cinema pantheon.
In contrast, Pal’s cinematic classic from 1950, Destination Moon (DM), succeeded where Conquest did not, despite having its own fairly two-dimensional characters: They included a rather infamous stereotyped comic “relief” from Brooklyn, who was also there as an Everyman to answer the audience’s more scientific and technical questions.
Destination Moon had two things going in its favor, however: Most importantly, the film refused to compromise on its primary intention: To depict a crewed expedition to Earth’s natural satellite as realistically as possible for its day. In addition, DM arrived at a time and place where few science fiction films before it had presented space travel in such a manner: One classic exception was the German film Woman in the Moon by producer Fritz Lang (1890-1976), released in 1929. Among its multiple technical feats, Woman included the first depiction of a rocket launch countdown that had been added for dramatic effect.
Pal’s earlier creation also appeared years before the official start of the Space Age in an era where, despite the fact that both the United States and Soviet Union had been sending rockets into near space since the end of World War 2, most people considered the idea of putting humans on the Moon a distant – yet still exciting – fantasy.
By the time Conquest came along five years later, there had been numerous genre films with space missions as a main theme (although relatively few could claim to be very scientifically and technically plausible) released into theaters worldwide. The advent of actual space exploration seemed much closer than it did at the start of the 1950s, with both Cold War superpowers making serious claims of launching the first Earth orbiting satellites and even missions to the Moon just a few years into their future. As a result, Conquest had a lot more to live up to than its predecessor beyond just being about a manned rocketship voyage to another world.
For those who want to learn more about Destination Moon, check out this excellent essay authored by Albert A. Jackson here:
https://www.centauri-dreams.org/2020/06/12/destination-moon-a-70th-anniversary-appreciation/
Once More unto the Breach, Dear Friends…
Despite my less than enchanted first impressions of Conquest of Space, I felt compelled over time to see what diamonds might be buried in that film amongst all the cinematic dirt. After all, with Conquest, George Pal did attempt to make yet another good science fiction film based on realistic plans for both space vessels and expeditions. In addition, his track record of past excellent productions was certainly no fluke.
This time around, I learned among other things that Conquest may have had an important influence on 2001: A Space Odyssey, of all films. You will find a video that highlights where in Conquest that Kubrick may have been inspired for his own creation in the “References” section at the end of this essay.
This very deed alone is enough to give Conquest a second look. We will also examine what the film both did and did not do right as a learning exercise regarding filmmaking and human space exploration, as well as both a tribute and time capsule as a classic member of both early science fiction cinema and a promoter of our species’ real desire to reach into outer space.
A Distinguished Pedigree
Conquest of Space was the result of a growing interest in rocketry and space exploration begun earlier in the Twentieth Century. This fascination with reaching the vast realm beyond Earth only accelerated after the technological advancements and geopolitical changes spawned by World War 2.
One of the results of this interest was a book, titled The Conquest of Space, authored by science writer and rocket pioneer Willy Ley (1906-1969) and illustrated by legendary space artist Chesley Bonestell (1888-1986). The book had no written story to speak of, but it did lay out in a blending of words and art various plans for how humanity could explore and settle the Sol system at a time (the early Cold War era) when only a relative handful of rockets had penetrated space for a mere matter of minutes.
FIGURE 1. Where it all began: The cover of the first edition of The Conquest of Space (1949), depicting an imagined manned landing on the Moon with an iconic silvery spire spaceship and jagged lunar mountains in the background.
Conquest is a beautiful book that impresses even in today’s world built upon decades of thousands of real satellites and space vessels across the void from Earth orbit to the interstellar realm. In the years just before the advent of the Space Age, this adept confluence of art and science was nothing less than stunning to those who read it. The artwork alone, created by an artist with exacting standards for scientific and technical realism, is enough to see why this book inspired Pal to want to turn it into a film and how it influenced others such as science fiction author Arthur C. Clarke (1917-2008), who would work on both the film and novel versions of 2001: A Space Odyssey.
COMMENT: There was an even earlier book of the same title authored and self-published in 1931 by David Lasser (1902-1996), who became both the founder and first president of the American Interplanetary Society, now known as the American Institute of Aeronautics and Astronautics (AIAA).
This Conquest of Space was the first English language book to describe spaceflight and rocketry in a straightforward way: Lasser’s work included the depiction a fictional Moon mission infused with contemporary scientific and technical information. Among those who considered this book to be a life and career turning point for them was author Clarke.
FYI: Conquest was brought back into print in 2002 thanks to Apogee Books. You may also find a fascinating interview with Lasser here:
https://epizodsspace.airbase.ru/bibl/inostr-yazyki/iaa/2010/Ciancone_Lasser_David_Lasser_An_American_Spaceflight_Pioneer.pdf
The other key place where Pal et al borrowed spaceship designs and other ideas for the film Conquest was a series of articles published between 1952 and 1954 in Collier’s, a popular magazine of the day. The publication had hosted the First Symposium on Space Flight on October 12, 1951, at the Hayden Planetarium in New York City and shared the fascinating results in its pages over a two-year period.
This influence is apparent in the very first issue reproduced here, where the opening of Conquest mirrors the artist’s depiction of a giant wheeled space station and winged rocket ship in Earth orbit:
http://dreamsofspace.blogspot.com/2012/03/colliers-march-22-1952-man-will-conquer.html
You can review a complete list of each Collier’s article and their issues here:
https://www.rmastri.it/spacestuff/wernher-von-braun/colliers-articles-on-the-conquest-of-space-1952-1954/
The Collier’s magazine articles were later collected, revised, and expanded into three books published by The Viking Press of New York, all of which I highly recommend
acquiring, if possible:
• Across the Space Frontier (1952)
• Conquest of the Moon (1953)
• The Exploration of Mars (1956)
The very same articles were also big influences for a series of complimentary television episodes for Walt Disney as part of their Tomorrowland setting. The first two episodes, “Man in Space” and “Man and the Moon”, premiered in 1955, with the former program hitting the airwaves just one month before the arrival of Conquest in the cinemas. The third installment, “Mars and Beyond”, debuted just two months after the beginning of the official Space Age with the launch of the Soviet satellite Sputnik 1 on October 4, 1957.
The Disneyland series did utilize stories to showcase how contemporary experts envisioned space expeditions into Earth orbit and beyond to the Moon and Mars. However, they mainly existed to describe the mission and technical details of these journeys; there was no real character development to speak of.
I was particularly struck by one scene in “Man and the Moon” where two orbiting astronauts discover what looks like the remains of an artificial structure on the surface of the lunar farside. However, neither the explorers nor the episode narrator make any comments or even visibly react on what should be a startling find. The incident is never brought up again.
Thankfully for real science and humanity this was just a fictional event, as I cannot imagine even the most stoic astronaut or scientist not responding in some form to what appeared to be an alien artifact on our neighboring world. Had such an object been found in Conquest, one could at least be guaranteed that our characters would most definitely react to it, and rather loudly at that.
OBSERVATION: I get that Disney wanted to present a sober and serious program on a subject that the general public and even some professionals of the day had trouble taking as near-future reality, but for astronauts to witness something on the Moon likely made by non-human beings and do apparently nothing about it is in certain respects worse than turning the spectacle into some kind of overblown space fantasy. When the Apollo astronauts were both circling and walking upon our celestial companion just over one decade later, they were often heard exclaiming at all sorts of natural views there, and the majority of these men were trained test and combat pilots not usually given to emotional outbursts, at least while on the job.
Although never official, Conquest of Space could also be seen as a sequel of sorts to the films that George Pal made for Paramount Pictures Corporation, starting with Destination Moon in 1950, a painstakingly scientific and technically plausible depiction of a manned lunar expedition using a nuclear-powered rocketship christened Luna, appropriately enough.
Producer Pal then followed up this cinematic pioneering triumph with two different takes on space travel: In 1951’s When Worlds Collide, a vessel not too different in the V-2 rocket style design from Luna – only much larger and launched from a long rail going up a mountainside – is used to save a rather small slice of the human race when a rogue exoplanet collides with Earth and obliterates our globe.
COMMENT: The failure of Conquest at the box office and the difficulties in making that film kept Pal from his plans to produce a sequel to When Worlds Collide, which would very likely have been titled After Worlds Collide and based on the 1934 novel co-written by Edwin Balmer and Philip Wylie.
Two years later, Pal released The War of the Worlds, his take on H. G. Wells (1866-1946) famous science fiction novel first put together in print in 1897. This time the spaceships came from another planet, an ecologically ancient and dying Mars, where they were built and utilized by its natives not to explore the Sol system but rather to attempt an invasion and conquest of Earth to make into their new home.
COMMENT: Chesley Bonestell contributed his talent to both When Worlds Collide and The War of the Worlds: He made a wonderful matte painting of an alien landscape in the former film and gave us an exquisite visual tour of all the major members of our planetary neighborhood in the latter – with the notable exception of Venus, which was still such a mysterious world in the early 1950s due to its relentlessly thick blanket of globe-spanning clouds.
Before the era of directly examining other worlds with space probes, many scientists considered it possible that Venus was conducive to life, as that planet was nearly as large as Earth and covered in those obscuring clouds, indicating a substantial atmosphere. This is why, in the original novel, Wells had his Martians attempt to colonize the second world from Sol after their ultimate defeat on Earth, where their invading forces had been “slain by the putrefactive and disease bacteria against which their systems were unprepared; slain as the red weed was being slain; slain, after all man’s devices had failed, by the humblest things that God, in His wisdom, has put upon this Earth.”
In his other contemporary astronomical works, Bonestell painted Venus as both a roasting desert and a wet jungle full of dinosaur-type creatures: Two contrasting venues that were considered scientifically plausible by various astronomers of the day. Contemporary scientists even played with the possibilities of Venus being globally covered by vast seas of water, oil, and even seltzer!
When the first attempts to reach the surface of Venus were being made by the Soviet space program in the 1960s, their spherical landers were designed to float in a liquid medium. They stuck with this plan even after the American flyby probe Mariner 2 had determined in December of 1962 that the global surface temperature of the second planet averaged over 800 degrees Fahrenheit, or 427 degrees Celsius, far above the boiling point of water. Later missions would revise that temperature to be over one hundred degrees higher. Recent studies show that Venus may once indeed have had oceans of liquid H2O, but they evaporated eons ago.
You may watch the title sequence opening to The War of the Worlds here:
https://www.youtube.com/watch?v=AZQTKs2YDPc
With these film successes, plus the multiple events that placed humanity just a few years from reaching space in a more permanent manner, Pal felt it was time to expand upon what he started with Destination Moon and create an even grander reality-based space adventure, with those fantastic recent books and periodicals serving as the ideal templates for his vision. They just needed a story with fleshed-out characters to tie all those amazing visuals and technological details together to make a popular level film.
Thus, the cinematic Conquest of Space was born.
The Day After Tomorrow… or Maybe the Week After Next
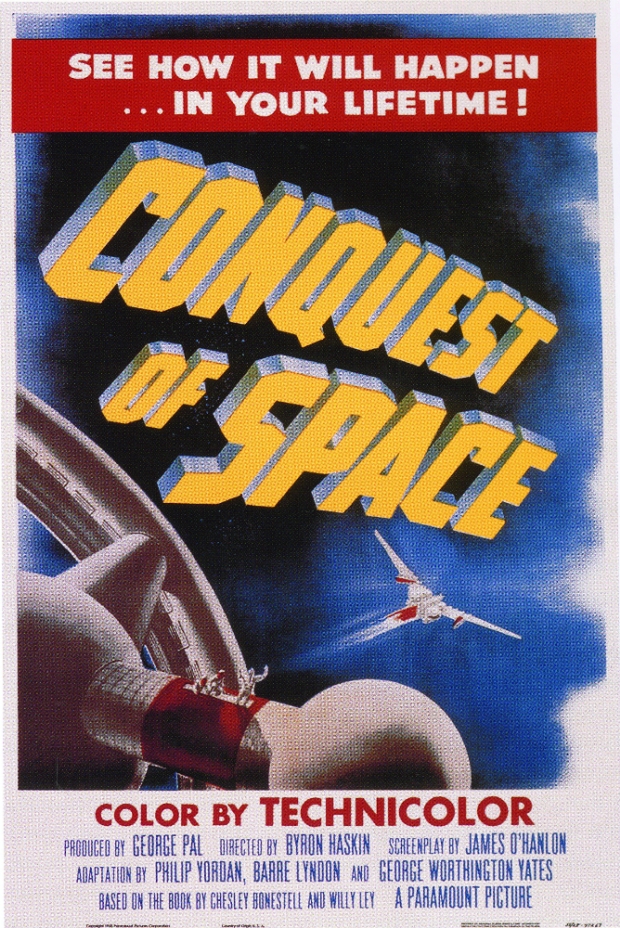
FIGURE 2. The theatrical poster for Conquest of Space, declaring how what we see in this film will happen “in your lifetime!”
With the primary introductions and background information now presented, it is time to look and comment upon Conquest of Space in depth. Naturally, there will be major plot spoilers throughout our journey together, so you may want to view the film before continuing with this essay. If you have not yet seen Conquest, or you have but still desire a refresher, one way you can view the film is through the appropriate links in the “References” section after the main body of this essay.
Conquest is said to take place sometime in the then-future decade of the 1980s, possibly the exact year of 1980. However, outside of some impressive space technologies and multiple large viewscreens, we residents of the Twenty-First Century might be forgiven while watching this film for thinking the story occurs in the 1950s or perhaps even the 1940s, judging by the World War 2 era stereotypes, styles, and mannerisms of the characters and the story elements we will encounter.
Our film opens in outer space over the predominantly blue curvature of the planet Earth. Two clearly artificial structures appear above our cosmic home in the center of this scene: On the left is a large, wide space vessel resembling a fixed-wing aircraft with an attached rocketship and four spherical fuel tanks in the center of this configuration. To our right is a gleaming white and spinning disc-shaped space station. Much further in the background shine the stars of the Milky Way galaxy, specifically those belonging to our section of this immense stellar island, the Orion Arm. This vast river of suns seems to be beckoning to those intelligent and capable enough to reach out for them.
We barely have time to grasp all this when the disembodied voice of a male narrator deeply intones when and where we are in literal time and space…
“This a story of tomorrow, or the day after tomorrow, when men have built a station in space, constructed in the form of a great wheel and set a thousand miles out from the Earth, fixed by gravity and turning about the world every two hours, serving a double purpose: An observation post in the heavens and a place where a spaceship can be assembled and then launched to explore other planets and the vast Universe itself, in the last and greatest adventure of mankind, a plunge toward the… conquest of space!”
Cue very dramatic orchestral music as the rocketship noisily blasts off into the Final Frontier in a cluster of smoke, flames, and sparks. The film title appears in large golden block letters, followed by the opening credits – none of which list any of the actors and the characters they play, which is unusual for a film of its day: One possible reason for this is that there were no big name actors in Conquest who would be considered box office draws.
To see the opening of Conquest for yourself, go here:
https://www.youtube.com/watch?v=pWINrpzDQCY
Let us step back for a moment to take a closer look at the introduction to our film:
As the camera closes in first on the rotating space station, we are told that this “great wheel” built by men is “set a thousand miles out from the Earth,” where it orbits our planet every two hours.
Just a few years after Conquest arrived on the scene, in 1958 to exact, scientists would discover that – thanks to the scientific instruments aboard the first successful American satellite Explorer 1 – the planet they reside upon is girdled by two main zones of energetic charged particles known as the Van Allen Radiation Belts. Trapped by our planet’s magnetosphere, these fields of radiation, which extend from 400 to 36,040 miles (640 to 58,000 kilometers) above our globe, can be intense enough to damage satellite equipment and cause physical harm to humans who linger there for long periods of time. Unless this cinematic space station has extra layers of shielding, the “great wheel” would have to be relocated much closer to Earth to avoid these belts and give its occupants further protection from solar flares and cosmic rays.
The narrator then explains that the space station has a “double purpose” for being up there: As an “observation post in the heavens” to look both down at Earth and up into the rest of the Universe and as an assembly yard for building vessels that can be launched towards other worlds in our Sol system and some day beyond into the rest of the Milky Way galaxy. This latter plan is emphasized by the image of the winged spaceship dominating the screen.
Early plans for space stations were epitomized by the one we see in Conquest: The spinning wheel design meant to provide a form of gravity for its human crew using centrifugal force; a “high ground” platform to monitor important activities on Earth such as global weather patterns and military actions; and finally, as a place to put together large spacecraft (shipped up in pieces from our world below via multiple rocket transports) to be sent virtually anywhere humanity wanted to explore in our planetary system.
Conducting meteorology from space and watching the actions of a nation’s rivals from Earth orbit have indeed become among the biggest reasons for operating in near space, along with international communications – although these functions are most often conducted by artificial satellites located in geosynchronous orbits averaging 22,236 miles (35,786 kilometers) in altitude, where they can match the rotation speed of our planet and thus seem to hover over one particular region.
While space engineers and scientists of the 1950s knew the first vessels to be sent into Earth orbit would be automated satellites, the technologies of the day were often considered still too bulky and cumbersome to provide more intricate work such as spying on other nations from hundreds of miles up. This is why the wheeled space station concept manned by dozens of personnel – and dominated by members of the military – watching over our world for any suspicious rival activities, up to and including surprise missile launches, were considered the best option for keeping the Cold War from turning into a hot one. At the very least, it was hoped that if the “other side” decided to start World War 3, we would not be caught off guard and could retaliate in kind, thanks to our ever-vigilant eyes in the sky.
COMMENT: In 1949, German rocket pioneer Wernher von Braun (1912–1977), whose ideas for future space utilization and exploration were among the major influences of the space vessels and plans for Conquest, wrote a science fiction novel he titled Marsprojekt, or The Mars Project in English. Von Braun created a story of the first manned expedition to the Red Planet set in the 1980s to explain in a popular manner how he envisioned such a voyage might come about.
According to the background history of this tale, we learn that humanity underwent a nuclear conflict in the 1970s which was won by the West when they used an orbiting space station named Lunetta to fire nuclear missiles upon the Soviet Union. Not long after this war, which established the United States of Earth as the dominating global power, a large reflector telescope on Lunetta determines that the famous Canals of Mars are real structures built by technologically advanced Martians and not mere natural features or optical illusions as some terrestrial astronomers had claimed. An expedition is funded and constructed at the space station, which includes three winged vessels designed to land on the fourth world from Sol. One of the mission goals includes determining if the Mars natives are either friendly or hostile.
In our history, the United States Air Force (USAF) seriously considered using an occupied space station to conduct reconnaissance operations from orbit: The effort was called the Manned Orbiting Laboratory, or MOL for short. The project got as far as the successful launching of an unoccupied station mockup in late 1966, but it was eventually determined that uncrewed satellites could do the spying much better and far more cheaply in comparison. MOL was cancelled abruptly in June of 1969.
The Union of Soviet Socialist Republics (USSR) did build and launch two working space stations under their Almaz (Diamond) reconnaissance program in the early 1970s. Publicly labeled Salyut 3 and Salyut 5, the cosmonauts aboard these cylindrical stations did perform some clandestine spy work, but even their officials concluded that robot spysats were just more efficient for this task.
That satellite telescopes can see the heavens much more clearly than their ground-based counterparts buried under those turbulent and cloud-strewn layers of terrestrial atmosphere has been known for a long time. The general public is well familiar with the astronomical results beamed back by the Hubble Space Telescope (HST) and most recently by the James Webb Space Telescope (JWST), revolutionizing our knowledge of the Universe in the optical and infrared spectrums, respectively.
Crewed space stations have also gotten in on the astronomical act: To cite just two historical examples, in the early 1970s, the United States Skylab astronauts observed our yellow dwarf star Sol in unprecedented detail thanks to their station’s Apollo Telescope Mount (ATM). They also monitored and recorded the activities on Comet Kohoutek: Their vantage point allowed the astronauts to witness this ancient ice ball when it neared Sol that astronomers stuck on Earth could not.
As for building and launching missions to other worlds, no real space station has yet been a part of such a major undertaking, unless you want to count the fair number of CubeSats that have been ejected from the International Space Station (ISS) to circle Earth for a wide variety of purposes.
Nevertheless, as global space utilization and industrialization continue to grow, there will one day be the equivalent of drydocks in Earth orbit. Space vessels will be built in sections on our planet and other places such as the Moon or a planetoid fitted for this task and then shipped via rocket to these special stations where teams will assemble them for missions all over the Sol system, just as Conquest of Space predicted. For as science fiction author Robert A. Heinlein (1907-1988) and several other prominent folks have said: “Once you get to Earth orbit, you’re halfway to anywhere in the Solar System!”
For more on this quote and concept, see here:
https://space.stackexchange.com/questions/35015/where-did-heinlein-say-once-you-get-to-earth-orbit-youre-halfway-to-anywhere
The Wheel
Once the opening film credits of Conquest end, we move back to the winged rocketship and space station. As the camera slowly closes in on the rotating station, the wordless scene is accompanied by ethereal background music that reminds me of the “Neptune” segment from The Planets, composed by Gustav Holst (1874-1934) between the years 1914 and 1917.
COMMENT: I would be surprised if this piece was not influenced by Holst’s work. If you would like to compare what is presented here in Conquest (and will be again later in the film almost every time the ship is shown moving through deep space) with Holst’s “Neptune”, visit here: https://www.youtube.com/watch?v=9BAFirzGlFk
We fade into the station, where we see two men in beige uniforms working at some controls. In the background along a wall are rows of instruments resembling face clocks with hands.
“Rocket coming up, sir,” one of the men in beige, a Corporal, relays to his commander, who suddenly appears in the frame with another officer, both of whom are wearing blue uniforms and much darker color caps. They all stare down together at what is revealed to be a small viewscreen, where a rocketship spewing white exhaust is shown heading towards them, with Earth in the background filling the screen.
“It’s the transport, right on schedule, eh, Captain?” declares the Corporal.
“No, sir, they’re late,” contradicts the Captain. “A minute and 33 seconds.”
“It’s a minute and 34 seconds, Captain,” corrects the other officer, a Colonel. “It’s not important, of course, but it could be. In celestial navigation, one second can be the difference between life and death.”
COMMENT: The Colonel is of course quite correct, although one might initially be wondering why he would need to tell this to a fellow officer working aboard the space station. One possible answer is that the Colonel considered this a teachable moment and did not want to pass it up. Another answer is that the comment was dually meant for the film’s contemporary audience, who were not generally expected to know much about celestial mechanics and rocketry. This will certainly not be the last time we witness a character committing to a bit of exposition for these very reasons.
Still looking down at the screen, the Corporal excitedly exclaims: “Gee, I hope they don’t forget to bring up the ice cream this time!”
Smiling at this seemingly innocent spoken desire, the Corporal turns to the two officers expecting them to agree with him, only to see the Colonel staring at him sternly. Realizing he has made a verbal slip, the Corporal suddenly stands stiffly at attention and partially faces away from the two officers.
“I thought I issued an order to the effect that food was never to be a subject of conversation on the Wheel!” the Colonel barks at the unfortunate man.
“I’m sorry, sir, I forgot.”
“There are some men aboard who are not permitted to enjoy the food that you eat, Corporal, and unless you’re anxious to share their diet, I’d advise you not to forget again.”
The Corporal assures his senior officer that he will definitely remember this particular “lesson.” The two officers walk off and head towards a nearby hatch. As the Captain opens the heavy metal door, the Colonel gives the Corporal one final warning look before the two officers depart the control room.
The two men enter another section of the Wheel. The Colonel, whose name is Samuel T. Merritt, activates some switches and dials on a panel labeled Observation Screen. A magnified image of the full phase Moon appears on the wall before them, with the Milky Way somehow showing behind the large bright sphere. Samuel and the Captain, who is in fact his son, Barney Merritt, meaningfully stand in front of the Moon from our perspective.
COMMENT: Although we never actually see it, nor is it even mentioned specifically in the film, this magnified display of Earth’s only natural satellite is evidence that the Conquest space station has a telescope designated for astronomical work, an “observation post in the heavens” as the narrator stated at the opening to this film. This scientific instrument was always a part of the orbital station design as envisioned by Wernher von Braun and others. The onboard telescope is meant for examining the other worlds of our Sol system up close without atmospheric interference as a prelude to their exploration via spaceship.
Looking at the projected lunar image, Samuel makes some wistful commentary to his son which also reveals the purpose of that winged spaceship parked near the station.
“The Moon, Barney. A few days, a month, and we’ll be on it,” declares Sam, who is in the process of retrieving a pack of cigarettes from his lower jacket pocket with his right hand and placing one of them in his other hand.
Barney, however, has other thoughts on his mind than exploring Earth’s celestial companion.
“Do you realize, sir, that I’ve been up here a full year without any leave?”
“There are several of us in the same boat, Barney,” reminds his father.
Barney protests that he had “only been married for three and a half months” before being essentially commandeered to serve on the space station.
“I’m sure Linda will understand,” Samuel responds about his son’s spouse. “She’s a sensible girl. After all, when a girl marries a soldier…”
“Soldier!” Barney retorts, strongly enough that his father stops placing that lone cigarette in his mouth in mid movement. “Ghost, you mean. A robot spinning around the world every two hours on a tin doughnut! That’s what you’ve been to Mother for three years – and what I’m becoming to my wife!”
Samuel shouts out his son’s name in a shocked and offended reply, but Barney continues his rant.
“I’m sorry, sir. You built the Wheel, and you’re proud of it. You’ve got every right to be, but… well, why me? We were happy down there. A little cottage right on the base, she was just beginning to furnish it, and you yank me out of it.”
“You belong here, Barney. You’re my son. Space is your heritage.”
Samuel’s offspring is neither impressed nor deterred by his father’s heartfelt declarations.
“I formally request, sir, that inasmuch as service on the Wheel is voluntary and I have never been accorded the privilege of volunteering, that I be granted permission to return to Earth on the transport rocket.”
At that moment, another man in a beige uniform enters the section with some news for Samuel.
“Colonel, sir, there’s a storm building up in the Pacific. A real lulu. Might be a hurricane.”
“Chart it and notify all weather stations likely to be affected,” orders Samuel.
“Yes, sir,” is the beige man’s succinct reply before he heads off to do as he is told.
COMMENT: In addition to creating a dramatic pause, this moment symbolizes what the public would learn just a few years hence: That observing Earth’s weather from space will become a huge boon to the field of meteorology, especially in the detection and monitoring of hurricanes and other cyclonic storms. Thematically, this moment can also be seen as an allegorical point that the story is about to become stormier for the characters – which it will.
Samuel walks over to the viewscreen and shuts it off. The Moon disappears, replaced with blackness.
“Permission denied, Captain,” is the Colonel’s blunt response.
With obvious frustration and anger, Barney quickly storms out of the observation section without saying another word. The father merely watches after his son while lighting up his cigarette and finally inserting it into his mouth.
COMMENT: This space station must have a very efficient air filtration system to allow smoking in such a relatively confining place where one cannot simply open a window if they want fresh air or step outside unfettered. This is also another cultural clue that it may be circa 1980 in the film, but circa 1955 in most other respects.
To be fair, in the real year of 1980 there was still a lot of public smoking going on, even though the serious health dangers to inhaling carcinogens had become widely known in the previous two decades.
In the book Across the Space Frontier (1952), written by Joseph Kaplan, Wernher von Braun, et al, they had this to say about crewmen smoking onboard their space station concept, which was the template for the Wheel in Conquest:
“Even smoking will probably be strictly rationed, partly to save oxygen and partly to avoid overloading the capacity of the air-conditioning unit.”
IRONIC SIDE NOTE: During the first press conference for the seven chosen astronauts of Project Mercury held in April of 1959, one of the very first questions from the media asked of our pioneering “star sailors” was how many of the men smoked and could they handle going without lighting up during their space missions? Three of the astronauts acknowledged that they smoked on a regular basis, but all of them said they could go without smoking during those times. Considering just how cramped the Mercury spacecraft interior was, there would not have been much choice for them in any case.
Space Fatigue
Our scene changes back into space. With that same ethereal music, this time we head towards the winged spaceship parked some distance from the rotating space station.
Inside the spacecraft, we see four men enclosed in white spacesuits preparing the ship for its historic journey. One might think the conversations between these men working on a sophisticated vessel meant to land humans on the Moon would be quite technical in nature, perhaps even introspective and philosophical. However, as we listen in, we discover that their exchanges could be happening just about anywhere, such as a loading dock…
“Somehow or another, I kind of hate to see this job get finished,” says one man with a distinctly Brooklyn accent. “It’s like my cousin Seymour. He’s a plastic surgeon. He built a face for an ugly dame once, which turned out to be so beautiful, he fell in love with her! So off she went with the garbage collector!”
“You afraid this beautiful ship will go off without you, Jackie?” replies another suited man who we will learn is Sergeant Imoto, a native of Japan.
“Precisely and definitely the opposite,” retorts Jackie, who is also known as Sergeant Siegle.
The third Sergeant in the room, Roy Cooper, shares his feelings on the topic of conversation.
“Well, frankly, I’m… I’m frightened of going, but… I’m more frightened of being left behind.”
“For what you scared?” asks the fourth member of this group, Pete Donkersgoed, as he emerges from an opened hatch in the deck. “We build this ship, so we fly it.”
“And so we get to the Moon,” Siegle chimes in with an expression of annoyance. “Who’s gonna guarantee we ever get back? I’m with Pete.”
Sergeant Siegle then offers more of his thoughts about the lunar mission to the group, continuing to contradict himself in the process.
“For a fat, solid year, I been eating birdseed out of this goofy sombrero with no squawk!” declares Siegle, commenting on something that will only make complete sense to the audience later in this story. “Now, let some other heroes take it from here. This little guinea pig ain’t going on no more joy-hops for the great Colonel Merritt! And if old Space Happy thinks otherwise… he can take his ship and…”
“And what, Sergeant Siegle?” demands old Space Happy – I mean Colonel Samuel T. Merritt – who has been surreptitiously listening in on their conversations from the Wheel the entire time. Jackie Siegle looks around in surprise, embarrassment, and fear at the voice coming over the radio.
Jackie makes a weak attempt to disguise his voice to his superior officer.
“Sergeant Siegle just left, sir!”
Satisfied that he has sufficiently intimidated his charges into line and keeping their thoughts about the mission to themselves, Colonel Merritt turns away from the radio panel to other business in the control room of the Wheel.
Meanwhile, Roy Cooper is busy connecting a live cable to an open panel full of electronics. Suddenly there is a bright spark followed by a series of loud electrical discharges that panic Roy into immobility.
“Roy!” cries Imoto. “Secure that cable!”
“I… I… can’t… move a finger,” Roy replies haltingly, his eyes wide and his suited body plastered flat against the nearby wall.
Imoto and Siegle rush in to grab the loose cable and prevent a potential fire or worse. Once they have the situation under control, the two sergeants turn their attention and concern to their colleague.
“Are you hurt?” asks Imoto.
“No, but I…” starts Roy. Imoto presses him further.
“What is the matter with you?”
“I don’t know,” Roy answers. “I’m… I’m… paralyzed.”
“Let’s get him back to the Wheel!” shouts Siegle. “Taxi!”
The taxi Sergeant Siegle calls for is no four-wheeled yellow cab from Brooklyn, but a small sled-like craft piloted by a single astronaut that we soon see approaching the winged spaceship from the Wheel. Roy and his fellow crewmembers are waiting at an open hatch in the side of the rocket.
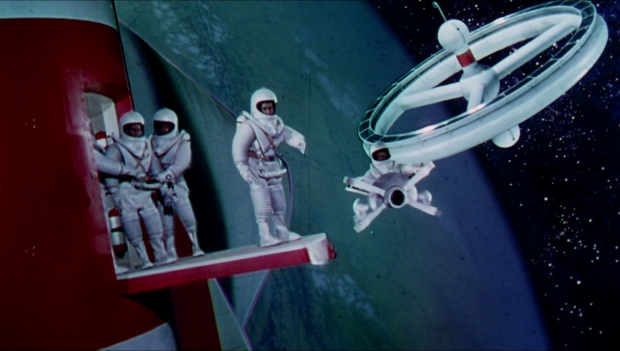
FIGURE 3. Here comes the space taxi Sergeant Siegle called for to get Roy Cooper back to the Wheel for medical attention. The other astronauts working on the spaceship will also ride back with Sergeant Cooper by being towed like water skiers. I guess that makes them space skiers, ay?
“We’ve got a sick man here!” Siegle barks to the taxi pilot over his suit radio, likely adding to Roy’s discomfort and embarrassment in the process. “Gotta get him back to the Wheel!”
They lay Roy face down across the back of the space taxi in a manner not unlike loading inanimate cargo. Then the three remaining crewmen grab on to towlines attached to the rear of the taxi.
“AII set. Shove off!”
The pilot fires a short burst from the taxi’s thrusters and the vessel moves away from the spaceship towards the space station, pulling along the three astronauts like water skiers – minus any water or skis.
COMMENT: It is a very good thing for the astronauts riding on those tow lines that the space taxi’s main stern thruster only produces short bursts of exhaust, or at least does in this case when it comes to bringing extra passengers along in this manner, because otherwise these men would be right in the line of the rocket’s fire! I also wonder why the taxi doesn’t have more room or at least a platform/gurney of some kind for carrying cargo or passengers, especially if a crewman requires medical attention? Perhaps there are other models of taxis for various functions at the space station and this was the one most immediately available due to the perceived urgency of the situation?
Back in the Wheel, in an area right outside the airlock, we see personnel dropping down their discarded spacesuits attached to a line from a wide tunnel overhead. The astronauts then follow their protective coverings using a tall black lattice structure as a ladder, where they subsequently pick up and carry off their suits to be stowed away in cage-like lockers.
Imoto and Roy Cooper are putting away their spacesuits in the same fashion. We become aware of an older, larger man in a beige uniform decorated with sergeant stripes standing next to the pair, his arms folded. He is carefully observing the activity around him with an expression that could be either one of concern or annoyance, or possibly both.
COMMENT: We the viewing audience will soon learn that this stern Irishman is called Sergeant Mahoney and he has been placed in charge of ensuring the well-being of the Moon mission crew – whether they actually like it or not.
“Are you feeling better, Roy?” Sergeant Imoto inquires of his friend and colleague.
“I’m all right, I guess,” Roy answers, not sounding entirely confident. “Yeah, I’m all right.”
Having been listening to their conversation, Mahoney doesn’t hesitate to cut in.
“Let’s have the straight of it,” Mahoney demands, without any social platitudes. “What’s wrong with the Iad? What’s the matter? You sick or something? You hurt some place?” Placing his arms akimbo, Mahoney almost gets into Roy’s face waiting for an answer.
“No, I… I just couldn’t move out there,” Roy tries to explain, “but… I’m all right.”
“You couldn’t move, you say? Why not?” Mahoney pushes even further into Roy’s personal space as the volume of his voice goes up.
“I don’t know, I… I just couldn’t.”
Just then Sergeant Siegle walks up from around one of the metal storage cages, coming to Roy’s defense.
“He’s all right, he told you. Leave him alone! It ain’t important!” declares the very person who only a short while earlier was shouting to everyone in range how Cooper was “a sick man.”
“You know the Colonel’s orders with you incubator babies,” Mahoney snaps back, just barely turning towards Siegle and then only for a moment. “Even a pimple is important. You bluebirds are my responsibility, and he’s reporting to the infirmary. Come on, lad, I’II take you meself.”
Without even asking cursory permission, Mahoney grabs Roy by the arm and starts escorting him away. Siegle makes a rude vocal noise mocking the gruff Sergeant, who pauses his steps and glowers for only a brief second before continuing with Roy to the infirmary.
Staring after the departing Cooper and Mahoney, Siegle comments to Imoto that “it’d be worth taking a trip to the Moon, just to get rid of that overgrown babysitter!”
COMMENT: Considering that the rocket crew had to radio the Wheel with an urgent need for a space taxi, which I presume included requiring a reason for that transportation, I am surprised that someone from the medical staff on the station wasn’t waiting for Roy at the airlock to escort him to the infirmary upon his arrival. Instead, there seemed to be no one in that section of the Wheel aware of what happened over on the spaceship, including the men’s “overgrown babysitter”, Sergeant Mahoney.
The scene fades to the station infirmary, where a doctor by the name of Major Kurt Elsbach is finishing his examination of Roy. In the same room with them is Colonel Sam Merritt and another doctor named Sergeant Andre Fodor, a native of Austria.
“What is it, sir?” Roy asks nervously. “I… I’m all right, aren’t I?”
The doctor assures Roy that he is fine, only adding that what happened to him on the spaceship was “nothing more serious than a momentary lapse of nerve function.”
Still anxious despite the doctor’s response, Roy walks up to the Colonel.
“You believe him, sir?” Roy asks the Colonel as to what the doctor had just said. “I mean, this couldn’t make any difference. It’s been a whole year, sir, and after all this time, I… I’d hate to wash out.”
The Colonel answers in a somewhat deflective manner.
“Well, I’d hate to lose you, Cooper.”
Roy thanks the Colonel and walks towards the hatch. Fodor opens the door for him, where he adds a friendly smile and a pat on the shoulder as reassurance to the man who exits.
Colonel Merritt walks up to the first doctor.
“Let’s have it, Kurt,” Merritt demands. “What’s really the matter with that boy?”
Before Doctor Kurt can comply, Fodor cuts in with his own unrequested response.
“Oh, Cooper’s in fine condition, sir,” Fodor interjects, clearly trying to defend his colleague and friend. “Why, you gave him a complete physical examination only three days ago, Major. A perfect score, remember? You don’t have to worry about that boy, sir, I assure you.”
“He was paralyzed out there, Sergeant,” Merritt responds matter-of-factly. “He couldn’t move. That’s something to worry about up here.”
Merritt quickly turns back to Doctor Elsbach to ask what is really going on with Roy.
“Somatic dysphasia,” the doctor replies, then explains his technical term: “Self-induced inability of the nerves to transmit brain messages. In your language, space fatigue.”
COMMENT 1: Now I am no Doctor of Medicine, but it would seem the Conquest writers created a new scientific term for what they are calling space fatigue. This makes sense, since these men live continuously in space in an artificial environment of a retro-future era unlike the one most Earth-based humans have known.
As an interesting side note: In the 1956 film Forbidden Planet, which takes place in parts aboard the confines of an even smaller star vessel that has spent over one year traveling sixteen light years from Earth to the exoworld Altair 4, they referred to a similar situation with their own new term: Space blues, with one remedy for it being shock therapy! FYI: The term and the subsequent discussion on these space blues did not make it into the final cut of Forbidden Planet; sadly, its mention was only in the 1954 draft script.
COMMENT 2: Both science fiction films considered the ideal crew for deep space missions to be structured like a military expedition with combined services, complete with the expected military levels of discipline and order. In addition, in specific regards to Forbidden Planet, the starship crew of the C-57D was described at one point by its captain as “nineteen competitively selected super-perfect physical specimens with an average age of 24.6 years.”
“Self-induced?” queries Merritt.
“Well, not consciously, of course,” Elsbach begins, with Fodor noticeably walking off to another part of the room where he busies himself while still paying attention to their conversation. Judging by his body language, one can tell that Fodor knows where things are probably going for Sergeant Cooper.
“Each mind has its own limits of endurance, at which point it rebels. The result can be anything: Simple hives, hallucination, headache, loss of speech, paralysis, total insanity, anything.”
Elsbach shuts off an examination light, then continues his lecture.
“ALL of us up here suffer from the same disease to some degree. It is to be expected. Man has never before lived in space. Fortunately, most of the cases are so minor they present no problem.”
“But Cooper?” Merritt asks.
“Cooper will be perfectly normal… as soon as you return him to Earth.”
“That bad?”
“What he experienced was simply a warning,” Elsbach explains. “If it happens again, it could be permanent.”
“I see,” says Merritt, mulling over what he has just learned. “How about the others?”
“Andre, Imoto, excellent,” begins the doctor, who looks over at Fodor, who looks back and then walks out of view.
“As for Siegle, Sanella, and Donkersgoed… every day with them, it is a new set of horrible afflictions.” This comment makes Merritt laugh knowingly. “Some of them completely unknown to medical science.”
Doctor Elsbach continues with his analysis.
“Furthermore, they all seem to have an absolute loathing for the Wheel, its commanding officer, its doctor, a certain Sergeant Mahoney, and the Space Corps in general. Everything, with the possible exception of good food…” Kurt pauses slightly to place a smoking pipe in his mouth “…and women.”
“In other words, they’re normal,” Merritt concludes, amused. “Thank you very much, Major.”
COMMENT 1: I just had to look up the last name of Donkersgoed, one of the lunar mission crewmen mentioned by Major Kurt Elsbach and whom we met briefly back on the spaceship. It is a real surname, but according to one Internet site it is only the “1,058,336th Most Common surname in the World,” which is not very surprising. Donkersgoed appears to originate from The Netherlands, which ties in with Conquest making an effort to show how international in composition this retro-futuristic space program is. In this paragraph alone I have already given essentially more attention to this poor fellow than he will receive in the entire released film!
COMMENT 2: For the record, Elsbach is a Dutch name. It is also the name of several tributary rivers in Germany. Siegle is a variation on Siegel as well as Segal and Segel. It is a German and Ashkenazi Jewish surname going back to at least Eleventh Century CE Bavaria. Siegle and its variants denoted people who made wax seals for official documents or sealed them.
COMMENT 3: The actor who played Jackie Siegle, Phil Foster (1913-1985), was born and raised in Brooklyn (his birth name was Fivel Feldman). Before being hired by George Pal for his role in Conquest, Foster had made several popular short comedy films as “Brooklyn’s Ambassador to the World” for Universal-International. At least he was the genuine article.
Having left the infirmary, Colonel Merritt is next seen walking through a corridor towards yet another hatch, this one with a crewman in beige working on a small black control panel next to it. Suddenly Merritt pauses to lean first against the panel and then the door, gripping at the hatch wheel (also known as a door locking wheel, speed wheel, and a dog when referring to one on a submarine) in some kind of apparent distress.
“What’s the matter, sir, are you ill?” asks the concerned crewman, who prepares to steady the Colonel in case he starts to fall.
“No,” replies Merritt, standing up fully. “No, I’m all right.”
The crewman attempts to open the hatch for Merritt, but the Colonel quickly grabs the wheel and does it himself, forcing a smile on his face and telling the man to “carry on” before leaving the corridor and shutting the hatch behind him.
Alone in his office/quarters, Merritt shakily goes over to a wall and slides open the white panel there to reveal a half-hidden compartment behind it. From there he removes a small transparent container holding a white powdery substance and places it on top of the cabinet. Merritt scoops into this substance just once and deposits the powder into a cup. He then retrieves a translucent bottle containing a liquid and pours it into the same cup. Drinking the contents, Merritt takes in a deep breath and seems to feel better.
The scene switches to someone holding a black-and-white photograph of a woman in what one calls a “cheesecake” pose and signed “Always Yours Jackie Baby”. As one might suspect, the owner of this photo is Sergeant Siegle, who is looking at the image in the barracks section of the Wheel with some of the other crewman. Generic “swing” music plays in the background. Various pinups of beautiful women adorn the far walls of their living quarters.
“My Rosie!” Siegle exclaims to the men around him. “Thank heaven science ain’t found no way to put you up in capsules.”
Sitting on a bunk next to a worried-looking Roy Cooper, Siegle lifts up the photo to Italian crewman Pedro Sanella lying in the bunk above him and waves the image in the man’s face.
“The future Mrs. Siegle, Pedro! How’d you like to paddle that around in your gondola?”
Pedro takes a brief look at Rosie and declares “for a wife, too skinny.”
“Too skinny?” exclaims Siegle. “That’s beautiful skin, boy!”
Mercifully, the camera diverts our attention to Roy, who gets up and walks over to Fodor.
“Andre, what did they say?” Roy asks his friend. “I’m not out, am I?”
“Out of what, Roy?” Fodor inquires in return while wiping his right ear with a small towel after having taken a shower.
“The Spaceship. It must have a crew.”
“Now, look, Roy,” responds Fodor, taking on a reassuring tone, “the Colonel hasn’t told us definitely we are the crew.”
“We don’t have to be told,” Roy shoots back. “We are, you know we are.”
Roy then goes into an exposition not only to support himself but to explain the background of the mission crew to the audience. The men around Roy stop what they are doing to listen to his words, which become more frantic sounding with each new sentence.
“Every man on the Wheel won his place after six months of the stiffest competition in the world,” Roy begins. “Each one of us were handpicked from the winners for this special duty. Who else is being conditioned as we are? Special food, special exercise. Tests, lectures! Watched every second! Never any leave!”
Tightly gripping a metal bunker support pole, Roy becomes aware of how he is sounding and pauses, looking around at no one in particular, but now speaking to everyone present.
“You fellows know how… how tough it’s been. Now, just because I had a… bad couple of minutes out there….”
Feeling distraught and ashamed, Roy turns away from the group. Fodor silently gestures to Pedro Sanella to say something to cheer up Roy, or at least distract him.
“That’s funny, I didn’t think to have a bad couple of minutes myself!” Pedro jokes. The men laugh at the comment in support.
Sergeant Siegle automatically gives his opinions on the matter.
“I don’t think we’re going no place,” he claims. “AII right, so we built a spaceship. That doesn’t mean we have to fly it.” This is in direct contrast to Donkersgoed’s earlier comment of “we build this ship, so we fly it.”
Then Siegle comes up with both an alleged insight about their mission and his personal future plans all at once.
“Hey, maybe we’re guinea pigs!’ he declares. “Maybe they wanna find out how much of them cosmic rays a human carcass can absorb before we light up like Christmas trees. And at double pay, I can learn to like cosmic rays! With all that loot, boy, I’m gonna open a TV shop, settle down, marry my Rosie, and raise a houseful of kids. So if I glow a little in the dark, she could find me better!”
“If you get that charged with cosmic rays,” Imoto responds with some bemusement, “you’d better not plan on too large a family.”
“That’s a lot of borscht!” is Siegle’s “scientific” answer to Imoto. “One of them cats in the lab just had a litter of seven kittens, and she’s been up here longer than we have. And anything a cat can do, me and Rosie can do too.”
COMMENT: The gestation (pregnancy) period for your average domestic feline is about 65 days, or two months. As these crewmen have been on the Wheel for approximately one year now, not counting any other time they may have served in Earth orbit, this likely means there is a specialized in situ breeding program going on in the station – unless the cat was artificially inseminated via frozen sperm stored onboard earlier. I also wonder what other animals they may have on the Wheel for testing biological processes and responses to long-term exposures to the space environment?
Even in the pre-Space Age era when Conquest was released on the big screen, various fauna and flora had already become the first space explorers aboard suborbital rocket flights. The United States often preferred monkeys (and later evolving to apes such as chimpanzees) as their test subjects, while the Soviets favored dogs. Animal astronaut and cosmonaut missions would only increase as the Space Age turned into a Space Race. A few cats were later a part of the Animal Space Corps per se, but they were not a preference by the major space agencies.
Space Smorgasbord
Just then a two-tone chime sounds. The men excitedly rush to the barracks hatch.
“Last call for dining car!” cheers Imoto.
As expected, Siegle is even more basic and to the point.
“Food!” Jackie cries, flinging up his arms with joy.
Siegle, Imoto, Donkersgoed, and Sanella line up in pairs on either side of the hatch. They start a countdown in unison, getting as far as the number two when the heavy metal door swings open and Sergeant Mahoney appears in the entrance. He looks at the crewmen standing rigidly at attention.
“Shall we go, gentlemen?” Mahoney invites, laced with a bit of bemusement in his tone.
“Yes, Mother!” the four men respond as one in a sing-song voice and rush through the rectangular opening. Siegle goes through last, stopping just long enough to give Mahoney a playful and also mocking pinch on his cheek before heading on to the mess hall.
Roy Cooper is still in the barracks, leaning against a locker after his outburst. Unsurprisingly, Andre Fodor is there with Roy to offer some comforting gestures of support, which oddly include ruffling the hair on Roy’s head from the back. The two go to join the rest of their comrades.
In the long dining area of the Wheel, we see dozens of beige-clad men at the tables already well engaged in simultaneously consuming their dinners and enthusiastic multiple conversations. There is one table, however, that is conspicuously empty: Only a server is present, and he is in the process of completing the settings of plates, cloth napkins, white drinking containers that resemble formula bottles for infants, and one large platter covered by a napkin. This special table is meant for its next occupants, who just happen to be our lunar mission crewmen.
As the select group in blue march into the dining hall in single file, with Imoto in the lead, the rest of the crew stop eating and sit at attention. The men come to stand in front of their place settings, with Sergeant Mahoney strolling to his position at the head of the table.
“Be seated, gentlemen,” orders Mahoney, not unkindly.
The six crewmen sit down without hesitation. Just then, the rest of the members of the mess hall simultaneously stand up and burst into a “serenade” that leaves the special astronauts looking away, feeling conspicuous and embarrassed…
“Mahoney has six little lambs. /He has to watch their diet. /They helped the Colonel build his ship. /And now they have to fly it!”
The men in beige conduct a short-gestured synchronized applause, then laugh uproariously at their idea of humor before returning to their meals.
“Peasants!” Siegle shouts back at them.
“Dig in, fellas,” Mahoney says with a smile and an inviting gesture with his left hand towards the contents on the table.
At last, we come to understand what Sergeant Siegle was complaining about back on the spaceship when said he had spent the last year “eating birdseed out of this goofy sombrero with no squawk”: Underneath that one napkin set before our men turns out to be a multilevel Lazy Susan spinnable platform painted primary blue with labeled compartments for many kinds of food and drink… all in pill form.
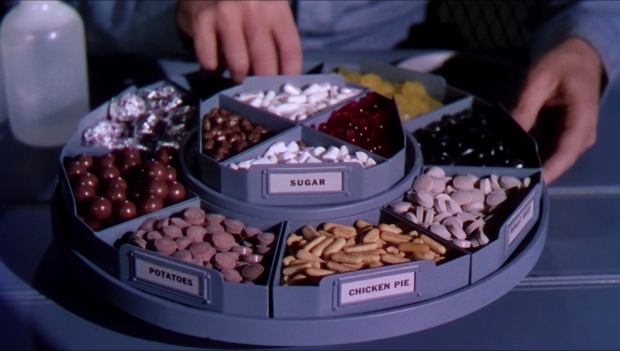
FIGURE 4. One of the more remembered scenes in Conquest of Space is the diet of our prospective astronauts: Not so much the food itself as the form it is in. Meals in a pill were one of the standard tropes of an imagined future for a technologically progressive humanity.
The camera closes in on the pill platter. Donkersgoed turns the platter a bit and selects several food pills from the tray, observing one of them grasped in his fingers with a lack of enthusiasm.
“Space smorgasbord,” Donkersgoed declares ironically to the rest of the table, before opening his mouth and popping in his dinner with a dramatic gesture.
Imoto, always the optimist, tries his portion and proclaims it is “pretty good today. Corned beef, I think.”
Holding up his quite compact meal, Imoto continues with his praise of this futuristic diet.
“Imagine… all the nourishment you need: No mess, no bother, and no waste.”
“I think I still prefer to eat the hard way,” Pedro Sanella concludes in turn. “Hey, Jackie! Pass me a cup of coffee.”
Sergeant Siegle picks out a coffee pill from its tray and gives it a short fling down to Sanella, who catches it.
Sanella looks back at Siegle and expectantly asks for “cream and sugar” with his coffee. Siegle obliges with the tossing of two more pills to his comrade.
Just then a server happens to pass by the lunar mission crewman’s table carrying a whole roast turkey for the other men in the mess hall. Siegle notices the cooked fowl first with his nose, then makes an exaggerated gesture with his head in its direction.
Mahoney looks at Siegle, disapproving of his behavior.
“Okay,” Siegle responds in a surrendered tone. “So I volunteered. So I’II eat.” Siegle goes back to his pills and then looks at Mahoney, who is also sharing in the rest of the crew’s diet.
“What are you eating it for?” Siegle asks the Irish sergeant, as if somehow this was the first time the man would have consumed the pills with them as well.
“Colonel Merritt eats it,” is Mahoney’s succinct reply.
“That’s a reason?!” Siegle says in astonishment.
“For thirty years, me and the Colonel have been banging around together,” Mahoney begins his answer. “Korea, Africa, China, now space. If he intends to shove off to anywhere else, I ain’t giving him any excuse to leave me behind because I ain’t eating the proper diet.”
Finished with his explanation, Mahoney looks down where the pill platter is presently residing on the table and asks Sanella for “some more of that corned beef, if you please.”
COMMENT: The staple and trope of many a science fiction story of the era was the consumption of food in pill form. In the Twentieth Century, as human civilization advanced and a desire for a more orderly world increased, it was considered and hoped that our most basic and strongest biological need, eating, would be rendered far less messy and uncouth with the turning of our meals into neat, compact forms. Condensing the food supplies for a deep space mission lasting for months or years would also be desirable in terms of not taking up precious volume aboard a confining vessel.
Would it actually work? Would we be able to survive on pills alone? Would we want to even if we could? This popular level article discusses this very subject, pointing out such important matters as our bodies requiring calories as much as we need nutrients:
https://www.popularmechanics.com/science/health/a8787/why-dont-we-have-food-replacement-pills-15248871/
The site Atomic Rockets did its usual fine job of discussing food pills and their issues. They even have two different views of the pill carousel from Conquest to accompany their segment!
http://www.projectrho.com/public_html/rocket/celss.php#id– HYPERLINK
Even if we can eventually succeed in making all our meals compact and clean, we may balk at them in the long run for the same reasons as some of the crewmen in Conquest grumbled about their pill dinners – made even more inexplicably torturous for them by being surrounded by the rest of the space station staff who are dining on full meals of meat and vegetables. Yet earlier, we watched Colonel Merritt chew out a crewman for merely mentioning a desire for ice cream!
We may have to change our very selves in order to truly adapt, survive, and thrive in space indefinitely before we can accept our nourishment in any other manner than the way we consume food now. Perhaps if we go that route, we may discover methods that do not even require our need to be organically fueled multiple times per day, if at all.
Mahoney turns his attention to Roy Cooper, who is lost in thought.
“You feeling better, son?” he asks Roy.
“Oh… fine,” Cooper responds without much conviction. He then has a question of his own for the sergeant.
“Mahoney,” Cooper begins. “You know the Colonel a Iot better than the rest of us. You don’t think he’ll wash me up?”
“Stop worrying,” Siegle interjects. “If he’s going off on an excursion, who’s he gonna take? He’ll have to ask for volunteers again. Us? That son of his?”
Siegle continues with a rumor he has heard elsewhere.
“I happen to know by the grapevine that the Captain has already put in for a transfer,” reports Siegle.
“So that leaves you,” Siegle says to Cooper. “So I hope you and the Colonel will be very happy together!”
Siegle suddenly gets distracted by declaring to all around that the current pill in his mouth “ain’t kosher corned beef!”
Mahoney, however, is much more interested in what Siegle had just said about Barney Merritt.
“So, the Captain put in for a transfer, did he?” Mahoney asks to confirm this news.
“Well, good riddance, I say!”
“He’s a fine officer,” Imoto replies in Barney’s defense.
“He doesn’t measure up to his father’s belt buckle!” Mahoney shoots back. “You know, I was with the Colonel the night he got the word the kid was born. We were in Indochina. We did a little bit of celebrating.”
Smiling at the memories of that night, Mahoney continues.
“I remember the Colonel – captain he was then – pointing up to the sky and said: ‘You see that Moon? That’s his birthday present. Someday I’m gonna give it to him.’
“A balloon on a string would mean as much to the ingrate!” Mahoney growls, clearly disgusted with Barney’s disloyal behavior towards his own father. “Putting in for a transfer!”
Mahoney’s rant is interrupted by a server wearing a chef’s hat carrying a large tray with various covered dishes to their table. The server places them before Roy Cooper.
Cooper looks up at the server with surprise and concern.
“This is a mistake,” he says.
“No mistake,” replies the server. “Compliments of Colonel Merritt.”
The server removes the covers from the dishes, revealing a full and delectable-looking meal identical to what the rest of the Wheel staff is consuming. Roy’s crewmates stare at the plates of unconcentrated food and hungrily announce out loud what they see before them.
“Steak!”
“With mushrooms, yet.”
“Asparagus!”
“Go ahead, Roy,” Fodor gestures. “Dig in.”
Cooper looks around anxiously at the rest of the men.
“Looks… looks delicious, doesn’t it?” he says, trying to be enthusiastic about this “gift.”
“Go on, cut it,” demands Siegle. “Cut that steak!”
Cooper picks up a knife and fork. With a bit of hesitation, Roy starts to slice into his steak.
Siegle, staring at Roy’s full plate, can barely contain his desire for real food.
“Man, that juice.”
With a piece of the steak impaled on his fork, Cooper starts to move it towards his mouth. Suddenly the man stops, knowing what all of this really means for him, and angrily slams his cutlery down on the tray before running out of the mess hall in frustration and shame. Fodor immediately leaps up from the table and runs after his friend out through the hatch.
The table remains silent for a moment. Then Imoto speaks to Siegle.
“You were saying, Sergeant Brooklyn?”
“I was saying, Sergeant Imoto,” Siegle replies. “If it wasn’t for a certain fatheaded stool pigeon just waiting for me to do it…” Siegle stares meaningfully at Cooper’s meal on his left. “Man, I’d be lapping up that steak juice…”
COMMENT: For those of you concerned as to the fate of our poor Sergeant Roy Cooper after being humiliated in front of his colleagues and subsequently washing out of the Space Corps manned lunar program, you will be pleased to know that Roy later changed his name to Bill Owens, joined the United States Navy (USN) where he reached the rank of Captain, and even designed a nuclear-powered submarine called Proteus. Originally designated U-91035 and meant for the study of the spawning habits of deep sea fish, the Proteus and its designer were recruited as part of the CMDF, which stands for Combined Miniature Deterrent Forces. Captain Owens and a small team of mostly medical experts would go on a critical top secret mission where no one had gone before as part of the West’s multitudinous Cold War efforts.
Sergeant Siegle’s declaration of “love” for Roy’s cattle meat is abruptly and violently interrupted by what sounds like a very loud explosion. The mess hall tilts to one side, sending its occupants falling and sliding down the deck and across the tables, knocking over trays and plates of food.
COMMENT: One could say that the mess hall became a real mess… hall.
The view changes to space, where the Wheel is being rocked by a collection of bright flashes along one side.
“Meteor, sir!” a crewman shouts to Colonel Merritt in the control room. Clinging to the edge of a wall panel, Merritt barks orders to his crew to evacuate and seal off certain sections and then fire the Wheel’s exterior jets (rocket thrusters) to stabilize the careening station.
We watch several men in spacesuits sealing off small holes in the station’s hull made by the impacting meteoroids with round metal plates held in place by air pressure. This emergency team is simultaneously busy putting out several mechanical fires with extinguishers.
Slowly the Wheel stops rocking and we return to a relative sense of safety and peace, signified by that same ethereal music as heard before.
Back in the mess hall, most of the men are attempting to pick each other up from the deck, with some staggering about groaning in pain and discomfort. Sergeant Siegle, who has somehow acquired several large pieces of turkey meat, emerges with a very different reaction to this event than his companions.
“Boy, oh, boy, what a fortune I could make with this thing at Coney Island!” Siegle shouts to several men in front of him who are otherwise occupied. “Boy, I’m telling you!”
Mahoney stares at Siegle: The man from Brooklyn not only has a big chunk of turkey in his hands, but also a smaller piece of the quite deceased and roasted bird dangling from the front pocket of his uniform. Siegle returns the look to Mahoney and defiantly bites off a mouthful of meat before walking away with his prize.
COMMENT: Rather surprisingly, the man from Brooklyn is apparently never reprimanded nor otherwise disciplined for this defiant act. We also never learn if the sudden introduction of solid food after a year of eating only pills would interfere with his digestive system. That Colonel Merritt saw no issue with having Roy Cooper served similar food after his own year of consuming pills may mean that such concerns are unwarranted, or they found a way to assist the human body in safely switching back.
Floating in a Most Peculiar Way
Some time later we are shown the smaller viewscreen witnessed early on aboard the Wheel in Conquest. Once again, we see on this very screen the white contrail of a rocketship slowly growing as the craft heads towards the space station. Captain Barney Merritt is observing this visitor carefully.
“Landing crew ready to make fast,” the Captain orders. “AII stations manned. Let’s go.”
With dramatic rising music, the winged ship arcs upward and then stops at an equidistant point between the big winged spaceship and the Wheel. Seemingly seconds later, a large metal hatch opens in the side of the red striped rocketship.
A small group of spacesuited men, each carrying a single duffle bag cradled in their left arms, emerge from the opening to walk slowly and a bit clumsily across the hatch, now serving as a sort of gangplank extending into space. The reason for their ungainly gaits is due to the magnetic boots in their suits holding them to the metal door.
The first man in line reaches the end of the hatch, where he is given a push on the back by the man behind him, sending him flying towards the Wheel. The scene is repeated three more times; with the same ethereal music, the first four men in this party drift in the direction of the space station, apparently without any obvious means for guidance correction or stopping when they arrive at the giant wheel.
There are just two men left at the delivery ship. One of them is staring rather apprehensively at the other men who are receding into the distance. His companion notices his fellow’s concern.
“It’s okay, Mr. Fenton. Don’t be afraid,” the astronaut says, adding a series of reassuring pats on his covered shoulder. “You’ll just float over.”
The man gives Mr. Fenton the requisite push, but not with his hand on his companion’s back like the others. Instead, he gives Fenton a shove with his foot on the man’s hindquarters! This sends poor Fenton tumbling end over end towards the Wheel, undoubtedly increasing his anxiety and perhaps even making him feel spacesick in the process.
COMMENT: Was this scene meant to be a deliberate joke for the audience? It may look amusing in a base way, until you realize the guy who got the literal kick in the pants was already scared being out in open space and has now probably graduated to outright terrified, flipping over himself repeatedly with no personal means of control. There is also the matter of treating a guest with respect, unless I am missing some aspect of traditional/contemporary military culture behavior?
This Fenton is clearly not used to conducting an EVA (extravehicular activity) – yet they shove him in the direction of the space station and just assume he will successfully arrive there. What equipment and protocols did the Wheel have to keep those visiting the station in this manner from either crashing into the station or missing their destination entirely, going off into the void with perhaps only a few hours of breathable air in their spacesuit between rescue and doom.
Perhaps the station crew thought they could just send one of their space taxis after Fenton in case he did miss the station, but then why not have this obvious VIP (Very Important Person) picked up by the taxi at the delivery rocket in the first place? It is just amazing, and not in a good way, how they (the crew and the script writers by default) thought it would not be a problem to have people just drift over from one vessel to another in the vacuum of space without so much as a jetpack or tether line – especially if they are new or otherwise unaccustomed to space travel.
Why are there no apparent means for a spaceship to just physically dock with the Wheel? This would avoid a multitude of logistics issues, including the ones just mentioned regarding getting passengers safely to the station. Were there some technical or budgetary problems with the special effects that removed the possibility for such scenes? Or, keeping in context with the reality of the film’s world, was there some sort of safety protocol in place that did not allow a rocket to be near the space station, thus the reason they had to park a certain distance?
The makers of Conquest cannot be completely excused about this situation by having dwelled in the era before human spaceflight: Destination Moon tackled the pitfalls of EVAs in 1950, showing what could happen to astronauts who found themselves in space with no way to control their trajectories or even lacking something solid to push against. Again, for a film that was meant to depict space travel as realistically as possible for its time, this EVA scene is just one of multiple failures of logic that hamper the diligent viewer from taking Conquest as seriously as it wants.
“It’s Just Across the Solar System!”
Thankfully for all concerned, although we are never shown precisely how it was accomplished, Mr. Fenton and the rest of the party from the delivery rocket do arrive alive and intact at the Wheel. When we see them next, they are climbing down a ladder inside the station, their spacesuits already stowed away. Fenton is the only member of the group wearing a dark suit and tie.
Captain Barney Merritt is there to greet them at the base of this ladder.
“Hiya, Johnnie!” greets Barney to the man who is the pilot of the delivery rocket. “Got you back on the milk run, I see.”
“Yeah,” Johnnie acknowledges. “Somebody’s got to service this box kite.”
Johnnie is carrying a brown business-style briefcase, which he hands to its owner.
“Brought you a visitor.” Johnnie declares to Barney. “Doctor…”
Barney finishes the introduction.
“Dr. Fenton. Nice to see you,” Barney says, shaking the man’s hand. “The Colonel’s expecting you, sir. If you’d like to freshen up first…”
“Thanks, later,” cuts in Fenton, the owner of said briefcase. “If it’s possible, I’d better see the Colonel at once.”
“Of course.”
Barney turns to a man standing behind him.
“Take charge of the new men, Lieutenant,” he orders. The officer guides away the rest of the group, with Johnnie following along. Barney escorts Dr. Fenton in a different direction, towards his father. The new arrival looks around the space station interior in wonder as he is led off.
We find Colonel Samuel Merritt in his office, where he is leaning against a wall containing a viewscreen. He is consuming yet another portion of that unnamed medicine we saw him drink earlier. The room buzzer suddenly sounds. Sam quickly puts away the medicine and its containers in their small cabinet.
“Come in,” invites Sam. The hatch swings open and Barney and Fenton walk into the room.
“George!” Sam exclaims with delight. “Thank heaven they sent you. How are you, boy?”
Fenton puts his briefcase on a nearby table and shakes Sam’s outstretched hand.
“A bit rocky, but all right otherwise,” Fenton reports. “And you, Sam?”
“Oh, fit as a fiddle,” replies Sam. “It’s against regulations to feel any other way on the Wheel. My own orders,” Sam adds with a chuckle, poking his index finger on his own chest. “Come on, sit down.”
“Thanks, I will.” Fenton walks over to a line of chairs against the office wall and chooses one to sit in. Sam takes a corner of his own desk for a perch.
“I didn’t know until a half hour ago that they were sending you up,” explains Sam. The Colonel points to Barney standing next to them.
“You remember my son, Barney, don’t you?” Sam asks Fenton while looking at Barney. “Dr. Fenton helped to plan every detail of this Wheel, Barney. They thought we were insane then, didn’t they, George?”
“Well, frankly, I thought so, too,” Fenton confesses. “But here it is. You put it up here.”
Sam pats Fenton on his extended knee in gratitude for the compliment.
“You know, for once, the International Authority has really sent up somebody who knows what it’s all about,” Sam declares, returning the compliment in the process. “And since you are one of the geniuses responsible for that… monstrosity,” Sam adds, rocking his thumb at the large Spaceship blueprint hanging on the wall behind him. “Would you mind telling me what it is?”
Not waiting for an answer from his friend, Sam gets up and walks over to the Spaceship diagram.
“We assembled this thing piece by piece as it came up, according to your brilliant specifications,” Sam begins. “It’s a masterpiece of technology and electronics. But it doesn’t make one particle of sense.”
A bit confused, Fenton gets up and walks over to Sam by the diagram.
“In just what way do you mean?” Fenton inquires.
“Well, here,” Sam answers, making a sweeping gesture across the diagram.
“Take these wings, for instance. What in blazes are wings doing on this ship? There’s no atmosphere on the Moon!
“The Moon?” Fenton interjects with surprise in his voice. Sam isn’t finished yet, though.
“And this booster,” Sam adds, tapping on the diagram where the booster is drawn. “All that power. What are we gonna do, go up to the Moon, or going through it? Or maybe we’re supposed to tow it back with us so those bright boys from every nation on Earth can have a better look at it. Is that the idea? Working for one government was bad enough, but now we’ve got all of them on our backs.”
Having made his little speech, Sam turns back to look at the Spaceship diagram again. As he does, Fenton takes the brief trip to the desk where his briefcase resides and zips open the satchel.
“I think you’d better read your orders,” Fenton offers while rifling through his papers from the briefcase. “General.”
Sam turns to Fenton in surprise.
“General?” he asks.
“Your promotion is among these dispatches,” Fenton replies.
Barney rushes up to his father and shakes his hand.
“Congratulations, sir. A general!” Barney exclaims, beaming with pride at his father.
“Your orders, Sam.” Fenton hands Sam a large manila envelope. Sam eagerly rips open the envelope to remove the letter with his orders.
Dr. Fenton turns to Barney with his own papers.
“And for you, Captain, your transfer,” he says. “You’ll be attached to Muroc, I believe, as you requested.”
The smile on Barney’s face fades. Barney looks at his father a bit embarrassed, as he had not told Sam he was transferring off the Wheel despite his father’s earlier orders against it. Sam is again surprised.
“You can return with me, if you like,” Fenton offers to Barney. The Captain gives Fenton a very curt nod of acknowledgement.
COMMENT: I found it odd that they would still call the California military and flight test base Muroc when it had been renamed Edwards Air Force Base in 1949, six years before Conquest of Space was released.
The audience gets to see what Sam’s orders are, which state as follows:
Supreme International Space Authority
To: General Samuel T. Merritt.
REVISED ORDERS:
1. Test flight to Moon is hereby cancelled.
2. Your destination is the planet Mars.
Order signed by Aram C. Cronin, Commander in Chief.
With a flair of dramatic music, stage lighting focuses on the numbered items of revised orders in the signed letter.
Sam looks up at Fenton and simply says: “Mars?” Barney repeats the same inquiry to his father.
Sam looks down at his orders again in a state of disbelief, then looks back at his friend.
“George, this is fantastic,” Sam states with a distinct lack of enthusiasm.
“No more so than going to the Moon,” George replies, not quite fully reading the room.
Sam walks over to his desk and around it, away from George and Barney. On the desk we can now plainly see a small scale replica of the Spaceship floating near the Wheel.
COMMENT: I bet this very model was used for filming at least some of the exterior Spaceship scenes throughout Conquest.
Still in shock, Sam begins to express his real thoughts and concerns about this news.
“Mars isn’t the Moon,” he begins. “There’s a slight difference of …several million miles. Not one word. No warning.”
In frustration, Sam slams down some of the papers in his hand, but these papers glide off the table and out of our sight.
“Just… take off and leave! It’s just across the solar system!” Sam shouts, in full irony mode.
Sam then turns to Fenton.
“I tell you we’re not ready, George,” Sam declares. “Why wasn’t I consulted?” Sam quickly jabs at his own chest.
Fenton walks up to Sam.
“We only reached the decision yesterday. There was some discussion about your age, and, well, General Cronin convinced the Authority…”
“And who convinced Cronin? You?!” Sam cuts in.
“A long time ago, the Supreme Council issued an order that the final objective of this project was to be the planet Mars,” Fenton explains to Sam. “You were present at all those discussions. The Moon was never anything more than just a test hop. Now the orders are to eliminate the test and proceed with the original plan. Time makes it imperative.”
Fenton throws in a more emotional appeal to the newly minted General.
“There’s only one man who can take that ship to another planet and bring it back: The man who built the Wheel.”
Sam is still unmoved by Fenton’s play to his ego.
“To gamble the lives of a crew of men… on as senseless a mission as this… is callous. It’s stupid!”
Fenton gets closer to Sam and becomes firmer in his mannerisms and speech.
“Stupid or callous it may seem to be at this time. It is not senseless! Man’s very survival on Earth depends upon the success of this or some future search for a new source of raw materials.”
Sam turns away from one of the chief designers of the station they occupy and looks at his orders again.
“General Samuel T. Merritt,” Sam declares out loud, reading his name and new position carefully. “A very imposing title… for a tombstone.”
“The orders are naturally contingent to your acceptance, General,” Fenton says, avoiding Sam’s histrionics. “You can refuse.”
“When do we leave?” is Sam’s answer, becoming more outwardly calmer while half-looking at Fenton. Fenton turns and walks away from Sam back to his briefcase, satisfied with the General’s response.
Barney quickly moves up to his father, concerned.
“You’re going?”
Sam turns to face his son.
“I’ve never refused to obey an order, Captain.”
“I never have either, sir,” Barney responds. “Until now.”
Barney meaningfully tears up his orders to return to Earth and his wife in front of his father, then turns to Fenton with a smile.
“When do we leave?”
Dr. George Fenton gives Barney Merritt a brief look, pleased that his intentions for journeying to the Wheel have been a success.
“I’m Sure Ignorant, Sir!”
The scene fades to a conference room where General Sam Merritt assembles a meeting of the remaining mission members, along with Dr. Fenton and Sergeant Mahoney. The group forms a rough semicircle around the General, who is at the front of the room wearing his full blue uniform, including his flight cap. Fenton and Barney are standing next to Sam.
On the wall behind General Merritt are several large paper charts drawn in red ink on white paper. One of them depicts the plan for the lunar voyage the astronauts were originally going to take.
“Our previous orders were to leave the Wheel, proceed to the Moon, orbit for observation, make a landing, and return to base.” Sam uses a long silver pointer to emphasize in turn each objective he mentions on the chart.
“These orders have now been canceled,” Sam declares while turning to the group, folding his arms behind his back. “We’re not going to the Moon.”
The men look at each other with surprise and confusion, except for Siegle, who is smiling at this sudden news.
“Mr. Fenton has brought us a new directive.” Sam unpins and tears away the sheet containing the Moon mission plans, revealing a new diagram beneath it, one detailing a different objective: The planet Mars.
COMMENT: So were those Mars mission plans always up on that wall behind the lunar objectives diagram? Or did Sam arrange them in this manner just for this meeting for dramatic effect? If the Mars flight diagram was always present, this gives General Merritt, among others, even less reason to be surprised about the change of mission plans, be they logical or otherwise.
“Our time of departure will be 11:36 tomorrow morning,” the General states. “Our destination, the planet Mars.” All the men look at Sam with serious faces. The General continues his explanation, using the new chart to highlight the details of this revised space mission.
“Now, that time of departure, both for the outer trip from the Wheel to Mars and for the return from Mars to the Wheel, is most important. We must arrive at the Martian orbit at the exact time when it is occupied by the planet. Obviously, the same precision of timing applies for our return.
“Now, you five men have been chosen after intensive competitive examinations. For the past year, you have been receiving special training and instruction for travel into outer space. The ship, however, can accommodate only two officers and three crewmen.”
QUESTION: Was this mission assignment number stated by General Merritt always the case? Or did they have to reduce the number of crew due to the change in destination? Otherwise, one has to ask: Were some of these crewmen always meant to be just backups to the chosen ones? Are five astronauts enough to successfully conduct the very first space expedition to Mars, which includes a surface landing?
“I have been assigned as commanding officer,” Sam explains. “AII other service on this trip will be voluntary. Since Captain Merritt has already volunteered, there are only three berths left open. Two of you, then, are going to be disappointed.”
“I can take it, sir,” declares Siegle with a sheepish smile and a sidelong glance at Mahoney, who is standing next to him.
Sam ignores Siegle’s comment and carries on, walking closely in front of the blue-uniformed men.
“Before I ask for volunteers, I should like to state my own preferences. You’re all fine men. Final selection will be made on the basis of special qualifications.”
The General stops in front of the man from Brooklyn.
“Sergeant Siegle.”
“Yes, sir?”
“I don’t think there’s a man on the Wheel with less formal education than you possess,” Sam states flatly in front of everyone.
“Yes, sir. I’m sure ignorant, sir,” agrees Siegle.
“Nor one with a better knowledge of advanced electronics,” Sam adds to his assessment of Siegle. “I should like to have you along.”
COMMENT: Real astronauts/cosmonauts/taikonauts may not have to be quite the “competitively selected super-perfect physical specimens” as described of the starship C-57D crew in Forbidden Planet, but they do have to possess a certain degree of physical, mental, and educational aptitudes working in tandem in order to be able to join a space mission. This was especially true in the early years of the Space Age. That a character like Siegle would be chosen to fly into space at all, let alone on the first manned mission to either the Moon or Mars, simply defies both logic and competency, I do not care how much he supposedly knows about “advanced electronics.”
As stated previously, there was a similar Brooklyn stereotype character in Destination Moon. However, although he did serve as a surrogate for what Hollywood considered to be the average contemporary audience member as well as the resident comic relief, that mission’s need for Joe Sweeney at least possessed some story legitimacy: The launch of the rocketship Luna was under a very tight deadline, with the added peril of having the entire mission shut down by federal government officials who did not want to see the success of a space voyage funded entirely by private industry. The original radio and radar operator unexpectedly wound up in the hospital with appendicitis, so Joe was the only other choice they had.
Sam moves away from Siegle and up to Imoto next.
“Sergeant Imoto: You’re a graduate of Osaka University, with two years of postgraduate work at the Colorado School of Mines. That knowledge is valuable.”
The General then turns towards Fodor, who is just to his right.
“Sergeant Fodor: Two years of medicine in Vienna, right?” Fodor gives Sam a quick nod in response.
COMMENT: Have they found a way to shorten the amount of time one goes to medical school in this retro-future 1980? Or was Merritt simply reiterating how long Fodor was in Austria while earning his medical degree? And if so, why just his time in Vienna? And what kind of medicine are we talking about here? A deep space, long duration crewed mission would have definite need for a real physician, so that only makes sense. We will just have to assume Fodor has the proper qualifications for this journey.
To add, I also wonder if the Vienna reference indicates that Fodor has had training as a psychologist/psychiatrist/therapist? This field was relatively new and sometimes viewed with skepticism when Conquest premiered; Vienna was often connected with Dr. Sigmund Freud (1856-1939), the founder of psychoanalysis who opened his clinical practice in the Austrian capital city in 1886. This might be one more reason why Fodor acted so concerned over Roy Cooper, seeing the man’s mental stress and responding as a therapist might. I also wonder if Dr. Elsbach was a psychoanalyst as well, considering he was the man who diagnosed Cooper’s “space fatigue” for Sam Merritt.
“You’re both needed,” concludes the General.
Sam walks back towards the Mars mission diagram on the wall and turns to the group again.
“Before any of you accept, I should like to make it unmistakably clear… that the dangers of this journey are above and beyond anything that the Space Corps or your own governments have any right to ask of you. I can give you confounded little reason for this attempt to reach Mars… and no assurance at all that it will even be successful. It’s my personal conviction that no one but an idiot would volunteer. And I shall strongly suspect the sanity of anyone who does.”
COMMENT: Dear Sam, I may not be a fictional General of the Space Corps commanding a space station and soon to be a Mars mission, but I really do not consider this to be a very inspiring pep talk – especially one where you call any of these men who have already sacrificed well over one year of their lives and are undoubtedly long aware of the many inherent dangers of space travel idiots for wanting to be the first humans on the Red Planet, not to mention suspecting their sanity as well.
Could the General have been conducting a psychological ploy to scare away anyone who did not really want to journey across millions of miles of interplanetary space? Perhaps, but I am doubtful of this. Sam’s words seemed quite sincere, ill-chosen as they are. Most ironically, Sam’s little speech here will later come back to haunt him.
Convinced that his message has been received, Sam then asks “who wants to go” to Mars? There is a brief silence in return from the men, then Sergeant Imoto steps forward.
“Is it permitted to disagree with the General, sir?” asks Imoto. Sam assures him that it is.
“In my humble opinion, sir, there is an excellent reason for this voyage.”
“Well, suppose you tell us about it,” replies Sam, giving Imoto the floor to speak.
“Some years ago, my country chose to fight a terrible war. It was bad. I do not defend it. But there were reasons. Somehow those reasons are never spoken of.
“To the Western world at that time, Japan was a fairy-book nation. Little people living in a strange land of rice-paper houses. People who had almost no furniture… who sat on the floor and ate with chopsticks. The quaint houses of rice paper, sir, they were made of paper because there was no other material available. And the winters in Japan are as cold as they are in Boston.
“And the chopsticks? There was no metal for forks and knives and spoons, where slivers of wood could suffice. So it was with the little people of Japan, little as I am now. Because for countless generations, we have not been able to produce the food to make us bigger.
“Japan’s yesterday will be the world’s tomorrow. Too many people and too little land. That is why I say, sir, there is urgent need for us to reach Mars: To provide the resources the human race will need if they are to survive. That is also why I am most grateful to be found acceptable, sir. I volunteer.”
“Thank you, Sergeant Imoto,” says Sam with sincerity. “You’re not a little man.”
COMMENT: This speech by Imoto is one of the key events that viewers remember from Conquest of Space, especially more modern audiences – just not in a very favorable way. It is a mid-Twentieth Century Westerners’ take on why Japan joined the Axis powers in World War 2, made even less palatable by being condensed into a very brief commentary. Note how none of the other crewmen from countries outside of the United States had to justify their presence on the station and their reasons for wanting to go to Mars on a cultural level.
When Conquest premiered, it was just under ten years after the end of that global conflict. For most contemporary audience members, WW2 was quite recent history, with even greater meaning for the many who had served in the military then. That Producer Pal included a person of Asian descent playing a scientist and astronaut from Japan in an otherwise white main cast was bold and progressive for an American cinematic production in 1955. Outside of this speech, Sergeant Imoto is one of the most intelligent, educated, and emotionally stable characters in the film. As we will see, Imoto’s expertise will be largely instrumental in procuring the success of the primary reasons that the Space Authority chose Mars as their “final objective.”
The real reasons Japan entered the Second World War are naturally more complex and multifaceted than Imoto could have possibly given in such a short time. The quest for more resources was indeed one goal for the actions of Japan’s leadership, but it is only part of the whole story. The multiple references to the height of the average Japanese person, the ways Imoto claims the nation lives, and why they are supposedly perceived so, I feel deserves no acknowledgement.
The other real purpose of Imoto’s near-final words – “Japan’s yesterday will be the world’s tomorrow. Too many people and too little land. That is why I say… there is urgent need for us to reach Mars: To provide the resources the human race will need if they are to survive” – back up and justifies why humanity as a whole must reach and explore the Red Planet as conceived in this film: To find new resources to support an ever-growing technological civilization that can no longer be confined to a single world without serious consequences.
It doesn’t help that General Merritt’s reactions and expressions, although meant to be sincere and respectful, come across more as condescension towards Imoto, especially his comment “You’re not a little man.”
Just to add the icing to this cultural cake, the actor Benson Fong (1916-1987) was born in California and descended from China. Fong spent much of his career playing different Asian roles regardless of his true ancestry. This is a long and common practice for Hollywood that has deliberately diminished in recent years but has yet to entirely vanish.
Sam asks if there are any more volunteers for the revised space mission.
With a confident smile, Mahoney steps toward his old friend.
“Look, General, sir, you wouldn’t want these helpless infants along on a mission like this,” declares Mahoney. “Now, a couple of tough old soldiers like ourselves…”
“You’re not going, Mahoney,” answers his old friend. The Irish sergeant is naturally taken aback.
“I’m within me rights to demand a reason.”
“You’re twenty years too old,” is Sam’s immediate reason.
“Too old?” Mahoney fires back. “I am three months younger than you, Sam Merritt, and with twice the endurance. Who was it carried you on his back for seven miles after the Battle of Bloody Hill in 1952, when all the breath you had left in you wasn’t enough to blow out a candle in a Halloween pumpkin!”
FACT CHECK: Assuming Mahoney is referring to the Korean War, which took place officially from 1950 to 1953, I could not find any Battle of Bloody Hill listed from that conflict. There was a Battle of Bloody Ridge during this war, but it was fought in 1951. If nothing else, Mahoney’s comment adds one more bit of support that Conquest does take place either in or about the year 1980.
“Now, I said no, Mahoney,” Sam shoots back, almost as if he is scolding a disobedient child. “Now, you angled your way onto this Wheel against my specific orders, and you’re not gonna bull your way onto the Spaceship.”
Sam juts his right index finger into Mahoney’s face. Fenton and Barney are visible in the background: Fenton seemed bemused by this exchange, while Sam’s son remains more reserved.
“And if you say another word, I’m gonna have you thrown into solitary for a month! And tied to a wheelchair when you get out!”
COMMENT: So, the Wheel has a jail, or at least a section dedicated to securely isolating those who commit violations aboard the space station? Interesting. As for Sam’s mention of a wheelchair: If everyone on the station must be physically fit, how does the apparent presence of that piece of medical equipment fit into this rule? Or does one who breaks the law in space gets an automatic ticket back to Earth instead to be dealt with there?
Chagrined, Mahoney turns away and merges back into the group without saying another word.
“I’II go, sir,” Fodor says rather somberly. Sam acknowledges Andre with a nod.
“Well, sir…” Sergeant Siegle chimes in, with some obvious reluctance. “Well, I’d hate to see everybody eat with chopsticks, so… Check!”
The General walks up to Donkersgoed and Sanella.
“To you men, our thanks, for your patience and all your sacrifices,” says Sam to these two men. “The Earth rocket leaves in two hours. Get packed, huh?”
Sam then dismisses everyone and heads for the meeting room exit. Siegle quickly runs over to open the hatch for General Merritt. Sam briskly leaves the room, with Fenton and Barney right behind him.
These three are barely gone when Donkersgoed and Sanella break into exuberant cheering and gesturing for each other, clearly happy about not having to visit Mars. They affectionately slap their colleagues on their arms and rush out the open hatch, with Fodor following next.
Siegle and Imoto face each other at the hatch.
“Speechmaker,” Siegle calls his friend.
“Chopstick,” Imoto slings back.
The two laugh and continue the group arm-slapping behavior with each other, then head out.
Ignored by the others, only Mahoney is left in the room. Obviously disgruntled, the Sergeant makes an even-paced exit, with certain thoughts coming to his mind along the way. It is not hard to guess that this will not be the last we see of Sergeant Mahoney.
The Female of the Species
The scene changes to something seemingly out of place: Against a bright yellow background, a man in a pink silk outfit wearing a pink turban is carefully walking among a large group of scantily clad women sleeping on the ground. He is playing an instrument normally associated with snake charming to wake up the women, who soon start dancing seductively.
As the scene pans out, we discover we have neither been transported to some kind of Westernized Arabian fantasy world, nor did some editor accidentally slip in the wrong film: We are still aboard the Wheel twirling about in space. The men of the station are watching an entertainment program on a large viewscreen aimed straight at their libidos and responding as imagined.
One man who is not partaking of this event is Captain Barney Merritt: He is sitting at a table in the back, smoking a cigarette while writing a letter to whom we can only assume is his wife Linda, explaining why he won’t be coming home to her any time soon. One rejected missive draft lies crumpled up near him on the table.
Meanwhile, Sergeants Imoto, Siegle, and Fodor are enjoying the broadcast show among the crowd, with the first two smoking as they watch the lead woman of this production, who is wearing a bright pink outfit, crooning about making love to some sheik “on the desert sand.”
While not outwardly behaving as their colleagues are to what is being shown on the big screen, Imoto and Siegle are “inspired” by these somewhat exotic women to discuss the possibility of life on Mars, in particular the potential for female life there. They are concerned, however, as to what kind of female organisms they might encounter on the Red Planet, for they may look nothing like the beautiful women gyrating before them. Ah, science.
COMMENT: This musical scene was taken from another Paramount Pictures film titled Here Come the Girls, released in 1953. A comedy vehicle for Bob Hope (1903-2003), the uncredited lead singer in pink was Rosemary Clooney (1928-2002).
Due to Conquest, I took the time to find and watch this film. While never destined to be a cinematic classic, Hope made it entertaining and humorous enough, as he was still in his comedy career stride. The comedian played Stanley Snodgrass, a very untalented and uncoordinated chorus “boy” in numerous Broadway musicals in the year 1900, which he usually gets fired from for being so bad at his job. Clooney played as Hope’s acting co-star and long-suffering girlfriend.
To the boos and jeers of the station crowd, the beautiful women occupying the large viewscreen suddenly vanish, replaced by the words SPECIAL BULLETIN and someone saying: “We interrupt this program to take you now to the press room of the Trans-World Communications in New York City.”
The large white block text is quickly replaced by a male television announcer sitting at a desk strewn with two microphones, a telephone, and of course a clear glass ashtray. International clocks and two Teletype machines line the wall behind him. The announcer reads the following from a piece of paper in his hand to the camera:
“Ladies and gentlemen, it has just been revealed by the Security Office of the Supreme International Space Authority that within the next 24 hours, exact time to be given later, Man’s first spaceship, built and commanded by General Samuel Merritt, will blast off on the most fabulous voyage ever conceived by the human mind. Their destination is the planet Mars.”
The crewmen around the trio in blue are stunned by the news, asking them if they really are going to the Red Planet.
“You want our autographs, peasants?” Siegle jokingly asks their admirers.
The announcer on the screen continues.
“We take you now to our permanent station on Mount Palomar.”
The screen shows the Wheel and the Spaceship at a distance, presumably being imaged by the giant 200-inch (5.08-meter) Hale reflector telescope on Mount Palomar, which was the largest such astronomical instrument in the world at the time, keeping that record until 1993. A couple of the crewmen even stand up and point at the screen, remarking upon realizing it is them as seen from Earth.
“There they are, ladies and gentlemen, the satellite and the Spaceship,” declares the TWC announcer. “The dream of all mankind come true. The moment is almost here, the moment for that creation of the Wheel to launch out into limitless space to carry five heroic men to a new planet and to immortality!”
As the announcer waxes poetic, we see brief scenes of Barney Merritt still writing his letter and Sam Merritt in the privacy of his office, surrounded meaningfully by his medicine and intently reading a small black bound book.
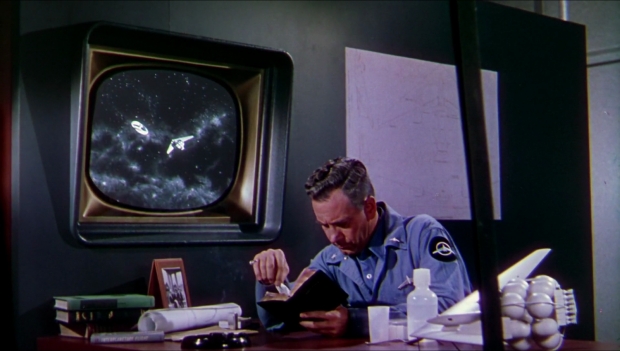
FIGURE 5. This brief but meaningful scene encapsulates all that General Samuel T. Merritt has gone through and will go through before this journey is over.
The announcer carries on.
“And now, ladies and gentlemen, please stand by while we close our circuits for the final special event of the evening: A private last look at the world for the heroic men of the Wheel alone. Take it away, Vienna!”
The screen changes to some place in the capital city of Austria with a curtained backdrop, where one balding man is speaking in their native tongue to an older woman wearing a deep maroon colored coat and a simple scarf. Fodor stands up, staring at who he sees on the screen.
The man in Austria grasps a long silver microphone and speaks into it while looking at the camera.
“This lady is Mrs. Heinz Fodor, gentlemen, the mother of Andre Fodor.”
The man gives the microphone to Mrs. Fodor and explains to her in Austrian how to use it. Fodor walks closer to the screen. She is obviously a bit nervous but also quite emotional for her son, which is apparent in her speech to him.
“Andre. Andre, my boy, this is your mother. Can you hear me? What you are doing must be good. You are a good boy. Please, Andre, be careful. God watch over you, my boy, and bring you back to me.”
The man with Fodor’s mother retrieves the microphone and gives the transmission back to New York City.
The new scene on the screen is the opposite of what we just witnessed: Instead of a caring, doting parent, we are introduced to one Miss Rosalie McCann, Sergeant Siegle’s girlfriend Rosie. She appears as a platinum blond wearing a revealing blue dress with a dark fur stole draped over her shoulders. Long black dress gloves and a sparkling diamond necklace complete her wardrobe. The woman is clearly aware she is on television.
Jackie is barely able to contain himself. The guys in the room, who were respectfully silent when Andre’s mother was speaking to her son, go back to acting just as they had when watching the video of the women dancers.
“Mine, all mine!” shouts Siegle to the entire station.
“Do you miss me, Jackie, baby?” asks Rosie in a seductive voice. “I miss you, honeypot.”
Ms. McCann continues in a manner that I will only describe as Marilyn Monroe (1926-1962) on a budget.
“You’ve been away a long time, Jackie, but where love is concerned, what’s a year this way or that when the flame of love is burning? And it’s burning, Jackie, right here, forever.”
“In here, too, Rosie, baby!” declares Jackie.
“For me, there never could be anyone else but you. Never, ever, ever!”
The pair’s riveting conversation is suddenly interrupted by a man’s voice off-screen beckoning Rosie to leave. She asks this unseen person to wait a minute. Jackie has an immediate change of attitude.
“So, I won’t say goodbye, Jackie,” Rosie carries on. “Just farewell.”
The unseen presence tells Rosie to “come on.” Rosie shoots back “In a minute, Sidney!”
“I gotta hurry now, Jackie,” says Rosie. “Mama’s waiting.”
“Mama!” Jackie exclaims in disbelief. “Oh!”
Rosie gives Jackie one last goodbye, then blows kisses at the screen. Jackie wipes them off his mouth in disgust.
“Don’t forget to bring your Rosie back a nice souvenir from Mars,” she adds.
“Souvenir!” Jackie shouts. “You two-timing tomato! For that Sidney I got a souvenir!”
Jackie rushes up to the screen, ready to smash it to get at his rival.
“Come on out, you rat! I’II fight you! I’II throttle you! That’s what I’II do!”
Several crewmen grab Jackie and restrain him before he can do damage to the viewscreen and possibly himself in the process. This rather absurd scene ends abruptly; we are immediately transported outside the Wheel, where we witness the Spaceship blasting off for Mars!
COMMENT: This scene, where a good deal of screen time is taken up just to see how women are considered in Conquest, is one of several which made me question how serious Pal and company wanted this film to be taken as a realistic depiction of humanity’s near future. It is clear that gender equality was not on the table; then again, if you study how people in that era often thought about how life would be for their descendants, the focus was more on technological advancements compared to social ones. Conquest is certainly no exception here.
COMMENT 2: The abrupt scene change from Siegle railing over his “two-timing tomato” to the Spaceship noisily heading into deep space made me wonder what the thinking was here. Were the producers also embarrassed by the displays of “floozies” and the men’s reactions to them, Sergeant Siegle’s in particular, so they wanted to get on to the space adventure as quickly as possible? Did the filmmakers want to ride on the base excitement generated by that scene and “convert” the energy into a rocket launch?
Why did we spend so much screen time on dancing women, Fodor’s mother, and Siegle’s now ex-girlfriend? I suggest it was a combination of budgetary limitations and the producers thinking they needed to pander to general audiences’ expectations of the day. Conquest was not a typical film for its time, and, at the end of the day, Hollywood was and is a business designed to make profit from its products. If anything, the effort to make Conquest seem relatable to the masses among all those space vessels and alien worlds only hurt its reputation. Most audiences came to see something different in this case but left with a standard World War 2 buddy film set in the Final Frontier.
“Set Your Gyros for Mars!”
As stated above, one minute we are watching Sergeant Siegle try to tear his way through a big viewscreen to get at his romantic rival, the next we are in space seeing a giant winged spacecraft blast off to another planet. We observe the Spaceship slowly but surely gain velocity as it roars away from the Wheel in a wild trail of smoke, flames, and sparks.
COMMENT: As with many science fiction films set in space, both then and now, Earth-bound audiences expect their spacecraft to make noises even in a near total vacuum environment. Yet another area that Conquest just wasn’t bold enough to be a cinematic pioneer.
Inside the spaceship, we and the mission crew watch the velocity of their vessel increase thanks to the labeled Space Speed Indicator (with the notation Miles Per Hour below, along with Made in U.S.A. in even smaller script) with its moving red needle. The men also know they are moving faster due to the gravitational equivalent forces, or g-forces, upon their bodies, which we the audience see up close in the increasingly distorted faces of General Merritt and Fodor – and rather grotesquely at that.
With some difficulty, Sam reaches for the throttle with his right hand to cut off the rocket engine power, succeeding after several painful moments.
The ship engines stop firing and their sound is replaced with that same near-dreamlike music we encounter most every time there is a non-dramatic scene set in the void. Earth is now a waning gibbous blue-green globe steadily shrinking into the distance.
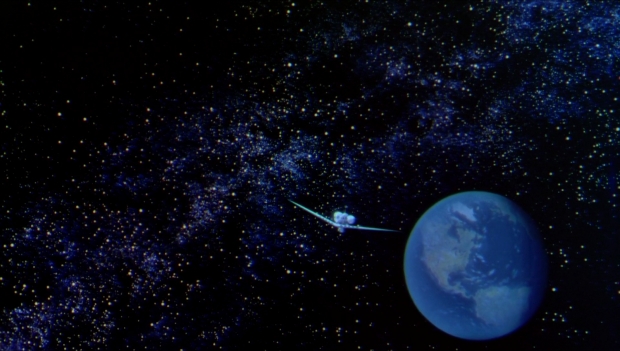
FIGURE 6. Spaceship One sails away from Earth at 20,000 MPH as the vessel begins its long journey to the planet Mars.
“Holding 20,000 miles per hour, sir,” reports Imoto as our view of the Space Speed Indicator confirms this.
“Set your gyros for Mars,” orders Sam as he catches his breath. Barney confirms his father’s order.
Viewing some distance away, we see the winged ship make a long, curved upward movement against the starry background. Our view inside the vessel will now be mostly a vertical one – just forget about there being no real up or down in space.
The men begin to unstrap themselves from their cushioned seats.
“On course, Captain?” Samuel asks his son.
“On course,” replies Barney.
Just below Sam and Barney, we see Siegle, Fodor, and Imoto still reclined. Siegle and Imoto engage in their usual friendly banter after a memorable incident.
“You were saying, Sergeant Brooklyn?” asks Imoto rhetorically as they all remove their safety belts.
“I was saying, Sergeant Imoto,” replies Siegle, “that the next time, I am definitely gonna try the train!”
Sam calls down to the rest of the crew.
“You men can get out of those couches now. We won’t need them again till we land on Mars.”
“Ain’t that just grand, Sergeant?” Siegle says with irony. “Nothing to worry about for millions and millions of miles!”
Siegle climbs out of his couch and slides down the long metal ladder dividing the crew couches, heading towards the ship level where there is a small black control panel and a tall thin microphone. This is the radio communications “room”, although in truth it is just one small open corner of the ship.
As Siegle is moving towards the radio, a voice can be heard emanating from the device.
“Spaceship, Wheel calling. Come in, over.”
Siegle sits at the console and flicks on a toggle switch.
“Spaceship One to the Wheel,” answers Siegle. “Come in, Wheel. Over.”
“Hello, Spaceship One!” replies their base.
COMMENT: This is the closest we get to an official title for the rocketship taking them to Mars and the only time any formal name for it is spoken in the film. Why they would not give the first manned space vessel to Mars an actual designation seems unrealistic. The earliest satellites and manned spacecraft of the Space Age all had names, both their model type and a more specific name for each mission.
Granted, it is their only ship made by humanity sending a manned crew to Mars, but I doubt that no one, especially the crew, would leave it unnamed. The space station seems to have no formal name, either, but they often call it by its nickname, the Wheel. In von Braun’s previously mentioned Mars novel that influenced Conquest, their space station was called Lunette, for Little Moon.
They could have called it Ares One. Not terribly original, but at least it would make sense, especially since this is an international effort and space agency. After all, the rocketship in Pal’s film Destination Moon was called Luna, the Latin word for Moon. The Soviets had a long habit of calling their lunar and planetary missions after the celestial body the probe was being sent to explore plus the sequential number– unless it failed, then it was given the cover name of Cosmos or sometimes Zond, the latter which simply means Probe.
Other fictional examples include Ares III in the 2015 science fiction film The Martian and Ares IV in the Star Trek Voyager episode “One Small Step”, first aired in 1999.
TO ADD: Ares was the ancient Greek god of war, the basis for the Roman god of war, Mars. This name was given to that planet due to the reddish hue it has as seen from Earth, undoubtedly reminding a culture based on power struggles, conquest, and overall strife of the color of the blood spilled so often in such conflicts.
In one respect, calling the fourth world from Sol after a war deity, based on what we now know scientifically about Mars, would appear to be quite off base. However, if you look at it from the perspective of the multiple prices paid by humanity to be able to reach other worlds such as the Red Planet, then it does make a sort of sense. After all, rocketry evolved from its initial use as a weapon of war going back to the ancient Chinese.
“Professor Fenton for General Merritt,” orders the man from the Wheel. Siegle calls up to Sam, who also slides down the ladder to reach the radio. Siegle stands at attention next to the General as the latter man speaks next.
“How are we doing, George?” greets Sam to his friend, Dr. George Fenton.
“Good, General,” is Fenton’s response. “You’re getting a bigger push from Earth than we anticipated, however.”
“Is our cotangential orbit correct?” inquires Sam, who is asking if the ship will meet up with Mars at the proper time and place.
“We’re computing it now,” replies Fenton. “Keep your radio open. We’ll be in constant communication as long as possible.”
“Incidentally, TWC has okayed relay broadcasts for morale,” Fenton adds. “Your boys like to hear some mood music?”
Sam’s face darkens a bit and he becomes somber.
“Thanks,” the General replies. “For this mood, there is no music.” The conversation ends, undoubtedly leaving Dr. Fenton and anyone else listening in perplexed by Sam’s final comment.
COMMENT: Was the crew of Spaceship One not allowed to bring any personal reading or music playing devices with them on this mission? What about a general library, perhaps on microfilm since I presume the spacecraft interior volume and weight would be at a premium? How about games like cards, checkers, and chess? Why did the crew need permission from Trans-World Communications to listen to morale broadcasts, in particular “mood music”?
Whether it be in space or in the oceans via submarines, long duration missions without some form of entertainment and distraction for the crew are not healthy psychologically. This was a known fact way before Conquest came along. This Mars-bound crew would have no places for “shore leave” or any kind of pit stop while confined to some rather small living areas on the ship.
No Unnecessary Floating
Sam orders Siegle to monitor the radio. As Sam leaves, Siegle sits down next to the control panel and lets loose a big exhale of air, presumably affected by the General’s comment regarding his mood. Jackie leans his head on his left hand with his elbow resting on the panel, lost in thought.
We then discover that Siegle’s black boots are unzipped. Although he isn’t moving much, Siegle somehow manages to float out of his footgear and slowly sail upwards, which he doesn’t seem to notice at first.
As this is going on, Sam is giving orders to Imoto and Fodor off screen to “go aft and check the tail pipe temperatures.”
COMMENT: Are there no gauges or other instruments in the rocketship’s main control room for indicating temperatures in the ship’s propulsion area? This duty seems like one of the things that could be done remotely, for reasons that include both time and safety.
Siegle’s flight path is suddenly interrupted when he bumps his head on the back of one of the couches, waking him out of his stupor. Jackie slaps the side of his head with his hand in exasperation at his antics.
“Look at him! He’s off again!” cries Imoto, witnessing his friend’s predicament – and apparently not for the first time.
Sam appears and rather roughly grabs Siegle’s left leg to pull him back down.
“We went to a lot of trouble to develop those magnetized shoes,” intones the General just inches from Siegle’s face. “Now, you get back into them and keep them zipped up!”
Sam then turns meaningfully to Imoto and Fodor, who are standing to his immediate left.
“We’ll have no unnecessary floating aboard this ship,” he demands.
Siegle catches himself starting to rise again and quickly zips up his fancy space boots. Meanwhile, Imoto and Fodor pull up a big white circular mesh deck plate so they can go aft and check on those tail pipe temperatures.
As Imoto holds the loose plate, Fodor looks down the access tunnel – and sees one of their white spacesuits silently floating up towards him!
“General! Look!” Fodor cries out.
Sam says to “grab him” and “put him over there” regarding the spacesuit. The men lay the suited figure on the deck. Fodor kneels and cradles the person’s helmeted head in his arms.
COMMENT: How did Sam et al know there was a person in that spacesuit? What if it was just an empty suit that came loose during the launch phase? There was blood smeared on the interior faceplate of the helmet, which might have been a clue, but the organic mess also kept whoever could be in there an initial mystery.
The men remove the helmet to discover the suit’s occupant is… Sergeant Mahoney in an unconscious state! The crew sees that the lower half of Mahoney’s face and ears are bloodied.
“Don’t just stand there,” Sam shouts to Siegle. “Get some water!” Jackie acknowledges his superior officer and moves quickly to obey this order.
“Of all the stupid, harebrained things to do. Stowing away on a spaceship!” Sam adds to the room.
“What a beating he must have taken during the blastoff!” notes Barney.
“Wake up, you insubordinate lunatic!” Sam shouts in anger and frustration at Mahoney, who remains unaware of his surroundings. “Thirty years in the Army and still too brainless to obey an order. Wake up, so I can have you shot!” Sam grips and shakes the suit’s right breathing tube to rouse Mahoney.
“He’s coming to,” declares Imoto.
“What are you doing aboard this ship?” demands the General.
Mahoney reaches into his suit through the neck ring and pulls out a familiar green dental instrument inside a transparent container.
“You forgot your toothbrush,” answers Mahoney with a weak smile.
Sam grabs away Mahoney’s “gift” but then concedes through his facial expressions that he can’t really stay mad at his best friend and companion of over three decades, despite the seriousness of his actions.
COMMENT: So how did Mahoney get aboard the spaceship? Was no one monitoring the vessel? Surely there were a constant stream of crewmen present preparing the craft for its now quite extended mission. As Mahoney was already denied a seat on the ship by the General himself and he would have required a space taxi to reach Spaceship One from the Wheel (or, My God, did they expect people to just float over from the Wheel to the Spaceship and back unassisted?), what excuse did Mahoney give to get over there?
Next set of questions: Did no one on the wheel miss Mahoney at any point? Once his job to babysit his “bluebirds” was complete, Mahoney must have been given a new assignment aboard the Wheel which would require his reporting to a new and different authority figure, presumably a station staff officer. Perhaps Mahoney might even have been ordered back to Earth, as his friend and protector would be gone from the space station for a long while: Sam did mention that the Sergeant “had angled [his] way onto [the] Wheel against [Sam’s] specific orders.” Another official might take note of this aspect of Mahoney’s career and send him home in part as a reprimand for disobeying a superior officer.
Beyond all this detail, Mahoney was also the kind of boisterous character one would be hard to miss, especially in the limited confines of a space station.
I have to wonder if Mahoney was already thrown out of the service for this insubordination in absentia once the Space Corps learns the Sergeant has deserted his post. The Earth authorities may not be able to do much of anything directly to Mahoney while he is millions of miles and several years away from them; however, once he returns, Mahoney can assume his long military career is over – and that would be but the very least of his legal troubles.
My final and most important question set: Putting aside Mahoney’s multiple breaches of military etiquette, doesn’t the Sergeant’s mere presence put the whole mission in jeopardy? Since Sam stated that only five individuals could go on this expedition, this would mean there is not enough food and other necessary resources for a sixth person, excluding any presumed emergency supplies. Did Mahoney at least bring his own cache of meal pills before stowing away?
I also wonder if Mahoney’s physical presence, meaning his body weight, will have a negative effect on the mission parameters, since again the expedition to Mars is only planned and balanced for five astronauts, not six. In Destination Moon, mass became a critical factor when the Luna crew discovered they used too much fuel when landing upon the Moon and had to shed a certain number of pounds to achieve escape velocity and make it back to Earth. At one point it even seemed that one of the four mission astronauts would have to stay behind on the lunar surface to ensure the survival of the rest.
One final final question: What skills, if any, does Mahoney bring to this mission, aside from being the now former overbearing monitor of a small group of adults? We and the crew of Spaceship One will now be forced to find out.
Too Perfect to be Accidental
Our next scene is an external view of the Spaceship careening through starry space. If one looks carefully, you can see those big wings wobble just a bit – as if the vessel is some kind of model!
Inside Spaceship One, Barney is at the stellar navigation station charting their course to the Red Planet, looking out at space through the transparent dome. Sam is just behind his son pacing the deck and thumbing through his little black book, which turns out to be a Judeo-Christian Bible – the King James Version, perhaps?
Barney seems to know what his father is doing without even looking at him first.
“I don’t remember you reading the Bible so often, sir.”
“It’s the one book you never really get through reading,” Sam replies. “Man’s every move, his every thought, his every action is in there somewhere, recorded or predicted. Every move except this one.”
Sam continues with his near soliloquy.
“According to the Bible, Man was created on the Earth. Nothing is ever mentioned of his going to other planets. Not one blessed word.” Sam moves right behind Barney, looking out through the dome with him into space.
“At the time the Bible was written, it wouldn’t have made much sense, would it?” Barney inquires, looking up at Sam.
“Does it now?” is his father’s evasive response. “The Biblical limitations of Man’s wanderings are set down as being the four corners of the Earth. Not Mars or Jupiter or infinity. The question is, Barney, what are we? Explorers… or invaders?”
“Invaders?” asks Barney, genuinely puzzled. “Of what, sir?”
“Of the sacred domain of God… His heavens,” answers Sam. “To Man, God gave the Earth. Nothing else. But this taking of… of other planets… it’s almost like an act of blasphemy.”
“But why?” asks Barney. “They belong to no one else.”
“We don’t know that,” Sam replies.
“But look, sir,” Barney implores his father. “It couldn’t be just an accident that at the very time when Man’s resources on Earth are reaching an end, Man develops the ability to leave his own world and seek replenishment on other planets.”
“The timing is what fascinates me,” Barney adds. “It’s too perfect to be accidental.”
“Those other planets might already be tenanted,” Sam declares, and he may not be wrong, although both father and son are most likely referring to intelligent life forms, not just any kind of organisms such as microbes or plants.
“I don’t think so,” rejects Barney. “The Universe was put here for Man to conquer.”
“I don’t know. I… I just don’t know,” is all Sam can say, looking weary and confused from this impromptu debate.
Barney suggests to his father that he get some sleep, which Sam agrees to and wishes his offspring good night as he marches up the ladder. Barney looks ahead again at the stars, though his face betrays the worry he feels at his father’s comments.
Asteroid, Dead Astern!
We transition to the next scene with a repeat of Spaceship One traveling through interplanetary space, ethereal music and wing wobbles included.
We find Barney staring at a medium-sized viewscreen full of bright stars. The Captain is flicking a panel toggle switch on and off repeatedly. The stars on the screen begin to get overtaken by a series of expanding wavy black diagonal lines before going completely blank.
“Topside view is jammed,” Barney announces to his father, who is sitting next to him. “Can’t move it.”
“Sergeant Siegle, Sergeant Fodor, get out there and free that pickup,” order Sam to the two men, who are just a few feet away from him.
“Out, sir?” Siegle nervously asks the General. “But the ship is going 20,000 miles an hour, sir!”
“So are you, Sergeant,” answers Sam. “You won’t fall off. The operation’s the same as it would be on the Wheel. Get going.”
Fodor is amused at Siegle’s reactions to him as they walk off to perform this duty and the scene fades to change.
COMMENT: I would think this bit of space physics would be taught and well known to anyone venturing into space, even Siegle. After all, a vessel needs to be moving at least 18,000 miles per hour (28,968 kilometers per hour) just to keep circling Earth, as the Wheel does. I realize this is Siegle fulfilling his story job as the Question-and-Answer Man to the general audience, in particular the pre-Space Age one of 1955, just before the dawn of orbiting satellites.
Outside the ship, Siegle and Fodor are carefully walking along the length of its silvery hull. Suddenly Siegle stops and bends forward a bit.
“Look at her,” Siegle says to Fodor, pointing at a beautifully rendered blue and green Earth in the distance. The Western Hemisphere is facing them, lacking a remarkable amount of cloud cover.
“If we ever get back, you know what business I’m really going into?” Jackie asks, then gives himself the answer. “Real estate.”
“Cut out that chit-chat up there and fix that pickup!” bellows General Merritt over the radio, publicly stinging Siegle once again for idle conversation while he is working.
“Yes, sir,” Siegle replies, almost wearily. He then adds an ironic comment out loud, despite what just occurred.
“That’s what I love about this job: The privacy!”
Fodor attaches himself to the ship via a long tether then walks ahead up the tower holding the pickup at top, a rather large radio dish.
At the base of the dish on the tower, the two men stop and begin their repairs. As Fodor braces an interior portion of the tower with his hands, Siegle uses a type of bolt tightener tool on the mechanism.
“Try it now, sir,” Jackie requests.
Barney toggles the panel switch back and forth. The screen flickers with his actions, then the bluish white stars come back into sharp relief.
“It’s okay now,” Barney answers Siegle. “You men go forward…”
Barney and the audience suddenly witness a large orange sphere appear in the center of the viewscreen, as if materializing out of nowhere.
“Asteroid, dead astern!” Barney alerts the crew.
Siegle and Fodor see the growing threat and quickly rappel down the tower towards the airlock.
The giant glowing asteroid looms right behind the ship. Sam activates the aft rockets, causing the ship to dive straight down below the space rock.
Its rockets still blasting, the spaceship levels off as the asteroid just barely misses it above.
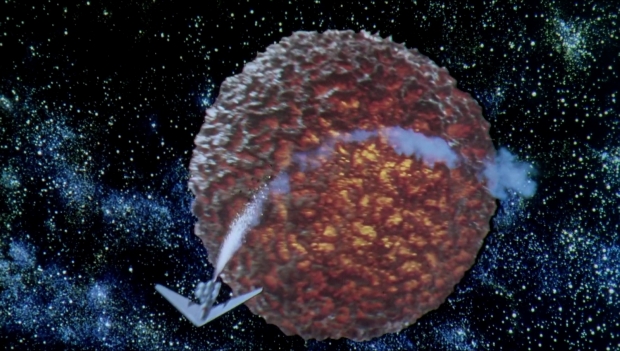
FIGURE 7. An improbable asteroid bears down on Spaceship One, which is just able to avoid the cosmic threat with some fancy maneuvering.
Siegle and Fodor are still outside the ship, crouched down together on its hull. They watch the intruder pass uncomfortably close over their heads.
“Come on, let’s get inside before they throw something else at us!” Siegle says to Fodor.
No sooner do the men stand up than small glowing objects begin to whizz past them and impact the ship with metallic clanging sounds. The crewmen inside rush to the transparent navigation dome to see what is making this noise.
“Meteor fragments!” Barney exclaims. The giant asteroid was trailing a collection of loose debris moving at very high velocities.
Fodor moves to unlatch himself from his safety tether when suddenly, one of the fragments smashes into Fodor’s abdomen, visibly tearing a hole in his spacesuit and ripping through his body! Andre horrifically screams in pain and is thrown across the length of the vessel, while the crew watches in helpless shock. The poor man is left dangling at the end of his tether, right in full view through the navigation dome.
Sam switches on the nearby radio and orders Siegle back into the ship airlock at once, then quickly telling Imoto and Mahoney to help the man, which they rush to comply.
QUESTIONS: Why was this asteroid glowing orange as if it were molten hot? Did it just have a collision with another asteroid and was sent careening in the direction of Spaceship One? If so, it seems to have retained its spherical shape and lack of impact craters quite well. Could it be undergoing some form of interior geological activity, despite being rather small for a member of its celestial class? Perhaps the regolith itself is colored orange due to some ancient volcanic eruptions, such as was discovered on the Moon during the Apollo 17 mission to the Taurus-Littrow valley in December of 1972.
As for the trailing debris that was fatal to Sergeant Andre Fodor, modern space expeditions have shown that some asteroids do have plumes of particles and small rocks flying off their surfaces – and for reasons other than impacts and volcanic/geyser activity. Carbonaceous asteroid 101955 Bennu demonstrated this phenomenon to the cameras of the OSIRIS-REx surface sample return probe during its two-year mission there in 2019.
A Fallen Comrade… Who Won’t Fall Away
The drama we just witnessed has not gone away, in the quite literal sense. The body of Andre Fodor is still floating outside Spaceship One after the mission’s encounter with the bizarre asteroid, dangling at the end of his tether. The suit’s faceplate is masked red with blood; more of Andre’s blood is splattered across the top of the observation dome!
Inside the vessel, the men are keeping themselves silently occupied – except for Sergeant Siegle, who is just staring at Fodor’s spacesuited body through the dome.
Mahoney appears from below deck, breaking the tense silence by asking “if anybody’d like some hot coffee… I could heat up a couple of tins.”
COMMENT: What happened with the coffee pills we saw the men consuming earlier in the film? So, does this mean the ship has a stove as well, maybe even a galley? How does this square with their diet of pills-only food?
I had similar questions regarding Forbidden Planet, where their starship had a crew of two dozen men who had to be fed for at least two years in interstellar space with their only stop being at the destination exoplanet. Their ship, C-57D, was not much roomier than the one in Conquest, yet they not only had a galley but a dedicated chef (Cookie) who made the meals! In my Centauri Dreams essay on that film, I asked how they were able to accomplish feeding the whole crew within a limited ship volume and no resupply stops: Concentrated food pills was one potential answer, yet why would they need a cook and a galley if all their food were constituted into pill form?
I know that these two science fiction films are not documentaries, Forbidden Planet especially; however, Conquest of Space is supposed to be a fairly realistic depiction of how humans might venture into space and the nearby worlds of our Sol system. A large segment of Conquest was already spent on what, how, and why the astronauts were supposed to consume nutrients during their mission. Yet the concept seems to have been dropped by this seemingly innocent comment from Mahoney.
Mahoney looks around, but no one answers him, or even bothers to look in his direction. Not even Sam.
Our attention returns to Siegle, who has stopped being the funny man of our story. As he continues to stare out the dome at his friend, Siegle starts to chant, steadily becoming louder and more frantic with each repeat of the phrase.
“Get lost, Fodor,” Siegle demands. “Get lost, will you? Get lost. Get lost. Get lost!”
Mahoney puts his hands on Siegle’s shoulders.
“Take it easy, lad. He can’t hear you.”
Seeing how this tension could turn critical, Imoto decides to speak up, aiming his comment at the General.
“Sir, shouldn’t someone go out there and…?” Even Imoto cannot finish his sentence at the thought.
In response, Sam stands up and grabs his Bible, then slowly walks towards the ladder while staring out the dome at Fodor, seeming not to be entirely sure of himself. Imoto reaches for the General as if to help him, but Sam puts up his hand to stop Imoto before climbing down the ladder towards the airlock to perform his duty.
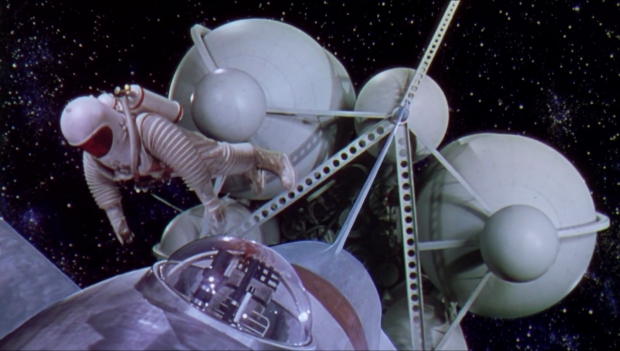
FIGURE 8. The still-tethered body of Sergeant Andre Fodor floats over the spaceship like a ghost, disturbing the surviving crewmembers inside in different ways.
COMMENT: Why was Fodor out there with Siegle in the first place? I did not see Fodor doing anything during the repairs that couldn’t have been done by anyone else on the crew. Fodor was the only qualified professional doctor on the ship, yet he was sacrificed for a relatively simple repair job. Worse yet, that asteroid was a giant MacGuffin anyway, an excuse to create some action and tension during their long interplanetary journey between Earth and Mars. Further, that asteroid looked and acted like no real asteroid we have yet seen and likely does not exist, trailing debris aside.
COMMENT 2: Meteor strikes were among the bigger concerns of space travel in the 1950s: Note how the Wheel was clobbered by an entire cluster of them large enough to shake the station and cause hull breaches, all without warning. This has made me wonder why the Spaceship wasn’t shielded against such objects, being so exposed as it was when circling Earth. Perhaps an Earth-orbiting drydock structure could have performed this double-duty for the vessel, along with an in-space alert system for rogue space rocks.
MORBID OBSERVATION: With Fodor’s untimely passing, now the ship should have enough resources for their unauthorized stowaway, Mahoney.
Though I’m Past One Hundred Thousand Miles, I’m Feeling Very Still
We find ourselves outside the ship with Sam standing on its hull, preparing to give a eulogy over the prone body of Andre Fodor floating in front of him. The deceased remains in his spacesuit, his torso wrapped in some kind of blue covering tied about him with a rope. Fodor’s blood still stains the interior of his helmet faceplate.
Using his Bible, General Merritt reads a text from the Book of Samuel:
“O Lord, rebuke me not, nor chasten me in thy hot displeasure. For thine arrows stick fast in me, and thy hand presseth me sore. There is no soundness in my flesh because of thine anger, and there is no rest in my bones because of my sin. Make haste to help me, O Lord… my salvation.”
BACKGROUND AND COMMENTS: This passage is from Psalm 38 involving the actions of David, the third king of the United Kingdom of Israel (lived circa 1000 BCE). In short: David wanted the wife of another man, so he ordered this man, an elite soldier in his army named Uriah the Hittite, to fight in a battle where the chances of him not coming back were high. David’s plan succeeded: Uriah was killed by enemy forces and David married the widow, whose name was Bathsheba.
As one might imagine, God was quite displeased with David and let the king know this through His servant, the prophet Nathan. David became very concerned to the point of illness after this visitation. Scholars believe that David wrote Psalm 38 while awaiting God’s forgiveness, which He eventually did as David was truly repentant for his actions. It also doesn’t hurt to have connections in very high places, either.
One might well ask what this biblical passage has to do with Sergeant Fodor and the answer is, not much. It is obviously Samuel T. Merritt projecting his own feelings and anxieties on their mission. Fodor’s death by cosmic forces, which Sam has undoubtedly seen as divine punishment and a warning to humanity for entering His sacred domain, has only exacerbated an already unstable situation for the General.
Once done with his reading, Sam gently pushes Fodor’s body away from the ship: The man’s mortal coil drifts off in the direction of our yellow dwarf star, until it is lost in the solar glare. Fodor has now become an interplanetary denizen, to circle Sol for perhaps millions of years, unless he is encountered by others before then.
COMMENT: I am assuming that Andre Fodor is among the first, if not the first, person in their society to receive a deliberate, if initially unintended, burial in interplanetary space – in part due to the fact that no space mission had even gone past the Moon before now. I have to wonder if anyone else in their reality had been given such a burial, such as one in a low Earth orbit where their remains would eventually burn up upon contact with our planet’s atmosphere. These would be the equivalent of a burial at sea, a tradition going back thousands of years. To give just one famous example: The first man to walk on the Moon, Neil Armstrong (1930-2012), had his ashes ceremonially interred in the Atlantic Ocean in 2012.
As for burials in space in our world, human ashes have been sent on flights aboard the Space Shuttle and into Earth orbit via Celestis, Incorporated. In 1999, astrogeologist Eugene Shoemaker (1928-1997) became the first, and so far only, human buried on the lunar surface when his cremated remains crashed along with the Lunar Prospector probe into the Moon’s southern polar region. Astronomer Clyde Tombaugh (1906-1997), who famously discovered Pluto in 1930, had some of his ashes placed aboard the New Horizons deep space vessel. After becoming the first probe to flyby Pluto in 2015, New Horizons exited the Sol system into the wider Milky Way galaxy, only the fifth such vehicle from Earth to do so. This made Tombaugh the first person to enter interstellar space, in a manner of speaking.
The Planet and the Blasphemy
Back in the Mars-bound ship, the cinema screen is filled with a black boxy speaker sectioned into eight connected segments. A voice from the vicinity of Earth is emitting from it.
“Wheel calling Spaceship. Wheel calling Spaceship. Come in, over.”
“The Wheel,” exclaims Siegle. “They’re trying to get us!”
Barney Merritt, who is already manning the radio station, motions with his hand for Jackie to be quiet.
“Spaceship to Wheel, we hear you. We hear you. Come in. Over.”
At the other end of the radio, there is only silence.
“Lost them,” declares Siegle.
“No, it takes time,” corrects Barney. “Millions of miles.”
COMMENT: Another “lesson” for Conquest’s contemporary audience, who might not know that radio signals travel at the same speed as light waves: 186,282 miles per second or nearly 300,000 kilometers per second. As distances in space are quite vast, even the highest velocity allowed by physics takes time to cross the vast void. Normally I might want to add that a supposed expert in “advanced electronics” as the General claimed Siegle to be should know this particular fact, but at this point it does little good anymore.
Barney keeps repeating his last message into the microphone, hoping for a reply from the Wheel. Meanwhile, Siegle walks over to General Merritt nearby, who is staring out the navigation dome at the planet Mars, which now looms rather large in their starry sky.
COMMENT: The apparent size of the Red Planet is the audience’s only indication of how much time has passed on this mission so far. The other notable feature is that while this depiction of Mars does display the “canals” that had been reported by astronomers since 1877 – and went on to do much to inflame the idea that the planet has intelligent life that built the canals to retrieve water from their polar caps to thirsty cities along the equator – they appear more subdued and resemble natural features compared to similar contemporary depictions.
“Getting bigger all the time, isn’t it, sir?” Siegle remarks to the General about Mars.
“Yes, Sergeant,” answers Sam, tightly gripping a metal strut. “The planet and the blasphemy.”
Siegle looks at the General with an expression of serious puzzlement.
“The what, sir?” Rather than explain his comment, Sam turns away from Jackie. Just then the radio crackles to life.
“Spaceship, Spaceship. Wheel calling Spaceship. This is Fenton. We’ve been calling you constantly for weeks. Come in, over!”
“Spaceship to Wheel,” answers Barney. “This is Captain Merritt. Stand by for the General.”
Barney excitedly turns towards his father.
“General, sir, the Wheel!”
As if coming out of a daze, Sam walks over to the radio. Barney vacates his seat so that his father can sit at the console.
“Merritt speaking,” Sam says wearily into the microphone. “Here’s the report: Lost course for several days due to near collision with asteroid. But we can still reach destination as plotted, which may be Mars… or hell.”
As Barney and Jackie look at their leader, stunned at his words to Fenton, Sam carries on with his diatribe, growing more frantic with each new sentence.
“This voyage is a cursed abomination. If it were possible, I’d come back now, return the ship to Earth — and blow it up!”
“General, please!” shouts Barney as he attempts to turn off the radio. Sam ignores his son.
“Together with all plans in existence for building another!” Sam stands up from the radio console and continues ranting while walking away from the two men back towards the navigation section.
“We’re committing Man’s greatest sacrilege! And we can’t stop!”
Barney sits back down at the radio and attempts some damage control on the situation.
“Professor, the General isn’t well,” Barney explains to Fenton. “Fatigue. He’s been suffering from severe headaches and insomnia. He’s very tired, almost exhausted.”
COMMENT: Space fatigue. The very ailment that got Roy Cooper thrown off the mission for having a momentary panic attack. Yet here is the General threatening to destroy Spaceship One and Barney tries to play it off as his father is just having a rough patch.
“We lost a crew member, Andre Fodor, struck by a meteor. The General took it very hard. I’m sure it’s only temporary. He’ll be all right, don’t worry. Everything else is under control.”
COMMENT: If this were a real deep space mission and its leader was spouting things like blowing up the ship, not to mention the multiple signs of mental instability he was showing well before this, the next in command would have been expected to take over the leadership of the crew and their expedition. The afflicted former leader would have to be restrained and confined until he could receive the proper treatment at some future point. However, this is a film of major inconsistencies and the reactions to Sam’s emotional breakdown are par for the course. There is also an important lesson here about the importance of impartiality when it comes to who is in charge and in the chain of command for such space missions.
As Barney tries to smooth over his father’s tirade to the Wheel, Siegle quietly moves to the nearby ladder and slides down it to the next level. Mahoney and Imoto are standing on either side of the ladder when Siegle arrives, looking at him expectantly. These two must have heard what was going on with the General, being so close to the deck opening and in such confining quarters, yet their next comments indicate no such awareness.
“Hey, you guys, we’re in trouble,” Siegle declares to the men upon his arrival.
“Off our course?” asks Imoto.
“No, the General,” Siegle corrects his friend and starts gesticulating as he keeps speaking. “You ought to hear the blast he just threw at the Wheel. Not just that Bible talk he’s been spouting lately. Crazy stuff. Things like blowing up the ship. Things like that.”
Siegle taps his forehead with multiple fingers.
“Gone. Just like Cooper.”
Mahoney suddenly grabs Jackie by the shirt collar and pulls him just inches from his face.
“Listen, you slimy little calumniator,” Mahoney insults Siegle. “If you were one-tenth the man the general is, you’d be twice the man you are!”
COMMENT: Calumniator (plural calumniators) – A person who calumniates, meaning they slander or make personal attacks upon others. In addition to Mahoney once again displaying his aggressive nature and overprotective behavior towards Samuel Merritt, I am surprised he knew this two-dollar word. To add, Siegle wasn’t attacking the General’s character, he was rightly reporting his very founded concerns over the man’s unstable expressions and potentially dangerous actions. Unfortunately, there was no proper channel available for Jackie to perform this duty other than attempt to warn the rest of his fellow crewmen.
However, I do not agree with Siegle’s assessment on Roy Cooper that he was “gone” mentally like the General. Cooper was suffering from the effects of severe stress imposed upon him by an overbearing regimen of work and discipline; he was never mentally unbalanced like Sam has become. As Dr. Elsbach said back on the Wheel: “Cooper will be perfectly normal… as soon as you return him to Earth.”
Mahoney carries on with his misplaced feelings for his unwell friend and throws in a physical threat for bad measure at Siegle.
“What he says or how he acts is not for you to criticize. And if I catch you doing it again, I’II pull out your filthy tongue and strangle you with it!”
Mahoney lets go of Siegle’s collar with a small shove. Siegle responds with a noise of disapproval and a dismissive wave of his hand before walking off. As he watches Siegle move out of sight, Mahoney climbs up the ladder to the next ship level, where he finds Sam staring at space through the navigation dome.
Mahoney walks behind Sam and grips his right hand on the General’s right shoulder. Sam turns.
“Sam. How about coming down to the parlor and having a little cup of tea with a lonesome old friend?”
Sam nods wordlessly and, with a look of gratitude and relief on his face, follows his “lonesome old friend” to this parlor.
COMMENT: So, no tea pills. either? And the ship has a parlor? Where? We also see that, despite his blustering and warnings to Siegle, Mahoney does know his friend is in trouble and quietly tries to help Sam in what limited ways he can.
For All Mankind
Mars appears much larger in size as the spaceship closes in on its target, until it seems to disappear into the planet.
The crew are gathered around the viewscreen as the surface details of Mars become visible to them: A mix of pale orange and dark brown swirls, along with a few smaller purple regions that could be lakes of water. Several large craters extend towards the world’s curved horizon. The men of Spaceship One are the first in their human history to see Mars so up close and clear.
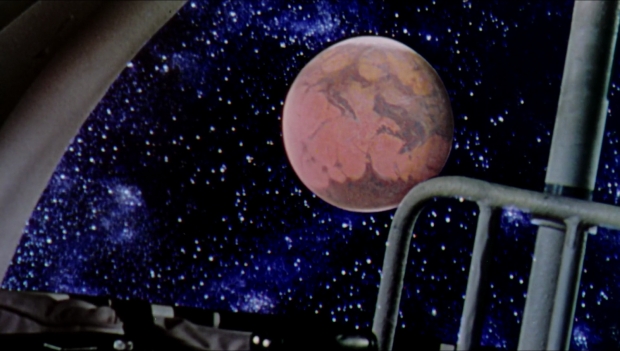
FIGURE 9. The crew of Spaceship One gets its first good look at their destination, a world that appears fairly close to what the planet Mars is really like.
COMMENT: Pre-Space Age plans to explore the Moon and Mars most often did not consider sending automated probes to those worlds first as would become the logical fashion. Instead, they would try to glean as much information as they could from Earth-based telescopes and then hoped the crews would be able to fill in the rest when they reached their destinations.
Thankfully, even our first manned attempt to reach the Moon with the Apollo program was preceded by a virtual fleet of robot vessels gaining as much data about our nearest space neighbor as possible before any astronaut’s boot would ever touch its surface. Mars has also been receiving a similar treatment since the first successful flyby probe, Mariner 4, sailed over the Red Planet in 1965 and returned the first in situ images and data.
“Look at her, all red-faced and pouting, like she might be angry with us,” Mahoney observes aloud about Mars in his own fashion. “And with us coming all this way to visit her.” Sam turns and looks at his friend meaningfully.
“Aft viewer, Sergeant Siegle,” orders Barney. “We’ve gotta release the boosters.”
“Hey, don’t we need them tanks?” Siegle asks (nope, not saying a word…).
“They’re empty,” Barney informs the man. “Just excess baggage now. The reserve tanks below will take us back home.”
Barney confirms that they are ready; Sam gives the order to jettison the boosters.
An exterior shot shows the booster tanks separating from the main ship over Mars. The braking rockets along the front of the long wings then kick in to slow the ship into orbit about the planet.
The crew watch from their viewscreen as the evacuated tanks hit the planet’s atmosphere and begin to burn up from friction with the atmosphere, making quite a show of their demise. Sparks trail the burning fuel tanks in a long bright tail like swarming fireflies. Soon enough the fireworks vanish and the tanks drift away, still glowing from the heat, to soon impact somewhere on the Martian surface.
“Look at it go!” exclaims Imoto about the receding tanks. “That’s what would happen to us if we hit the atmosphere that fast.”
“AII right, men, positions for landing,” declares Barney. The crew quickly moves to the ladder and scrambles up the metal rungs to prepare themselves in their designated couches. The last to respond, General Merritt slowly follows the others.
We see and hear Spaceship One firing its braking rockets along the front of the wings over Mars. Once through entry into the planet’s atmosphere, the white metal shields of the large cockpit windows spread open, revealing to the crew the alien world they will soon land upon.
As the planet looms larger in these framed windows, General Merritt begins to show some real distress on his face as he guides the vessel’s descent.
The spaceship appears out of the dark blue gloom of the Martian sky, looking almost like a bat gliding in. We move back into the cockpit, where Barney watches his father closely across the visually unappealing arrangement of the ladder and its cylindrical support beam dividing them and the rest of the crew into two rows.
“Slowing nicely, sir,” Sam’s son compliments him. “Clear landing ahead.” The Space Speed Indicator performs its function and shows the craft’s speed dropping rather swiftly.
Instead of answering Barney, Sam begins to chant the word “No” several times.
“We mustn’t. We can’t,” the General then declares. “We haven’t the right!”
Sam suddenly grabs the throttle and slams it forward as far as it can go. The ship begins to accelerate while still plunging towards the surface.
“General, stop!” Barney shouts at his father. “What are you doing?! You’ll kill us!”
Barney scrambles over that awkward ladder arrangement towards his father and desperately pulls at his throttle-pushing arm with both hands. The red needle on the Space Speed Indicator begins climbing back up several thousand miles per hour each second as the ship’s aft rockets blast away.
Sam tries to remove Barney’s grip on his arm, but his son quickly wins this struggle and Sam is slammed back into his seat, making his hand fall away from the throttle.
Barney pushes the throttle back with one hand while using his other appendage to keep his resisting father from trying to crash them again. The forward braking rockets fire in unison, making a long trail of white smoke as the winged vessel slowly begins to drop its velocity again over the rock-strewn Martian landscape.
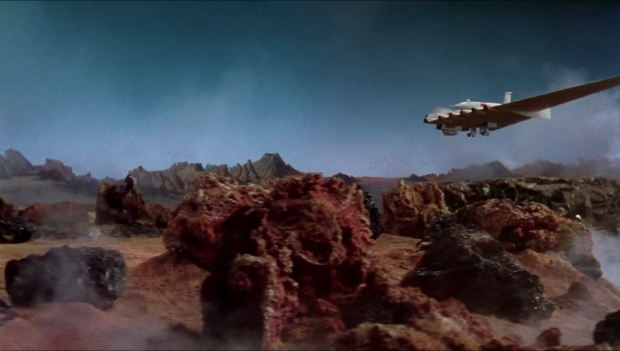
FIGURE 10. Spaceship One flies over a boulder-strewn Martian surface as it makes a perilous first landing on the Red Planet.
Thankfully for the crew, the ship skids into an ocher-colored boulder-free patch of ground and slides along this natural landing strip. The landing is still a rough one, however, as we see the crew being jolted around – Barney in particular, who precariously hangs on to the ladder, having no time to get back into his couch.
The ship keeps moving across the surface until its momentum is finally stopped by a cluster of dark boulders in its path. The crew has made it to the Red Planet alive, although rather shook up from their unplanned rough landing. Siegle looks over at Mahoney and sees that he has been knocked out by their rocky encounter with Mars.
Still sprawled across the ladder, Barney reaches down and shuts off the rest of the switches on his father’s control panel. He then addresses the crew.
“Hold your places until the ship is raised.”
Barney pushes himself off the horizontal ladder and gets back into his couch. He then activates the main ship to start ascending to the vertical position, which is indicated to the crew by a large deep red circular “screen” with concentric circles and a smaller solid disk representing the rocket that moves towards the center of the circles.
Outside we watch the rocket ship steadily rise into the vertical position. The wings are bent up but have served their purpose of getting the men to the planet’s surface in one piece. The disc reaches the center of the indicator screen and clicks into place.
“The ship is upright, sir,” reports Imoto.
Now vertical, the view pans down to the seats below the General and Captain, to where Mahoney and Siegle are temporarily ensconced.
“Good landing,” judges Mahoney.
“This is a good landing?” Siegle says in disbelief.
“Any landing’s a good landing,” Mahoney replies, meaning any vessel touchdown you can walk away from is a preferable to the alternative.
Siegle looks down the access tunnel running between the two men. Jackie decides to perform his own impromptu version of the famous experiment with falling bodies that Galileo Galilei (1564-1642) is said to have conducted off the Leaning Tower of Pisa in Italy: He takes out of his uniform pocket a small orange ball (a gumball? A marble?) and releases it from between his fingers. The little sphere immediately plunges downward and is heard hitting bottom a few seconds later.
“Gravity,” Siegle remarks. “Beautiful gravity!”
One level up, Barney is tending to his father, who remains lying in his couch.
“Let’s go below, shall we?” Barney says to Sam in a gentle tone. Barney heads down the ladder as Sam slowly begins to remove himself from his reclined position, saying nothing all the while.
Outside, the exterior ship hatch opens like a drawbridge with a mechanical whirring sound, while a long ladder automatically extends down the side next to it. In the background can be seen the silvery starboard wing extending towards the Martian horizon. This new setting is covered with large boulders of various colors and what seem to be patches of light fog. A deep blue-green sky completes the alien vista the men are about to explore.
LESSON TIME: Planetary scientists thought the Martian sky color during the day at its surface would be a deep blue due to the planet’s thin atmosphere. That idea would remain until 1976, when Viking 1 landed on Mars and returned the first color images of its surroundings: The planet’s daytime sky hue turned out to be more of a salmon pink, due to the scattering of surface dust in the Martian air.
Mahoney is the first to move out onto the horizontal hatch as the other men follow. Barney steps onto the ladder and begins to descend it towards the surface, with Imoto right behind him.
Siegle marches out onto the hatch and lunges his arms upward in an expression of excitement and wonder. Mahoney grabs him by the back of his spacesuit and points towards the ladder, as if he thought Siegle might step off the hatch instead. Siegle gives a flap of his arms as if to say okay, I will go down that way then, and gets onto the ladder.
COMMENT 1: These extravehicular suits are different from the ones we have seen elsewhere in Conquest. They are colored blue instead of white, except for the white oxygen tanks, and do not seem to be pressurized, or perhaps only enough so the astronauts can breathe on Mars and not freeze from the generally cold temperatures.
As astronomers would learn just one decade later, thanks to automated deep space probes, the atmosphere of Mars is not quite as bountiful as displayed in this film. The air is also composed mainly of carbon dioxide rather than nitrogen as thought at the time. Not that it would have been breathable for a human in either case.
COMMENT 2: These blue suits possess nothing external to distinguish the crewmen from each other. Granted, their helmet faceplates were wide and clear to help distinguish faces; however, this did not work so well when the men were at a certain distance from each other. The Apollo astronauts and others found the need for distinguishing suit marks or patterns when examining mission photos to determine which astronauts were who while on the Moon: Starting with Apollo 13, each commander had a red stripe on their white spacesuit’s helmet, arms, and legs.
In Destination Moon, the four astronauts each had outfits in different primary colors, excluding their helmets. This pattern was copied in tribute in 2001: A Space Odyssey with the spacesuits for the crew of the USS Discovery and the action figures for Mattel’s Major Matt Mason lunar explorers.
Captain Barney Merritt becomes the first person to step on the planet Mars. However, unlike what would take place in our reality in 1969 via Apollo 11, Barney makes no famous declaration upon touching the planet with his boots, or any verbalized comment for that matter. Neither does he take the time to plant a flag. Once down the ladder, Barney simply walks away from the ship, surveying the scenery with only his eyes for the moment.
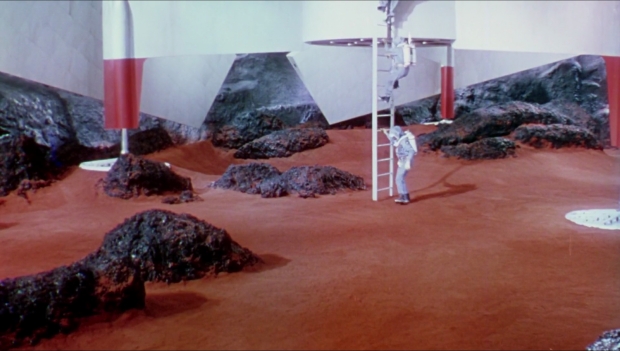
FIGURE 11. Captain Barney Merritt becomes the first human to set foot on Mars, but without any fanfare whatsoever.
COMMENT: Even in Destination Moon, a dedication speech is made by the first two crewmen once they have stepped on the lunar surface:
Jim Barnes: “Claim it, Doc! I’m your witness – claim it officially.”
Dr. Charles Cargraves: “By the grace of God, and the name of the United States of America, I take possession of this planet [!] on behalf of, and for the benefit of, all mankind.”
While the Apollo 11 astronauts could not claim the Moon or any other celestial body for their home country due to the parameters of the United Nations Outer Space Treaty of 1967, the designers of the dedication plaque, bolted to the laddered landing leg of their Lunar Module christened Eagle, appear to have been influenced by those spoken words in Destination Moon:
Here men from the planet Earth first set foot upon the Moon / July 1969 A.D. / We came in peace for all mankind
You may watch and listen to the landing crew of Apollo 11 describe and read their dedication plaque to the residents of Earth during their lunar mission here:
https://www.youtube.com/watch?v=9-skkXqZdE0
Imoto, Siegle, and Mahoney stand together next to Captain Merritt and join their leader in simply looking about the alien scenery in silence. General Merritt is conspicuously absent, but no one seems to be noticing his lack of presence.
Imoto decides to walk over to a nearby patch of ground. Kneeling, the Sergeant removes the right glove of his suit (!) and digs his bare fingers into the rust-colored minerals. Imoto scoops up a handful of Mars and brings it over to his comrades.
“Feels like good soil,” says Imoto, showing Barney his sample. The other two men gather around to see it as well.
“Given water, I bet you can grow anything here we grow on Earth. Big things, maybe, like in Jack the Beanstalk. Be wonderful to try and see what could happen.”
Imoto shakes the Martian soil from his hand, then reaches for something in his suit pocket.
“I have some flower seed,” Imoto declares, showing them the small white packet. “I been carrying them ever since I left Hawaii. I was gonna try them on the Moon, but here in this soil…”
“You’d need a pipeline from the Colorado River to water them,” interrupts Mahoney.
COMMENT: Not to disparage Imoto’s knowledge of geology, but I think the explorers would need to conduct more than a “feeling” to see if the Martian soil could indeed support life, particularly organisms from a completely different world such as Earth. That Imoto was going to try to grow them on the Moon is even more remarkable. Plants have been grown in lunar regolith brought back by the Apollo missions, but only in terrestrial conditions. There also seem to be no issues among the astronauts with Imoto introducing an Earthly life form to a world they have hardly even begun to examine.
Imoto swivels back to Barney, then suddenly notices something behind them.
“Captain, look!” Everyone turns where Imoto is pointing.
Water is pouring out of the base of the rocket onto the ground! The men run up to where the water is splattering all over the Martian surface.
“Must have damaged the pipes when we landed,” suggests Barney to the group. “Wait here.”
Barney rushes up the ladder by himself until he reaches the hatch level. Repeatedly he presses the hull button that opens the hatch, then climbs inside the ship before the hatch can even open fully. Once inside, Barney activates a button at the top of a black control panel along the wall next to the opening, closing up the exterior hatch behind him. He then presses another button that causes the hatch covering the access tunnel to the rocket engine section to rise open.
As Barney begins to strip out of his blue protective suit, he peers down the now open access tunnel – and discovers his father was responsible for turning open the large wheel valve that is causing the vessel’s precious water supply to spill out!
“General, stop!” Barney shouts at his father.
Sam stares up at his son with a wild look and begins to quote a Bible verse while brandishing a .45 caliber semiautomatic pistol in Barney’s direction!
“As wax melteth before the fire, so let the wicked perish at the presence of God!”
COMMENT: This passage is from Psalm 68. Biblical scholars believe it is part of a celebratory expression regarding the arrival of the Ark of the Covenant into Jerusalem (yes, that Ark of the Covenant, oh Raiders of the Lost Ark fans). The author was also praising and thanking God for letting His faithful servants be victorious over their enemies, which is undoubtedly what Sam Merritt had in mind when espousing this quote to his son.
“Father, stop it!” Barney yells frantically at his father, who is now turning another large valve wheel. “You’re mad! Hydrazine and nitric acid explode on contact! We’ll be blown to bits!”
ROCKETRY LESSON: The following is a quote from the space resource Encyclopedia Astronautica regarding the chemicals Barney tried to warn Sam about:
http://www.astronautix.com/n/nitricacid.html
“Drawing on the German World War II Wasserfall [V-2] rocket, nitric acid (HNO3) became the early storable oxidizer of choice for missiles and upper stages of the 1950s. To overcome various problems with its use, it was necessary to combine the nitric acid with N2O4 and passivation compounds. These formulae were considered extremely secret at the time. By the late 1950s it was apparent that N2O4 by itself was a better oxidizer. Therefore, nitric acid was almost entirely replaced by pure N2O4 in storable liquid fuel rocket engines developed after 1960.”
The chemical compound N2O4 is also known as dinitrogen tetroxide, more commonly referred to as nitrogen tetroxide (NTO) and sometimes amyl. N2O4 spontaneously reacts (meaning it is hypergolic) with hydrazine. These two compounds have become a common pair in modern rocket propellants.
Barney continues to plead with his father to stop, but Sam only warns him to “stay back” before firing several shots from his pistol at Barney, who ducks down out of the hatch entrance. The men who are outside, still standing around the flowing water, hear the gunfire. Mahoney immediately runs up to the ladder and ascends it toward the loud and frightening noises.
Laying on the deck just off the edge of the hatch entrance for protection, Barney calls down to his father.
“Dad, it’s Barney. Please, you’ve got to listen to me!”
Sam just looks upward while continuing to turn that valve. Realizing he must take action, as Sam has lost any final semblance of reasoning, Barney risks his life and starts climbing down the access tunnel ladder while continuing to beg his father to stop his sabotage.
“Don’t turn that valve! Don’t turn it! Father, please!”
At the base of the tunnel, Barney jumps down from the last few rungs and wrestles Sam to the deck before he can fire another shot. Then Barney leaps up and starts to turn off the valve.
Still on the deck, Sam sits up and lets off another round at his son: The bullet hits Barney in his left shoulder. Although bleeding and in pain, Barney goes after his father again, this time to get the weapon out his hand.
As the two officers struggle, Sergeant Mahoney arrives on the scene and immediately finishes turning off the wheel valve. Suddenly, there is a single gunshot. Mahoney turns to the men virtually at his feet.
Barney watches his father slowly sink back onto the deck: As they had fought over control of the pistol, the weapon went off and fatally hit Sam.
“Don’t blame yourself,” Sam says gently to his son. Then the General’s head tilts to one side and he is gone.
Before Barney can react, Mahoney violently grabs the Captain by the shoulder he has just been shot in and whips Barney around.
“You killed him!” Mahoney accuses Barney. “Your own father. You murdered him!”
Barney tries to protest, but the Irish Sergeant pulls Barney up to his feet and yanks Captain Merritt by the collar right into his face.
“Why, you no-good…” Mahoney seems ready to pummel Barney – or worse – then slowly lowers his right fist.
“No,” Mahoney begins. “No, Captain. You’ll not die, not now. But when we get back, and we’ll get back, we’ve got a date, you and me, with a court martial. And I’II be the witness.”
COMMENT: Assuming you aren’t given a court martial too, Mahoney, for disobeying a direct order from a superior officer and for being an unauthorized passenger on a vitally important and historic space mission, endangering both the success of the expedition and the lives of the whole crew in the process!
Bleeding from his left shoulder, Barney slowly turns away from Mahoney as he rants on and stares at the prone body of his father.
“And it’ll be a rope for you, Captain. And I hope they make it slow, very slow, so I can watch you kick!”
Mahoney moves away from Barney and down to the body of his friend. Barney begins to quietly sob in reaction to these traumatic events. The scene fades.
In the Corner Pocket of Nowhere
On the surface of Mars there appears something never seen before: A large and somewhat roughly designed metal cross, likely made from salvaged pieces of the ship’s wings.
Barney is standing in front of this cross, looking solemn. Next to him is Mahoney, glaring at Barney through his suit’s clear faceplate. Without a word, Barney slowly walks down and away from the small hill supporting the cross that is now his father’s final resting place.
Near the rocketship ladder, Imoto is busy scooping up some soil into his cupped bare hands. He walks up to Samuel Merritt’s grave site just as Mahoney is stepping down the inclined ground.
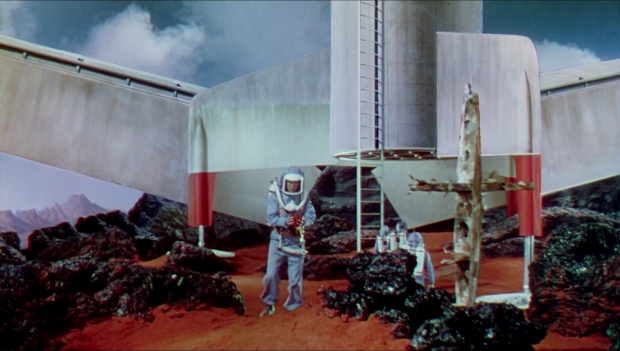
FIGURE 12. Sergeant Imoto brings a special experiment towards the final resting place of General Samuel T. Merritt.
On the hill, Imoto digs a small hole, then adds the soil he carried from the ship’s landing site. The Sergeant takes out his seed packet (simply labeled FLOWER SEED) and rips open the paper package. Removing a few seeds, Imoto pushes them into the deep reddish dirt with his finger, then covers them over with more soil.
Later on, we see Mahoney walking among some boulders on another hill with the ship in the distance. The Sergeant is toting a large white bag and a shovel. Wearily, he drops the bag on the ground.
Just below Mahoney in a natural depression is Siegle, who is busy using a tall drill, manually turning it to dig deep into the surface. The Sergeant is surrounded by several shovels and a scattering of white pipes of various sizes. The longer pipes are propped up against a large black boulder next to him.
Siegle looks up at Mahoney standing above him. Jackie grabs and presents a handful of soil (wearing his gloves, please note) and begins to address Mahoney on his findings in his own rough if succinct style.
“No signs of water,” Siegle begins his report. “Not even bugs or worms. They ought to give this planet back to the…”
Siegle pauses in thought upon realizing what he is about to say.
“Who do you give it back to? No people.” Siegle throws up his hands in exasperation and continues drilling for any water that might be in the Red Planet’s crust.
Later, we find the men gathered around Barney Merritt at the base of the rocketship.
“What water we have left is our life,” Barney declares matter-of-factly. “Every drop must be rationed. No washing, no heat.”
“Look, Captain,” pipes up Siegle. “Why couldn’t we kick off with what we got left?”
“We could, except for one slight detail,” answers Barney. “The Earth wouldn’t be there when we arrived.”
“How very unpleasant,” Siegle intones. “So how long we gotta stick around this crummy planet?”
“How old are you, Sergeant Siegle?”
“Just turned 29.”
“When you turn again, you can start packing.”
FASHION STATEMENT: In 1955, actor Phil Foster was 42 years old. With all due apologies, Siegle doesn’t look close to 29, even if one uses the excuse that young people in earlier eras tended to look a bit older than their actual ages due to hair and makeup styles, mannerisms, and dress.
Barney gets back to the business of their surviving on Mars for the next year.
“You and Sergeant Mahoney start draining the pipes of the heating system,” Captain Merritt orders, then heads up the ship’s ladder.
“Well, he says we gotta squeeze the water,” Siegle opines to the rest of the crew. “So, let’s get to squeezing. It’s gonna be a cold, dry winter.”
A Christmas Miracle
Despite their situation, or perhaps because of it, the remaining men of Spaceship One are making an effort to celebrate the Christmas holiday while stuck on Mars. A 24-hour face clock along the wall showing the date of December 25 is encircled with a makeshift wreath. A wool sock with a few meager items inserted into it hangs along one edge of the timepiece.
Mahoney, his head adorned with a green winter hat with ear flaps, is singing a slightly modified version of the first four lines of the traditional Christmas tune “God Rest You Merry, Gentlemen” as he and Imoto toast each other with small cups of liquid water. Sergeant Siegle, covered in a dark green blanket draped over his shoulders like a cloak, accompanies Mahoney on a harmonica as he sits at the radio console. Barney merely stares out at Mars through the navigation dome. All the men are wearing an extra pair of light-colored warm clothing under their blue uniforms, for it happens to be winter on Mars as well.
COMMENT 1: Yes, I know in Earth’s southern hemisphere it is the summer season during Christmastime. However, you also know by now that Conquest was made in 1955 by a bunch of Americans and Westerners, so they are going to equate Christmas with winter. It is also a fact that Mars has seasons like our world, only they last twice as long since it takes the Red Planet 687 Earth days, or almost two years, to circle Sol just once.
COMMENT 2: Among the many small and large parallels between Destination Moon and Conquest, both men from Brooklyn happen to bring a harmonica with them on their space journeys. Was this the musical instrument of choice for folks from New York in the Twentieth Century, or was it just easier to carry around than, say, a baby grand piano?
“God rest ye merry gentlemen / Let nothing you dismay / Remember Christ our Savior / Was born on Christmas Day.”
“Call the Wheel again, Sergeant,” Barney orders Siegle, but with little enthusiasm beyond a sense of duty.
“What for?” inquires Siegle, lowering his harmonica. “To wish them a Merry Christmas?”
Realizing he has been given an order, Siegle tries contacting the space station circling distant Earth.
“Spaceship to Wheel. Come in, Wheel. Over.”
Siegle clicks off the radio switch in frustration.
“How long you gonna keep doing this?” Siegle asks impatiently, his five o’clock shadow fairly prominent on his dirty face. “We ain’t heard from the Wheel in months. And supposing they answer, so what? Maybe we ask for room service… and have them send up some ice water.”
Barney swings around to face Siegle. There is a combination of anger and conviction in his voice. His face reveals some definite strain from the past months. Behind him through the dome stand a chain of Martian mountains.
“We’ve done what no men in the world have done before us. We’ve got to let them know before it’s too late. If it’s humanly possible, we’ve got to report.”
“Report what?” Siegle counters. “That the operation was a big success, but the patients are dying on a lousy, dried-up ball in the corner pocket of nowhere?”
“We could report a murder, Captain,” interjects Mahoney, always knowing how to make an already bad situation a bit worse.
Barney looks up at Siegle defiantly.
“I’ve told you everything that happened, Sergeant.”
“Yes, Captain… that you have,” answers Mahoney, walking up to Barney. “And you can tell it to me from now until Doomsday, but don’t forget, I was there and I saw everything with me own eyes.”
Barney doesn’t bother to respond to Mahoney or even continue to stare at him. Quietly, Siegle gets up from his post and walks over to the ladder. Imoto takes his friend’s place at the radio. Mahoney stares out the dome.
“Spaceship calling the Wheel. Spaceship to Wheel. Over,” relays Imoto into the radio.
Mahoney suddenly slams his plastic cup to the deck in an outburst of anger. He then adds further fuel to the fire of discontent with superstitious babbling out of fear…
“The General wasn’t crazy, he was right,” Mahoney starts. “We asked for it. There’s a curse on this ship and everybody in it!”
“Baloney,” answers Siegle. Mahoney turns towards Jackie in surprise.
“You can leave that stuff back on Earth…” demands Siegle, but without even raising his voice. “But it don’t operate past a thousand-mile limit.”
The camera then focuses on Siegle as he expands his spiel to the rest of the crew.
“Only God can make a tree. Okay. Where is it? Where’s the trees and the flowers and the grass? Where’s the water? You hear me? Where’s the water?!” Siegle shouts his last sentence for emphasis before sitting down, clearly disheartened.
As Siegle gives his rant of frustration, Imoto has been staring out the dome. His eyes grow wide as he sees something surprising outside the ship and excitedly alerts the other men as he points with a gloved finger.
“Hey, fellas, look!”
The crew rush to the dome. A flock of small white flakes are falling across their view.
“Snow!” yells Mahoney.
“Boy, you can hit me with a snowball!” shouts Imoto with joy.
Someone declares “Merry Christmas” with heart this time as Barney orders them to open the hatch.
Our view moves outside where we see Spaceship One and the ground around it covered in life-saving snow as the instrumental version of “God Rest You Merry, Gentlemen” plays in the background. All four men are working at the base of the rocket: Barney and Mahoney are pulling down a long flexible tube from the ship to the surface, while two other gentlemen have taken the time to engage in a brief snowball fight! Barney signals them over to help with the tube while he climbs back up the ladder into the vessel.
Back in the ship, Barney is standing at some controls.
“Okay, Captain, let her rip,” shouts Siegle. Barney activates the tube’s suction function.
The other three men are holding onto the long tube to vacuum up the Martian snow via a small funnel at its one end into the ship to replenish their water supply. Barney watches their progress via a large manometer, a curved glass tube containing a red liquid that measures the change in pressure in the water tank as the precious snow is added to it.
As the liquid steadily moves from one side of the tube to the other, Barney puts his head into his hands from a sense of relief coming over him that they and the mission now have a real chance of being saved.
A Flower on Mars
The 24-hour clock is shown again. The adorning Christmas decorations are long gone, as the date on the clock’s face displays July 8. The red second hand slowly and steadily sweeps its arc.
The scene switches to an external view of the rocketship on Mars. The red-striped ship itself is still standing erect, with the wings flanked on either side. Trailing into the distance, the landing gear skid marks are still present in the reddish Martian soil.
The four men are busy at the base of the ship preparing for the long trip home. Siegle and Mahoney are rolling up a collection of large, ridged cylinders with a red cap at each end when Captain Barney Merritt stops them in mid-roll.
“Leave that, Mahoney,” orders Barney. “We won’t take anything unessential to the voyage.”
Sergeant Imoto, who is sitting nearby, asks his Captain a question regarding the ship.
“Everything okay up there, sir?”
“All secure,” answers Barney with a confident smile. “By this time tomorrow, we’ll be on our way home.”
Barney strolls over to Imoto to see what he is working on. Imoto picks up a light colored stone labeled with an identification number (147) from a cluster of various surface samples at his feet.
“Just about finished, Captain,” Imoto reports. “I think these soil and mineral samples will prove that life is possible on Mars. It can be done, sir. AII the elements are in those sacks.” The view switches to a group of small white tagged bags surrounded by several collected Martian rocks. “Even air and water, in other forms.”
Imoto goes into a brief soliloquy about Mars.
“Until now, this little planet has been alone… friendless, all drawn up into itself. So, it’s crusty, dried-up, and unyielding. But with patience and understanding and hard work, it could be made to blossom.”
“I wish I had your faith, Imoto,” says Barney with sincerity, before walking away to conduct another preparation task.
COMMENT: At least Imoto got some surface samples to bring home for detailed examination, thanks to the geologist’s dedication and expertise. In Destination Moon, that crew wasn’t able to retrieve so much as a lunar pebble, at least not intentionally. They did have an excuse, however, as the men of Luna had to shed every unnecessary ounce of weight from their ship in order to have enough fuel to leave the Moon and return to Earth (they had used more rocket fuel than planned when landing on the Moon).
The scene switches to the place where Imoto had planted his flower seeds on Sam Merritt’s grave. Emerging from the soil, which is surrounded by a protective marking ring of small black stones, we see… a short stem and two green leaves!
Imoto, who is standing over this incredible sight, calls out to his comrades nearby.
“Hey, fellas! Come!” Imoto shouts, waving his arm excitedly. “Captain, come! Look! Look! Look!”
The men run up the brief hill to Imoto.
“It grew,” the Sergeant declares. “A flower. A seed from Earth growing here. Look at it. A flower on Mars!” Imoto drops to his knees in wonder and delight. The others lean in closer to witness this small miracle.
“It is a flower,” exclaims Siegle as Imoto pours a container of water on the plant.
The crew of Spaceship One are given no further time to let this event sink in as they watch in surprise as several boulders on a nearby hillside start to tumble from their resting places. These boulders are quickly joined by a literal avalanche of rocks around them, as the ground begins to heave. A Marsquake!
All four men are knocked to the ground from this seismic jolt, their blue suits becoming covered in red Mars dust. The makeshift metal cross marking Sam’s grave lilts to one side. The ship is affected as well: The rocket is angled off its center but has not toppled over – yet.
“Hey, Captain, the ship is tilted!” yells Imoto. “We won’t be able to take off.”
A large sinkhole appears in the nearby ground due to the quake, followed by three more such holes in succession in a chain reaction. With each opening, the sands of the Martian surface start falling into them.
“That sand, look at it,” declares Barney. “It’s draining. Must be caves or crevices underneath.”
“This whole section is honeycombed. We drilled into a lot of pockets.” Imoto informs his Captain.
“Caves, caverns,” thinks Barney, hatching a plan. “If we could crack them open, the sand would pour in like water. We might draw off enough to lower that side of the ship.”
“Straighten her up?” asks Siegle.
“It’s possible. But how do we crack open the cavities?” asks Imoto.
“With the engines,” answers Barney, looking back at the rocket nozzles. “We blast. Hammer the ground with the rockets.”
“Great. But what happens if we open some cracks on the wrong side? We fall over,” points out Siegle.
Barney looks at his wristwatch.
“We’ve got exactly fourteen minutes to come up with a miracle and say goodbye to this planet, or we’ll never leave it!” Barney taps Siegle on the arm and quickly heads up the ship ladder.
Siegle turns to look at Imoto.
“You were saying, Sergeant?” Imoto rhetorically asks Jackie Siegle.
“I’m sold!” answers Siegle and they run after Barney. Mahoney follows last almost as quickly.
Once inside the ship control room, Siegle and Barney monitor the angle of their vessel with that red circular indicator we witnessed when the rocket was being raised up after landing on Mars. The disc representing the ship is over halfway from the center circle.
“Punch it, Captain!” Siegle says firmly.
Barney pushes several switches and levers. The rocket begins to blast, lifting off the ground a bit from the force of the bright white engine firing. Glowing blast sparks litter the surface.
The indicator disc has barely moved, if at all.
“Again, sir,” says Imoto.
Barney keeps blasting the ship’s engines. Suddenly, part of the ground beneath the rocket gives way, falling into a deep cavern as they had hoped.
Barney throttles the rocket engines again, causing even more of the surface to collapse into the cavern. This time one of the landing legs is affected, which starts to move as its natural platform gives way.
The indicator disc starts moving towards the center!
Barney keeps hitting the thrusters on and off. Even more ground collapses beneath them, although sharp-eyes viewers will note that the filmmakers reused the same footage we just saw of the ground collapsing into the cavern. This time they cut away from the scene just before the landing leg footpad starts shifting.
Barney stops throttling the engines. The indicator disc also stops moving towards the center.
“Try it again, Captain,” requests Imoto.
“That’s it. I don’t dare to…” Barney begins to respond. Just then, the ship shifts some more as the ground gives way on its own. The indicator disc moves right into the center of the circles!
“We’re perpendicular!” shouts Siegle with joy.
“Protect yourselves! We’re blasting off!” Barney orders his men, who run towards their protective couches.
Barney hits the thrusters at full throttle. The ship finally begins to lift off the surface.
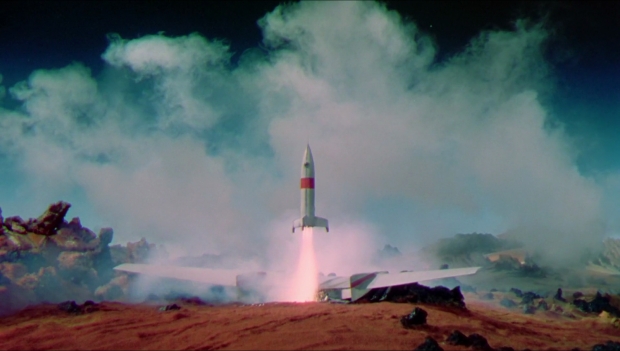
FIGURE 13. After many moments of peril, Spaceship One finally blasts off from the surface of Mars!
The crew, unable to reach their seats in time, are slammed to the deck by the resulting g-forces as the rocket blasts into the sky. Barney is pinned against the control panel. We see blood on the faces of Imoto and Siegle!
As the rocket plows upward, Barney is just able to withstand the forces on his body long enough to shut down the engines as they enter space.
Mahoney is the first one to get up off the deck. He pulls himself up towards the Captain. Imoto and Siegle pick themselves off the deck next. With blood framing his mouth, Mahoney stares at Barney, who is slowly getting up from the control panel and sporting his own bloody wound, a cut around his right eye.
“You did it,” Mahoney declares to Barney.
“A date is a date, Sergeant,” is Barney’s weary reply to Mahoney.
Siegle sits at the viewscreen station and turns on the monitor. We see the globe of Mars receding into the distance against a star-filled background.
Mahoney and Barney look at each other. Mahoney stands up fully, then walks over to a wall, facing away from the rest of the crew. Barney stares after him, wondering what his reaction is going to be.
Mahoney finally turns back and stands behind the rest of the men at the control panels. He begins to speak to them all without looking at any one in particular.
“It was a glorious way the General died,” Mahoney begins. The crew look at him in astonishment. Mahoney continues, turning from one man to the next almost as if they were going to defy what he is saying now.
“Sacrificing his life as he did to bring his ship and his crew safely to a landing on the rocky desert of a new planet. That’s the way the history books will tell it. Won’t they, Captain?” Mahoney says this last sentence right into Barney’s face. “A fitting end for a grand soldier.”
“For the man who conquered space,” Barney adds to Mahoney’s little speech. Then he gently nods at Mahoney to show that he agrees with this interpretation of his father.
Mahoney leans forward and puts his arm around Barney.
“Would you be caring for a cup of tea, Captain?” asks Mahoney, as he once did for Sam.
Barney smiles and nods.
“Thanks.”
What is left of Spaceship One is seen heading off into the void back to Earth. Only then, at the end, do our main actors get their due cast credits.
My Personal Take on Conquest
“The Conquest of Space [sic] is by no means a towering cinematic masterpiece, but it is a minor classic of its genre, and a neglected one.”
• Kinnard, Roy. “A New Look at an Old Classic: Conquest of Space” in Fantastic Films: The Magazine of Fantasy & Science Fiction in the Cinema, Volume 2, Number 2. Chicago: Blake Publishing Corporation, June 1979.
Before I begin my commentaries on the various aspects of Conquest of Space as they relate to how we once planned and still will plan to “conquer” the issues of permanently settling the Final Frontier, I want to give my overall review as a film of this now minor classic of the science fiction genre. As you might imagine, I have a number of things to say regarding this George Pal et al creation.
As I stated at the beginning of this essay, I was not nearly as familiar with Conquest as I had been for the other films I wrote about for Centauri Dreams. Conquest is seldom shown on television compared to genuine classics like Forbidden Planet and 2001: A Space Odyssey. The reviews of the film were often lukewarm at best and otherwise downright dismissive. It was hard to find anyone truly recommending Conquest except as part of fulfilling some completion list.
When I did finally see the film roughly one decade ago, my first encounter with Conquest was less than favorable: The mission plans seemed written by people who knew little about space exploration despite basing the film on a popular and technically accurate book on the subject. A manned mission to the Moon suddenly changed to Mars, with the person in charge of the expedition not seeming to pick up on all the blatant evidence for this major redirection of plans!
A multitude of other details of their depictions of living and working in space only served to further color my take on this film, but switching from a mission meant to go from near Earth to many millions of miles across the Sol system on a dime should have been seen as patently absurd, to say nothing of reckless, by even a child.
This is the kind of practical and technical nonsense one expects from the many lesser science fiction offerings that became so prevalent in the 1950s, where low budgets were matched with poor scripts filled with scientific howlers. A prime example comes from Rocketship X-M, which was rushed to release by Lippert Pictures to beat Destination Moon to the cinemas. The plot concerned a crewed space expedition meant for Earth’s satellite that ends up at the Red Planet after a series of natural and technical problems. One of the mission astronauts goes so far as to essentially say that God was the reason for all these events happening.
An even less appetizing feature of Conquest was its main characters. When I decide to delve into an entertainment production, how the characters are presented make or break a story for me, with only a few notable exceptions. Conquest seemed to be going out of its way to check off every stereotype it could muster, apparently in the name of making this “wild” vision of human beings among the stars more palatable to contemporary audiences.
Samuel T. Merritt
Colonel (later promoted to General) Samuel T. Merritt was meant to be the stalwart leader of this first manned flight to the Moon. He was also designed to represent how one should be when it comes to living in space and overseeing others who are part of this grand new experiment for our species.
Instead, right off the bat, we meet a man who chews out some lower ranking corporal who merely expressed the desire that a cargo ship approaching the space station they were on have a supply of ice cream on board. That we the audience would later become aware why Colonel Merritt responded as he did over such a seemingly innocuous incident does little to make him endearing. This situation does not improve when in the next scene, we watch Sam bluntly shut down his own son, Captain Barney Merritt, who requests a transfer off the Wheel so he can be with his wife, whom he has not seen in “a full year” and had to leave for Earth orbit just over three months into their marriage.
I get that Sam Merritt is supposed to portray the “tough but fair” type of leading officer expected from what is a military culture, but we are not given much to latch on to emotionally to care enough as to why he is so rough on those under his command, not to mention his own blood offspring. Even later revelations that Sam is one of the key people who made the Wheel and Spaceship One possible, along with the fact that he has been a career soldier for decades, are not enough to turn our sympathies.
When Sam later has an emotional breakdown – after we witness him personally removing a man from duty with far lesser ailments than he – and twice jeopardizes the entire expedition of the first human mission to Mars, which includes his only son, the best one can muster for General Merritt is a measure of generic pity.
As one might imagine, if the main character is neither sympathetic nor interesting enough to care about – note that caring does not necessarily have to mean they are automatically a good person; they can also simply possess enough of certain characteristics to make an audience want to continue watching them – then at least some of the other plot characters will have to pick up the slack in this department.
Unfortunately for Conquest, the writing is not sophisticated enough to give us any really compelling characters to truly root for, at least in a more complex manner. This is especially unhelpful in a story that many if not most Earth raised and bound viewers cannot deeply relate to. Nevertheless, I will present here their personal gems whenever I can find them.
Barney Merritt
Sam’s son Barney is a fine person as human beings go. He is relatable in that most would want his traditional good looks, commanding presence, and privileged life and career positions. Barney is also a bit “vanilla”; however, that is mainly the fault of the writers who wanted a typical Everyman in his role as seen from 1950s Western values.
I will add that Barney is rather remarkable in how he stands up to all of the challenges facing him, particularly when he has to deal with his deranged father in both life and tragic death, and then endures months of hostility and false accusations from a crewman who never should have been on that Mars mission to begin with – all while trapped on an alien planet far from home with a group of men who are counting on their Captain for survival.
As a bonus, while Barney faces his challenges as stoically as he can, he is not an unfeeling automaton: He weeps for his father’s death even after Sam had just tried to kill own son and sabotage the mission. Barney also misses his wife and their planned life enough that he is willing to give up a very promising career with the Space Corps which his father declared was his “heritage.”
Donkersgoed and Sanella
Most of the other main cast members – with three notable exceptions who will be more thoroughly examined – are sadly lacking in any detailed backgrounds or personalities beyond a few key traits. Of the men assigned to the initial lunar mission, Roy Cooper and Andre Fodor mainly stand out in large part because they are given more than a few lines and actions.
Pete Donkersgoed and Pedro Sanella are barely memorable and add little to the overall story. They are supposed to share in highlighting the international flavor of the Space Corp, but it is hard to say how much that attempt worked, even for the film’s initial audiences. As you may recall, the biggest interest that Donkersgoed stirred for me was to learn if his unusual last name was either based on reality or composed for the film. Their mildly “foreign” traits, namely their accents, seem to exist to add a bit of humor to the story when called upon.
Cooper and Fodor
While Cooper and Fodor are both more enduring characters than most of their crewmates, this is not due to any deep dives into their personal histories or other traits. Cooper serves mainly as the token example of what happens to any of the astronauts who are unable to handle the performances and pressures then considered to be required of these men to deal with the rigors and unknowns of space travel.
As for Fodor, we know he is a doctor who had “two years of medicine in Vienna.” He seems to be either working with or for the other introduced medical doctor on the Wheel, one Major Kurt Elsbach – yet another character for whom we have scant background, except perhaps that his smoking preference is a pipe, one of the chief signs of an intellectual type in those days. Fodor has a personal relationship with Cooper that includes multiple outward examples of concern for his welfare. The man also has a devoted mother who declares that Fodor is a “good boy.”
The only other items that make Fodor memorable in Conquest, if we are being honest here, are his unexpected and dramatic death and subsequent space funeral during the interplanetary phase of their mission to Mars. The final touch of irony is that other than seeing Andre’s screaming face when he is struck by that hurtling meteoroid, Fodor’s last scenes represent the man only as an inert body and then only in his spacesuit.
Sergeant Imoto
In another film of the era, a character like Sergeant Imoto might exist solely to represent a member of his ethnicity with a few badly stereotyped traits thrown in either out of audience expectations or for comedic effect, or a mixture of both.
While Imoto does stand out in Conquest of Space as the only non-white main character in the film cast, it is to the credit largely of Producer Pal, I presume, that Imoto is more than just his Japanese ancestry or his profession. That infamous speech about why Japan became involved in World War 2 aside, Imoto’s knowledge as a geologist on a mission to determine if humanity can utilize the natural resources of Mars for the continued existence of civilization is vital and key. More than this, Imoto demonstrates a level of professionalism and psychological stability throughout the film that most of the other main characters often seem to lack. It is safe to say that the mission to Mars might not have been as successful, or perhaps even failed outright, without the presence of Sergeant Imoto.
Imoto is more than just a professional astronaut and geologist: He is optimistic, possesses a definite passion for wanting to be on this Mars mission, is faithful to his duties and his companions, and shows a sense of humor. Imoto also shares a genuine friendship and camaraderie with Jackie Siegle: This is demonstrated throughout the film with Imoto often beginning a commentary on a situation with the quip: “You were saying, Sergeant Brooklyn?”
SIDE FACT: In Japanese, imoto (also spelled imouto) means “younger sister”. A person uses this term when talking about their sibling to someone who is not a member of their family. How and why this particular word may have been used for Sergeant Imoto escapes me.
Jackie Siegle
If you could poll those who have watched Conquest to vote on who is the most consistently annoying character in the film, the ballot winner would likely be Sergeant Jackie Siegle. He is a rather painful and dated stereotype of a resident from a certain borough of New York City designed to be the story’s comic relief and relatable figure.
Siegle is loud, brash, uncouth, inconsistent, self-contradictory, and deliberately ignorant of many crucial facts regarding outer space and the methods of traveling through it. I know that after my first few viewings of the film, Siegle was among my top reasons for disparaging of Conquest ever being anything more than a fading second-tier product of its time.
Now that I have conducted multiple viewings of Conquest for this essay, I have come to realize that while Sergeant Siegle is still all the descriptive terms I listed in the previous paragraph, he does have a number of positive traits that did bring forth humanizing elements to both the space mission and the film.
One of the more notable and noble traits of Sergeant Siegle is that he always stands up for his fellow crewmen. When Roy Cooper became paralyzed with fear on Spaceship One, it was Siegle who shouted for a space taxi to get the man back to the Wheel for medical attention. Soon after their return to the station, when Mahoney began aggressively nosing in on Cooper about his condition, it was Siegle who told the Irish Sergeant to back off, even though his warning was ultimately fruitless.
During their interplanetary voyage to Mars after Andre Fodor was killed and his body left to float outside the spaceship at the end of its tether, Siegle was the only one who became visibly and verbally upset at the fate of the man who had been with him on that repair job, while the rest of the crew stayed quiet and tried to pretend there wasn’t a corpse literally hovering over them like a ghost (enshrouded all in white, no less).
When General Merritt’s religious tirades went to a truly disturbing level, it was Sergeant Siegle who tried to alert his fellow crewmen about the potential danger to them all, only to be thwarted by Mahoney and his misplaced defense of his old friend.
When the crew had spent months stuck on Mars with dwindling supplies and just enough water to stay alive, Mahoney began a rant, mirroring Samuel Merritt’s earlier actions, that there was “a curse on this ship and everybody in it!” Almost surprisingly, it was Siegle who called Mahoney’s comments “baloney” and to “leave that stuff back on Earth… it don’t operate past a thousand-mile limit.”
As far as work was concerned, Siegle was not always the most initially enthusiastic member of the crew. More than once General Merritt called him out for not focusing on an assigned task and even got on his case about not properly wearing a pair of magnetic boots! However, when push came to shove, Siegle acquiesced to the tasks at hand and completed them, both on the Wheel and all the way to Mars.
So yeah, Jackie Siegle was a rough-edged character among other things, but he was also redeemable at several critical junctures of the plot. Whether other viewers had the patience and open mindedness to give Siegle a chance is another matter, though.
Sergeant Mahoney
If there was one character I could not find much redemption for even after multiple viewings, it was the Irish stereotype named Sergeant Mahoney. Not just because he was a dated caricature of someone from the Emerald Isle, but because his character was often a detriment to the mission due to Mahoney’s overblown and misplaced reactions to his friendship with Sam. Perhaps not as bad in one respect as Sam’s unhinged threats and actions, but his lack of professionalism and low manners caused enough problems for those around him.
Mahoney was placed in charge of monitoring the men who would fly first to the Moon, then later to Mars. He treated them like unruly children rather than the adults they were. As shown when we first meet the Irishman, his “bedside manner” with Roy Cooper left much to be desired.
For a man who has spent at least three decades in the military, Mahoney seems to think that established regulations and laws only apply to him when he feels like obeying them, in no small reason due to his long friendship with General Samuel T. Merritt. This led Mahoney to stow away on the spaceship to Mars: As I stated before, not only was this a serious violation of an expedition that the Supreme International Space Authority placed in its highest priority for the literal survival of humanity, Mahoney’s very presence aboard Spaceship One threatened to offset the entire mission.
The Sergeant’s aggressive and overbearing attitude during this Mars mission did more than just hurt morale. He threatened physical violence against Siegle when the latter tried to warn the crew about the very dangerous things General Merritt was threatening, even though Mahoney was well aware of his friend’s changed behavior.
Mahoney made things even worse when Sam was accidentally killed during his struggle with Barney to wrest away that weapon his father never should have brought onboard in the first place: Mahoney immediately accused Barney of murdering his own parent, adding how he can’t wait until they are back on Earth so he can be at Barney’s court martial to pay the ultimate price for his actions:
“And it’ll be a rope for you, Captain. And I hope they make it slow, very slow, so I can watch you kick!”
Mahoney pathologically never lets up on this accusation while they are stranded on Mars, not even on Christmas Day, not until they finally leave for home. Only then does the Sergeant have a change of heart, and this primarily due to the fact he didn’t want to see his old friend’s reputation be besmirched (nevertheless, the authorities back home had already heard Sam spouting some very disturbing things via the radio and one has to wonder if they really bought Barney’s cover excuses for his father’s words).
Mahoney’s final act is to transfer his feelings and affections from Sam to his son. Barney appears to agree with this transformation from Mahoney, perhaps in part out of a sense of relief for the moment. Undoubtedly the filmmakers wanted the audience to feel everything would be okay from then on and their mission and its goals would be a success. However, I was left with the unsettling feeling that Mahoney has simply replaced Sam with Barney now that his old friend is gone. I can even see Mahoney banking on this new relationship to get himself out of any trouble with the authorities upon their return to Earth from his stowing away against direct orders.
COMMENT: Pardon my cynicism here, but rewriting the history of what Sam Merritt did during the Mars mission would benefit the entire crew in case the authorities decided to pursue the question of why they let the General act as dangerously as he did for so long. His rant about blowing up the spaceship and destroying the plans for building any more was heard on Earth, or at least specifically the Wheel. Barney’s rather weak explanation for his father’s actions may not be enough for some, if we want to be at all plausible here.
Then there is the physical evidence of the bullet wound in Sam’s body (did they also leave in the bullet?). Some day the first landing site on Mars by humanity will be explored and if they encounter Sam’s remains, these future paleontologists may examine it and wonder why he was shot, leading to all sorts of questions and conclusions – although the original crew and those immediately involved with the mission will be long gone by then. I will discuss this possibility further later in this essay.
The makers of Conquest likely saw Sergeant Mahoney much like Sergeant Siegle, as a well-meaning if rough around the edges character whom contemporary general audience members could relate to. Unlike Siegle, however, Mahoney’s behavior just comes across as overbearing and downright disturbing at certain times, at least certainly to me. That he blatantly threatened others and an entire landmark space mission by his self-centered actions overwhelms any good intentions the filmmakers intended with Mahoney. His character often did as much to harm the film’s popularity as any of its flawed dialog, physics, and gaps in logic.
Now let us take a look at a deeper level of our “heroes” of Conquest of Space and how this relates to the wider issue of their depictions of humans living, working, exploring, and settling outer space…
Mother Mahoney: Family and Gender Roles in Conquest of Space
One aspect of Conquest noted by modern viewers is the lack of women who are seriously involved in this particular future vision of space utilization. When the female members of the human species are part of the plot, the roles they most often serve are either as someone’s mother/wife (Barney Merritt only mentions his wife and mother, while we see Andre Fodor’s doting madre, or mutter in Austrian, briefly on the big viewscreen) or an excuse to titillate the males in both the story and the audience (the dancing harem on the viewing screen followed by Jackie Siegle’s soon-to-be ex-girlfriend Rosie). There are no women physically present on either the Wheel or Spaceship One: The military cultured male staff see this as a normal state of things.
This ailment is hardly unique to Conquest, both in science fiction and real life. In Forbidden Planet, the only woman we see is Altaira, the young and beautiful daughter of Professor Morbius, who is almost immediately chased after by most of the all-male crew of the starship C-57D. In fact, in most genre cinema of the 1950s, if there were a woman or two as part of a spaceship crew, they were often relegated to being nurses or food servers for the men, even if their official ship position was as a scientist. In essence, they were caretakers and companions. If danger arose, as it always did, the women would turn to the big strong men in command for help and comfort.
This situation would continue into the next decade on its way to slowly improving. In the 1966 film Fantastic Voyage, the lone main woman character in the cast was almost kept from joining the otherwise all-male team of the nuclear submarine Proteus – including one Captain Bill Owens, who we Conquest fans recognize as Roy Cooper, played by actor William Redfield (1927-1976) – by a project general who insisted that “a woman has no place on a mission of this kind!” Even the landmark 2001: A Space Odyssey, released two years later, had no women in any substantial roles. With one brief exception, a Soviet radio astronomer who had just come from their observatory on the Moon, most of the women we did encounter aboard the film’s various space vessels were flight attendants, better known then as stewardesses. In addition, a young daughter and mother were seen and heard briefly on separate viewscreens.
On the smaller screen, the original Star Trek television series, which aired for three seasons from 1966 to 1969, became legendary for the social and cultural barriers it broke on contemporary media. However, even it could not escape certain aspects of its time: Women who served on the starship Enterprise and in Starfleet wore uniforms with very short skirts, along with fishnet stockings and knee-high leather boots. In the very last episode, it was revealed that women in this otherwise progressive future era could not serve as starship captains, with the only reason seeming to be their gender.
Returning to Conquest, it was interesting to see the directions certain characters took in the absence of women in their isolated societies. Although I am not certain how conscious the makers of this film were in regards to what I will be describing and discussing next, it does contribute to our understanding of how humans will behave and work in space both in settlements and expeditions – something we still have much to learn about despite over six decades of men and women being present in our Final Frontier.
Sergeant Mahoney, who anyone in the film would consider to be one of the more manly and generally tougher members of the crew, including himself, took on some roles traditionally held by women, both in a wider and more personal sense.
With the men who were going to the Moon (later Mars) under his charge, Mahoney acted like a mother hen. At one point the men actually called him Mother, albeit in a decidedly non-serious manner. Siegle even pinched Mahoney on the cheek in that same scene in a way a man might treat an older female family member. Again, this may have all been done in a playful way, but the underlying meaning is still relevant.
Mahoney referred to the men variably as helpless infants, incubator babies, and bluebirds, all diminutive creatures looked upon as being young and in need of care and protection; in other words, children. His children. Even the rest of the Wheel crew noticed this relationship, once calling these men of exploration “six little lambs” who belong to Mahoney. Siegle called the Irishman an “overgrown babysitter” after he hauled away Cooper to have him examined for potential weaknesses.
Another trait one might have assumed belonged to women in the era of Conquest was the habit Mahoney had of offering tea and coffee to others, especially when they seemed to need it as part of an emotional comforting. Mahoney did this for Sam Merritt after one of his anti-space religious tirades during the cruise to Mars: “Sam. How about coming down to the parlor and having a little cup of tea with a lonesome old friend?” Mahoney offers coffee in the same manner and intention after Fodor is killed by a meteoroid and his body hangs lifeless outside the ship in full view: “…if anybody’d like some hot coffee… I could heat up a couple of tins.” In one of the last acts we see on screen, Mahoney makes peace with Barney once they escape Mars for home by using “a cup of tea” to the Captain as an offering.
Pivoting to Sam and Barney Merritt regarding Mahoney, is he a substitute wife and mother to these men? Perhaps not literally, but his behavior and comments would suggest he has taken on those roles, whether the father and son ever asked Mahoney to or not.
Certainly, Mahoney’s devotion and loyalty to Sam goes beyond mere friendship to the point of obsession. He has followed Sam around for over thirty years, from when they served together during the Korean War to later joining him aboard the space station, against Sam’s specific orders, please note. Mahoney stowing away on the Mars mission, again disobeying a direct order from his superior officer and potentially endangering the entire expedition, should have been the final nail in the coffin under any other circumstances. Instead, Sam forgives his old friend after an initial outburst of anger and Mahoney “repays” his buddy’s leniency by enabling Sam’s degrading mental state to nearly the brink of getting everyone killed in the process.
Then there is the relationship between Roy Cooper and Andre Fodor. It is made quite clear that Fodor deeply cares about his friend, who is having a crisis of confidence due to the strains of preparing for his deep space mission. Some of Fodor’s affectionate behavior seems to be more than just friendly caring or professional concern, if Fodor is also a therapist as I speculated earlier. Am I, a resident of the Twenty-First Century, reading too much into this? Or am I seeing something that might have been missed by 1955 audiences? Older films did have deliberately hidden subtexts on subjects that would never have been allowed into the light otherwise. I am not certain that the writers of Conquest were in that particular frame of mind, at least consciously. Nevertheless, something seems to be there, but it is never seriously pursued, and the two characters are gone well before the end credits roll.
So, what does all this mean? That environments where one gender is prevalent cause some members to almost instinctively take on the mannerisms and expected roles of the absent gender? While I do not expect the makers of Conquest to have put that kind of depth of thought into this scenario, I have to wonder if they subconsciously picked it up from what they saw elsewhere, such as during wartime, and transferred it to the already military crew in space? This all needs to be added to the database for discussion on what may happen to crews on long space missions and how things should be handled – or not.
How Not to Run a Railroad
The major themes of Conquest of Space are the depiction of how humans will live and work in space and the reasons for wanting a permanent presence beyond the planet Earth. Both are important ideas we have been addressing in person since before the official dawn of the Space Age in October of 1957, with a great deal of work still to be done. Many aspects of how the film imagined where our technological civilization would be in terms of outer space circa 1980 have either not yet come to pass or did happen but in ways not imagined by the filmmakers in 1955.
Take the space station concept, which Conquest envisioned as a large, spoked wheel-shaped structure serving as a hub for lunar and planetary expeditions, due to the concepts thought up by Wernher von Braun and others. As of the third decade of the Twenty-first Century, we do have a permanent structure circling Earth called the International Space Station, or ISS. However, in addition to being a collection of cylindrical modules and large solar panels rather than a solid wheel, the ISS is primarily a civilian agency operation conducting various microgravity experiments. If missions to other worlds do ever launch from a space station, it will not be the ISS.
As for other envisioned purposes of this space station, including an astronomical observatory, weather outpost, remote military reconnaissance, and even a weapons platform, some of those ambitions were and are conducted on the ISS and some previous stations, but these functions have long been largely taken over by multiple automated satellites – an aspect of the real Space Age that Conquest did not pick up on, whether for dramatic purposes or a lack of foresight.
In our reality, we did put humans around and on the Moon well before 1980 thanks to the Apollo program. We bypassed the need for a space station due to costs and schedules; ironically, America’s first such station, Skylab, was a technological offshoot of Apollo, rather than the other way around. We have yet to send any crews to Mars: The first missions may happen in the 2030s, where it could be a race between the United States, China, and even private industry.
OF NOTE: One aspect of space utilization never addressed in Conquest, which seems ironic considering that the primary purpose of their first deep space mission was to acquire extraterrestrial resources to replenish their society, is the mining of asteroids, more accurately known as planetoids. Millions of these “space rocks” circle Sol containing combined megatons of various valuable raw materials. Unlike Mars or even the Moon, these bodies are relatively small and therefore have a fraction of the gravity well that astrominers would have to deal with on those larger worlds. Although most are much further out in our Sol system, comets would also make ideal places for resources, especially water, which will be vital not only for human survival but as part of our space propulsion infrastructure.
Unfortunately, the only asteroid that made an appearance in Conquest was depicted as a deadly threat to the Mars mission, and a quite bizarre looking and behaving one at that. Overall, the film had the right idea as to what space is for in terms of practical uses, they just needed to expand their cosmic horizons – and so do we.
Moving beyond this comparison of the real and fictional space programs, let us now look at how Conquest considered the best way to prepare humans for the Final Frontier, and whether it was (or will be) the best way to go.
The first terrestrial expeditions, be they across land or oceans, were always either ones of military or economic expansion, or both. If and when science was involved when it became a field in the more modern sense during the Enlightenment, it often rode in the back seat of such expeditions, to use a phrase.
This was even true to a degree for Apollo. Yes, there was certainly a lot of science conducted on those lunar missions, but the program existed primarily as both a geopolitical maneuver of the Cold War and a test of new technologies. There were even some engineers at the National Aeronautics and Space Administration (NASA) who wanted Apollo to be strictly an exercise in mechanical engineering. In truth, if science were the main purpose for sending missions to the Moon, then unmanned explorers would have been able to perform the necessary tasks to learn about our neighbor in space, and at far cheaper costs.
As the ability to reach space via rockets became available to humanity post-World War 2, the Moon was looked upon not as a new Antarctica of scientific research, but a strategic high ground for remote military spying and missile bases. This thinking is reflected in Destination Moon when General Thayer explains to a group of potential investors why the United States must lay claim to the Moon first:
“The reason is quite simple. We are not the only ones who know that the Moon can be reached. We’re not the only ones who are planning to go there. The race is on – and we’d better win it, because there is absolutely no way to stop an attack from outer space. The first country that can use the Moon for the launching of missiles… will control the Earth. That, gentlemen, is the most important military fact of this century.”
Conquest reflects this perspective with a space station manned by military personnel as part of a Space Corps (think Space Force), a branch of the Supreme International Space Authority, which in turn is part of a world which appears to be united as one governing body, possibly similar to the United Nations. Everyone on the first lunar-turned-Mars mission are military personnel, though thankfully Sergeant Imoto is also a trained geologist (in our world, the Apollo program would not have a professional geologist as part of an astronaut crew until the very last landing expedition).
The film reflects the attitude that humanity cannot really move into space until we have set aside our differences and start working together for the benefit of all. While a noble sentiment in theory, in reality the first men to set foot on the Moon did so as part of a political rivalry with a Cold War adversary, which might not have taken place at all otherwise. Even the Space Age itself might have happened much later if not for the machinations of the Cold War.
If the filmmakers were plucking ideas from Wernher von Braun’s 1949 novel The Mars Project as I discussed earlier in this essay, this global unity might have come at a very high price, as the German rocket pioneer envisioned a nuclear war where the Soviet Union was defeated by missiles launched from an orbiting space station. One will note that neither Russians nor Chinese are ever seen or even mentioned in Conquest, although it is not impossible that a few background members of the Wheel came from those very nations.
COMMENT: When Sergeant Mahoney was listing the places he and Sam Merritt had served together during the dinner scene aboard the Wheel, the nation of China was included: “Korea, Africa, China, now space.” Mahoney also mentioned Indochina in another part of the same dinner conversation: Did Conquest incidentally predict the Vietnam War?
To continue: Were the two men in mainland China as part of a military conflict or something else? Does this mean that if the writers assumed the United States conducted a nuclear strike on the Soviet Union that China was also part of this war, being a communist nation and fellow Cold War rival? And what took place with Africa, which is an entire continent and not a singular nation? About the only thing we can assume from the evidence presented to us is that the West is now in charge of this retro-future world.
In the early days of the Cold War, it was often sincerely believed that a nuclear war was “winnable” even though they also knew there would be losses on both sides rivaling any previous conflict. This is why General “Buck” Turgidson infamously said the following in the great Stanley Kubrick film from 1964 titled Dr. Strangelove or: How I Learned to Stop Worrying and Love the Bomb, which reflected real strategic thinking at the time:
“Mr. President, I’m not saying we wouldn’t get our hair mussed. But I do say no more than ten to twenty million killed, tops. Uh, depending on the breaks.”
Would humanity recover from a nuclear war and go on to be a united and peace-loving society reaching for the stars? I must question this thinking, as even the reduced numbers of nuclear weapons in current global arsenals would still be more than enough to wreck our technological civilization and poison much of Earth’s environment for centuries. Certainly far more than just “ten to twenty million” people would be killed in the initial attack as well, with many millions more dying across the planet in the aftermath. Even Star Trek imagined the human race bouncing back from a nuclear war and developing warp drive just a few decades after the event.
However our species became united in Conquest, the next topic to tackle is whether the military discipline approach is the best method to train astronauts for long deep space missions.
There would be a need for a degree of rigid training and discipline to determine who would make the best candidates for such expeditions. However, would this constant control work in the long term? Perhaps for some, but fellows like Roy Cooper expressed a different feeling on the matter after he froze during an emergency situation aboard Spaceship One:
“Every man on the Wheel won his place after six months of the stiffest competition in the world. Each one of us were handpicked from the winners for this special duty. Who else is being conditioned as we are? Special food, special exercise. Tests, lectures! Watched every second! Never any leave!”
For those men who were posted to the Wheel in Earth orbit, a trip home was just a matter of hours away if the need arose. This is not the case on a mission to Mars or some other distant world in our Sol system. Crews would largely be on their own; even radio transmissions from Earth would take many minutes or longer for two-way conversations, depending on where their vessel was headed. While discipline and monitoring would be a necessity for the occupants of such journeys, there would also by necessity be required a level of easing up on certain regulations for the literal sanity of the crew.
Judging by what we were shown in both the interior design of Spaceship One and the treatment and subsequent actions of the crew during their trip to Mars, I am not certain their best interests and well-being were the focus by those who designed the mission – whose members of that team includes Sam Merritt!
COMMENT: I recognize that the issues I will be detailing next come in part from the limited film budget Conquest was given as well as the apparent lack of ergonomic design input when it came to the spacecraft interiors. Although they did have several serious space texts as a foundation for the film, along with other related works and real experts to consult from, the filmmakers cut corners that reduce the levels of how much one should rely on Conquest as a guide to real space utilization. If nothing else, the film can serve as an example of how not to live and work in the Final Frontier, which may be just as useful.
Note the crewmen who were thrilled at the prospect of not going to either the Moon or Mars – not just Siegle but Donkersgoed and Sanella, who literally jumped about and shouted with joy when they were dismissed from the mission by General Merritt. How does this jibe with Cooper’s comment about the stiff competition conducted by those who wanted to be the first to set foot on another world? Did the later “conditioning” turn them off? Is this evidence that military discipline can backfire if pushed too far?
Man Does Not Live on Bread Pills Alone
We have already looked at the pros and cons of a diet made into pill form in the essay section titled “Space Smorgasbord”. The need for providing enough nutrients for a group of people on years-long space missions, which also do not overwhelm their vessel in terms of volume and weight, is certainly a vital matter. What is not so certain is whether such compacted meals would ultimately work, or if the crew would want to subsist on them for several years even if they were viable.
Conquest rather muddied the waters on this issue after making such a big deal on the topic – then they abandoned it altogether! First, we watch the expedition members having to eat their daily pill diet surrounded by the rest of the Wheel crew having full-on meals, including roast turkey! However, once they are on their way to Mars, we do not witness them ever eating anything or even talking about food, although we know they had to. To add further to the contradictions, Mahoney refers multiple times to drinking both tea and coffee in liquid form, even though during the Wheel dinner scene we witness the consumption of coffee pills, complete with cream and sugar pills!
COMMENT: One item that was addressed in Destination Moon but not in Conquest was the issue of swallowing food and drink in microgravity. In Destination, our lunar explorer from Brooklyn had trouble consuming a space sickness pill at first. He is told by a comrade that “it’ll take practice without gravity to help.” When real astronauts made it to the Final Frontier, they found that the swallowing muscles in their throats were able to manage what they were designed to do without difficulty. Space sickness, which would become a real bane for a while in the early years of the Space Age, was another topic that the older film brought to light that Conquest ignored altogether.
As for other needs such as companionship, Conquest treats this largely as a joke, when the subject is broached at all.
Take for example the one “romantic” relationship we see in the film between Jackie Siegle and his girlfriend Rosie McCann. When they are having their personal chat via viewscreen – with the entire Wheel crew watching, no less – we and Jackie simultaneously discover that his planned future wife is cheating on him with a guy named Sidney. Naturally Jackie becomes very upset at this shocking news and tries to attack his rival through the screen!
As Conquest is so good at doing, the subject is never brought up again, although we know Jackie must have been incredibly hurt and embarrassed at such a public loss and blow to his ego. How did this affect his physical and psychological transfer from the Wheel to the expedition ship, where he would be gone from Earth for several years? Siegle seems to have recovered from losing “his” Rosie soon enough, which the filmmakers appear to imply was never a very serious relationship much beyond its physical aspects, at least to them.
However, we know that astronauts are human beings and not robots, warriors, or deities. They have feelings and relationships just like every other member of their species. That they often live and work in a rather different environment than the denizens of Earth make them no less vulnerable to the same emotional issues prevalent in all persons. The infamous case in 2007 of a love triangle gone wrong between active-duty NASA astronauts is a prime real-world example of this very subject. That the space agency has yet to seriously deal with how space travelers will relate and deal with one another on long journeys across the void where the stakes are high and numerous is anything but a humorous matter.
This is one thing both Conquest and NASA have in common: They make it difficult for astronauts and those who want such a career to be anything less than superhuman. They weeded out those considered unfit for such rigorous duties, which is a smart safety action in one sense, but it also keeps those who only might need just a little help to get through certain hurdles from seeking the profession. With the ever improving automated technologies of space travel, in certain respects it should be increasingly easier for humans to function in microgravity environments without having to be any kind of perfect specimen.
Samuel T. Merritt became the epitome of just how badly things could get because he and his crew were expected to be always at the top of their mental and physical game. “It’s against regulations to feel any other way on the Wheel. My own orders,” Sam declares to his friend Dr. George Fenton, even though we had just witnessed him privately losing his composure and requiring some unmentioned medication to get through it.
Suffering from what the Wheel’s primary doctor called “somatic dysphasia,” or, as he adds, a “self-induced inability of the nerves to transmit brain messages…. space fatigue,” Sam is so regulation-oriented that he refuses to seek proper treatment and ultimately has a complete breakdown during the mission to Mars, where he ends up dead at his own hands and comes close to destroying the rest of the ship and crew in the process.
It was also noted by his son early on that Sam has not left the Wheel in over three years. If some of the crew are suffering from space fatigue after only one year, is Sam showing the typical results of a man in space two years longer than them? What does it say for those who will be on multi-year deep space missions with no real breaks? Or are these Sam’s particular issues? Does his age also play a factor here?
That seemingly unrealistic situation where Sam was somehow unaware that the lunar mission was being redirected to a Mars landing (not counting the fact that the change was officially made just a few days earlier from their time period): As George Fenton noted to Sam “a long time ago, the Supreme Council issued an order that the final objective of this project was to be the planet Mars. You were present at all those discussions.”
Could Sam’s changing emotional state and extreme focus on the lunar expedition, plus his unrelenting duties as commander of the space station, have been among the reasons he had forgotten this? Usually, a man of his rank and position would have a dedicated staff of lower-ranking personnel to assist him with both the important and day-to-day details of his various responsibilities, but we see no evidence for such a staff during his assignment on the Wheel.
How about Sam’s multiple times in combat during his three-decades long military career? We know he was injured in battle at least once, according to Mahoney. Did Samuel Merritt ever suffer from PTSD, or post-traumatic stress disorder? This was not a condition that was publicly discussed much or more seriously treated until the Vietnam War, but it was no less real then or now.
Real psychological problems on future deep space missions may not always come from the directions displayed in Conquest via General Merritt, but the potential for them is there. It is one thing to circle Earth for a few months inside a small vessel or space station; it is another matter to be on a deep space expedition lasting for years or more, or settling an alien world permanently. Barriers and even rules will break down; this may be good depending on the needs of the moment, but the possibilities for harm to a crewperson, other members of the team, or the overall mission are there. They will need to be addressed as they occur.
The next question is, who will watch the watchers? Artificial Intelligence (AI) perhaps? If so, will future AI technology eventually make a human crew redundant, even obsolete, unless they are on a mission to settle a new world?
In Conquest, we saw no prepared contingency plans in the event a crewman either died or became a threat to the mission either through mental instability or deliberate sabotage. Perhaps this was considered good fodder for drama, but real life cannot afford to play such games, especially on a space mission far from Earth. As it turned out, both emergency scenarios did happen to Spaceship One and it was almost pure chance in the latter case that the situation did not end in failure and more deaths. The proper checks and balances that might have actually saved Sam in the process were not only absent, but the biases and subjectivity of the crew, especially by Mahoney and Barney, only serve to exacerbate the events.
As to the interior design of Spaceship One, human aesthetics did not seem to be part of the planning. We never saw where the men slept or ate: Where was this “parlor” Mahoney referred to when he asked Sam to join him for a cup of tea? The ship doesn’t seem big enough to have any kind of actual cafeteria or kitchen. And did those safety couches double as beds? If so, then why did Sam tell the crew they would not need them during the interplanetary cruise until they reached Mars? Was he just talking in terms of flight safety?
Did these men have any place to go on the ship just to have a few moments of privacy from the rest of the crew? Judging by Siegle’s sarcastic comment during his interplanetary EVA, my guess is there are no dedicated locations for this need in Spaceship One.
We also never learned where the men disposed of their bodily waste while living on the vessel. I know showing a toilet or any such facilities were verboten in the Hays Code era of cinema, but since it is an absolute necessity for any human crew in space, waste management systems are a must. Even Star Trek one decade later became infamous for never showing a bathroom or having a crewmember even mention the need to use one.
Ironically, in our real space program, it is one of most asked questions about the astronauts by the general public. There was even a popular book written by a real astronaut with the title: How Do You Go to the Bathroom in Space? By Colonel William R. Pogue (1930-2014) who flew as part of the Skylab 4 mission between 1973 and 1974: That crew’s human endurance record of 84 days in space would not be broken until four years later, and then not by a NASA crew for another twenty years.
As I mentioned earlier in this essay, where were the Spaceship One crew’s personal items for this long voyage to Mars? We saw that Siegle had brought a harmonica, but where were the books or board games, even a deck of cards? The film gave the appearance that the men would be working most of the time when not eating or sleeping (which we were also never shown outside of them drinking their water ration on Christmas Day). Considering the amount of undue stress the men were under, as well as the undoubtedly long stretches of boredom both in space and on Mars, some means of distraction and emotional relief were in order.
The interior design and color layout of their first manned vessel to the Red Planet also left much to be desired. As you can see from this image of Siegle sitting at his radio control station, the color scheme is invariably white walls, ladders, and support struts, broken up only by solid black panels.
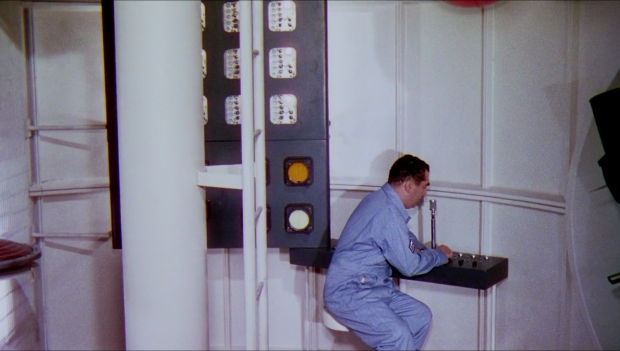
FIGURE 14. Sergeant Jackie Siegle sits at his post, the radio station console aboard Spaceship One. Like most of the vessel’s interior, it is not exactly designed for human comfort, either physically or emotionally.
Besides a lack of color, there were no photographs or paintings on the ship walls, or charts or anything else that we saw. The crew did some minor and temporary holiday decorating on Christmas; however, had that seemingly miraculous snowfall not arrived when it did to bring them water, one gets the feeling those ornaments would not have been enough to stop a complete breakdown of morale.
The USS Discovery spacecraft in 2001: A Space Odyssey was also fairly void of decorations and vibrant colors in its interior sections, often looking about as sterile as a hospital corridor. However, this was a deliberate artistic choice to emphasize how dehumanized people had become by their technology. I do not think the same conscious aesthetic level of thought went into the interior layout of Spaceship One.
It is films like Conquest and the overall representation of humans in space through most science fiction that led me rethink how our expansion into space should be done. My ideas began to solidify in 2017 with my Centauri Dreams two-part essay on Forbidden Planet in and more recently my piece titled “In Person or Proxy to Mars and Beyond?” which you may read here:
In Person or Proxy to Mars and Beyond? | Centauri Dreams (centauri-dreams.org)
The genesis for this essay came from The Mars Society to write an article on the future of human exploration and settlement of the Red Planet. I saw this as an opportunity to bring up my issues with unmodified terrestrially raised people trying to settle environments they were not truly conditioned for, as well as the matters I have already raised about our space program’s lack of experience and preparedness for long-term space dwelling.
Watching Conquest, I realized that, despite having been made before the official dawn of the Space Age and with all its Hollywood-induced constraints, the film actually further bolsters my stand on the need for modified humans to be successful in the Final Frontier. This assumes we do not end up going strictly with AI and robots altogether venturing into space instead of our species and humanity lives and experiences their adventures vicariously through teleoperations and virtual reality (VR).
Explorers… or Invaders?
Apparently when Conquest of Space premiered there was still something of a debate whether humanity should venture into the wider Cosmos, not over the technical ability to accomplish such a goal, but if we were venturing into a realm not meant for mere mortals.
This was hardly a new concept, as throughout our history, self-appointed authority figures declared via the deities of the day what people could and could not do or dare to go.
The heavens, which were first assumed to be the literal place where gods and their minions lived, only to be later moved to a realm beyond our concept of the Universe, were deemed especially off limits. Of course, for the longest time few were concerned about anyone actually reaching space/heaven, for even flying in the air wasn’t a possibility for humans, let alone any higher up.
Whereas the long-term trope has been that our species thought we were the literal Center of the Universe and everything else circled around us, the truth is something rather different. Referring to Western culture, humanity was seen as occupying one of the lowest layers of existence residing on Earth, as if we were at the bottom of some cosmic pit where all the refuse and filth, both physical and moral, sunk to. The only thing lower than us was the underworld and its denizens. Above us reality was seen as pristine and pure, especially as one got closer to Heaven.
COMMENT: This is why Galileo and his contemporaries were excited about the Copernican view of the Universe. Instead of being stuck at the lowly bottom of reality, Earth was instead another planet circling Sol – meaning we were up in the heavens with the rest of the stars, which may also have planets and life of their own! I am rather simplifying the situation here, but I want you to see the main point. For much more information on this subject, please read The Book Of The Cosmos: Imagining The Universe From Heraclitus To Hawking, edited by Dennis Richard Danielson. January 15, 2002, Perseus Books Group edition.
The scene where Sam and Barney have an informal debate about whether God wanted humans in space I have transcribed in the essay section “Too Perfect to the Accidental”. I felt that Barney made some very good counter points with his father regarding how the idea of traveling to other worlds, or even the general modern structural layout of the Universe, would have been concepts well beyond the authors of the Bible, who were certainly neither scientists nor astronomers even in the ancient sense.
One issue that has changed its locations but not its content and urgency over time is the concern that Sam Merritt also thinks that some of the other planets in the Sol system “might already be tenanted.” His son disagrees with him, adding that “the Universe was put here for Man to conquer.”
Although by the 1950s most scientists would have expressed strong doubts that intelligent beings could exist even on Mars, there were some who held out otherwise. On the other hand, many of the same professionals felt reasonably certain that the Red Planet did have some forms of plant life and perhaps even lower animals. Even von Braun’s framing story The Mars Project involved the discovery of living, civilized intelligent natives on the fourth world.
While today virtually no serious scientist considers any other world in the Sol system besides Earth to be the residence of highly intelligent organisms, the prospects for extraterrestrial intelligences (ETI) elsewhere in the wider Milky Way have only become stronger with every new “smoking gun” discovery. This has added concerns by some how we might one day treat such beings as we expand into the galaxy, based on past human history between members of our own species. There have also been many concerns how some advanced ETI might treat humanity should they learn of our existence, also based on our own history of first contact.
In terms of whether God wanted us or not in His realm, things changed not long after the release of Conquest of Space, when the Soviet Union placed the first satellite into Earth orbit. Sputnik 1 shocked the West because most experts thought the nation wasn’t quite advanced enough to perform such a technological feat – even though Soviet officials publicly announced they were working on just such a project several years earlier.
The idea of an atheistic communist state building and launching satellites to circle Earth and beyond was too much of a potential threat for the West not to respond to. The narrative soon changed from whether God wanted us up there with Him at all to God demanded that we respond in kind with our own spacecraft to keep the heavens open and safe for God-fearing and freedom loving people everywhere!
As for Conquest’s take on the subject, even though Sam and Barney were fairly reasonable and polite about the subject matter with each other during their discussion, Sam’s later slide into outright madness and his violent actions fairly ruined any chances of the audience agreeing with the General’s side of things, except for those who also felt as strongly as he did and ignored any counter evidence.
As we do expand into space to one day include the worlds of other solar systems, will this debate rise again? We are already seeing signs of it even though the first true interstellar probes are decades away from being sent to the nearest stars. How things may turn out will depend on what methods we use to explore the Milky Way and who encounters whom first.
A More Modern Mars than Most
One of the pleasant surprises about Conquest is its depiction of the planet Mars. In the mid-Twentieth Century, science fiction often held the Red Planet to be an abode for life both intelligent and otherwise. This remained the case even after the first interplanetary probes examined the world up close in the 1960s and found it to be seemingly more like Earth’s Moon (also see George Pal’s 1953 film The War of the Worlds as just one example).
Real scientists were inclined to assume that at least some forms of lower life were present on Mars. The September 1955 issue of National Geographic Magazine contained an article by astronomer E. C. Slipher (1883-1964) blaring among other things that a large patch of green appearing on Mars roughly the size of Texas could only be growing plant life. The issue came complete with a beautiful color photograph of the planet showing this very phenomenon via a large Earth-bound telescope.
That is why the film’s portrayal of Mars is rather surprising, considering how Conquest often skipped over other scientific and technical points. Granted, they did have renowned space artist Chesley Bonestell do the research and work for depicting Mars, but apparently he was dissatisfied with the final results in the film. This is both ironic and unfortunate, as the planet shown in Conquest was much closer to the reality than would be realized until humanity began exploring Mars in-depth.
When we first see the Red Planet as Spaceship One approaches it in the film, it is remarkable how much Mars would resemble what later real orbiting probes would send back to Earth. There are large craters, both of the impact and volcanic variety: Scientists were genuinely surprised to see craters on Mars when Mariner 4 returned many images of them from its historic planetary flyby in 1965. Volcanoes would not be discovered until Mariner 9 began orbiting the planet in late 1971, although active versions were suspected to be causing the changing darkened regions on Mars even in the 1950s.
The Mars of Conquest also has what appears to be dry river channels and even a few regions suspiciously resembling small lakes. Mariner 9 would also first reveal these channels, which scientists suspected were cut by flowing water eons ago. In addition, the American probe would also discover that Mars contains several massive canyon systems, one of them which would stretch across the continental United States.
The “canals” of Mars are seen across the face of this Red Planet. However, they are not the artificial construct of a native civilization that used them to transport water from the Martian poles to their cities along the equator as Percival Lowell (1855-1916) believed what he saw through his telescope to be. Rather they are more natural features which astronomers like E. M. Antoniadi (1870-1944) concluded with their careful observations during brief times of clear seeing.
The surface of Mars as designed also needs to be applauded in the scientific sense. As seen as early as the Viking landers, boulders do indeed cover much of the planet. Most pre-Space Age (and even some post) versions of the Martian landscape, when not covered with vegetation or canals, were more like a flat sandy desert devoid of rocks and boulders. There are of course such places on Mars, but a fair deal of the planet’s face has rocks of multiple sizes deposited from impacts, shifting ancient water activity, and volcanic actions.
Conquest gave Mars some seismic activity and caverns to spice up certain scenes near the end of the film. It has been determined that the Red Planet does have both: In regard to quakes, the ones that were recorded by the Interior Exploration using Seismic Investigations, Geodesy and Heat Transport, or InSight, mission between 2018 and 2022 were not as powerful as the one in the film (the largest one measured a five on the Richter seismic scale). As for caverns, Mars orbiters have detected their openings on the surface, but exactly how many exist is yet to be determined. If the planet is riddled with them as Conquest seems to imply but are more structurally stable, they may be utilized as naturally open places to set up settlements, using the Martian regolith layers to protect the human inhabitants from ultraviolet rays, cosmic rays, and meteoroids.
As the explorers of Spaceship One learned the hard way, the surface of Mars in their universe – certainly at least in their landing region – was quite devoid of water in any form, even well below the surface. This feature of the cinematic Mars is also closer to the reality of that world, although water ice in vast amounts may exist deep within the Martian crust.
Nevertheless, this Mars can support at least one form of terrestrial life as Sergeant Imoto demonstrated with his sprouting flower, with a little help from some concentrations of water and perhaps the remains of the person he planted the seeds upon. As for native organisms, there seem to be none at all on Conquest’s version of Mars, another minority take on the planet by the film that would be questioned well into the era of robotic exploration of the Red Planet.
The only aspect which Conquest really missed on was the color of the sky, but until the first color surface images were sent back to Earth in 1976, it was assumed that the planet’s thin atmosphere meant a dark blue daytime sky. Scientists did not anticipate a salmon pink sky due to levitating dust particles, even though they were long aware of the planet’s many global dust storms.
I have to speculate that if Conquest of Space had been a better and more successful film, its version of Mars might have caught on with both scientists and Hollywood, tempering our views of our neighboring world perhaps far enough that contemporary scientists would have been more accepting of some of the less sanguine data about Mars and become less disappointed with what our robotic space explorers did discover there. This in turn might have kept at least the American space program from turning away from sending manned missions there, which were considered as early as the 1980s.
Turning Mars into Earth 2.0?
“Stupid or callous it may seem to be at this time. It is not senseless! Man’s very survival on Earth depends upon the success of this or some future search for a new source of raw materials.” – Dr. George Fenton
“Japan’s yesterday will be the world’s tomorrow. Too many people and too little land. That is why I say, sir, there is urgent need for us to reach Mars: To provide the resources the human race will need if they are to survive. That is also why I am most grateful to be found acceptable, sir. I volunteer.” – Sergeant Imoto
“I think these soil and mineral samples will prove that life is possible on Mars. It can be done, sir. AII the elements are in those sacks. Even air and water, in other forms.” – Sergeant Imoto, again.
“Until now, this little planet has been alone… friendless, all drawn up into itself. So, it’s crusty, dried-up, and unyielding. But with patience and understanding and hard work, it could be made to blossom.” – Sergeant Imoto, one more time.
Coming of age, or at least better awareness, in the 1970s, it was being made culturally clear that Earth was a finite globe floating in space. The resources being used by the growing billions of human beings living on our planet’s surface could not last forever. Long before they would run out, our civilization would descend into want and chaos as fossil fuels were depleted along with the dwindling number of places just to be able to live.
Space was seen as one of the best answers to this potential crisis. Folks such as Gerard K. O’Neill (1927-1992) envisioned large space cities circling Earth and elsewhere independent of residing on any world. The resources to build them would come from the Moon and the planetoids, where it would be easier and cheaper to mine and deliver them and thus avoid Earth altogether.
Conquest was a bit ahead of its time when it came to the reasons for utilizing the Final Frontier. Not only did it already have a floating space city of sorts in the form of the Wheel, but it foresaw the need for natural resources on other worlds to replenish our home planet long before the concept became “trendy” – or at least as an alternate source. This was somewhat of a contrast to Destination Moon, which saw Earth’s natural satellite more in terms of geopolitical and military strategies.
Of course, as with many other topics on its plate, Conquest was a bit muddled in certain respects when it came to this goal. For one, it is not entirely clear whether their space authorities want to explore Mars to bring back its resources to restock Earth, or to settle Mars to transform it into a place where humans along with terrestrial fauna and flora could expand to and thrive. Perhaps they wanted to achieve both.
This may be why the Supreme International Space Authority decided to bypass the Moon and send Spaceship One straight on to Mars, logic and practicality aside. For turning our natural satellite into another Earth probably seemed too much to accomplish in short order and they were desperate to replenish our resources much sooner than later.
Otherwise, it did not make a lot of sense to overlook the Moon in terms of settling space and mining there (the same goes for the asteroids, which I mentioned earlier). The Moon is much easier to reach than Mars via spaceship and it certainly contains many resources of its own, whether you could plant an unprotected terrestrial flower in its regolith or not.
This makes me wonder if this is the other reasons Mars had no signs of native life forms in the film, so humanity could claim the Red Planet for itself without having to feel guilty about taking it away from anyone, not even a Martian microbe.
To requote Sergeant Siegle as he was desperately searching for any water while stuck on Mars:
“No signs of water. Not even bugs or worms. They ought to give this planet back to the…. Who do you give it back to? No people.”
This topic also made me wonder about two events that took place on Mars due to its first visitors from Earth:
Sam Merritt died and was buried on the Red Planet. The crew of Spaceship One knew what really happened to the General and why, but they decided to make Sam into a hero upon their return to Earth.
I have to wonder what will happen one day if a future space archaeologist decides to examine the site of the first human burial on Mars? Will they note that Sam’s remains have a bullet wound in them, perhaps even the bullet itself? If none of the crew ever came clean as to the real story, just imagine the speculation from such a find – although Sam did leave some clues in his words and behavior during several radio conversations with the Wheel, which I would imagine were recorded somehow.
Perhaps this is all irrelevant if Sam’s grave fell into one of those caverns the spaceship was opening up as it tried to escape Mars. The General’s resting place was quite close to the rocket, so even its propulsion exhaust alone might have been enough to damage or even destroy the site. The only protection between the spaceship and Sam’s remains were a couple of feet of Martian regolith.
Now about the flower that Sergeant Imoto placed on Sam’s resting place: Was it also destroyed by the rocket launch or the collapsing ground? Or did the little plant manage to survive long enough to germinate and spread itself? The flower was hardy enough to start growing in this otherwise alien environment and we have seen on Earth how tenacious certain species can be once they get a foothold in a place even with just the bare minimum requirements for survival. Did Sam’s remains provide the extra nutrients the plant needed to grow?
Another factor in the flower’s favor is that it would have no competition for Martian resources for quite a while, either native or alien, so the plant could spread quite far, adding a fair deal of oxygen to the planet’s atmosphere in the process.
Imoto himself said that this Mars had all the elements necessary for sustaining life, including the occasional replenishment of water via snow. Perhaps he had inadvertently begun the terraforming process for Mars; this would certainly make things a bit easier for those settlers who will undoubtedly follow.
Conquest of Space… the Television Series
Was Conquest of Space the inspiration for a potential television series in the late 1950s? After all you have read and learned here about the film by now, one might be rather inclined to think otherwise, considering how profit-oriented the entertainment industry is: Conquest did not even recoup its production budget upon its release in 1955. In addition, the film was soon followed by far more popular, better produced, and – dare I say – more entertaining fictional exploits of brave men and some women boldly going into the Final Frontier.
Nevertheless, I discovered during my research on Conquest of Space that in 1959, Paramount Pictures Corporation had produced a film titled Destination Space that was in fact a pilot for a potential television series on the Columbia Broadcasting System (CBS) network. It would ultimately lose out to another series about men exploring space and subsequently fade away into obscurity.
Until now.
What was Destination Space about? In short, the United States space program, under the leadership of one James Benedict, is attempting to send the first manned mission to the Moon guided from the first manned space station in Earth orbit, a giant and very familiar-looking wheel-shaped structure.
The lunar expedition is aborted when a cluster of meteors smash into the space station, endangering all aboard. Back on Earth, Benedict has to defend the existence of his station from a senator who thinks it is a waste of money: They derisively nickname it “Benedict’s Billions.”
A second attempt is soon made to reach the Moon, but a new mishap befalls the mission: This time the lunar spacecraft’s nuclear engine malfunctions due to a buildup of ice. Only the quick thinking and response of the ship’s commander, Dave Reynolds, prevent a deadly explosion. An impartial observer sent up to the space station by the U.S. Senate to monitor the mission, Dr. Kurt Easton, decides that humanity must continue to strive for the Final Frontier, despite the dangers and setbacks.
Thanks to the sometimes miracle that is the Internet, you may watch the entire pilot episode here:
https://www.youtube.com/watch?v=uVQaOmcgm-E
One thing you will discover early on from Destination Space is that all the exterior scenes in Earth orbit are taken directly from Conquest of Space. That both products were owned by Paramount no doubt saved a lot of money on copyright fees and the budget in general. Perhaps this was also the studio’s attempt to get back some of its box office losses from the 1955 film.
The style and feel of the original film were easily adapted to the pilot in other ways. The opening of Destination Space utilized the introduction of Conquest, only modifying the title card and the narration to fit the pilot plot:
There are no limits to what man may achieve, for there are no boundaries beyond his dreams and no dreams he may not ultimately make come true: Destination Space!
The rocketship rode in orbit close to an incredible wheel-like structure that spun gently in an ocean of seeming nothingness 500 miles out from Earth.
This multi-man space station, dubbed BB, Benedict’s Billions, had been made a reality by a tremendous number of scientists and technicians working against fantastic odds and all of this stupendous effort had been spent in preparation for the next few moments to come.
It was nearing the instant of launching of the rocket ship that was intended to carry the first men into orbit around the Moon. The principal responsibility now was upon one man… that man was Jim Benedict, director of the U.S. space program.
COMMENT 1: Nice to see they fixed the Conquest dialogue about the space station being in a one-thousand-mile-high orbit, as the potentially lethal Van Allen radiation belts circling Earth had become known in the four years between the two productions.
COMMENT 2: Alford “Rip” Van Ronkel (1908-1965) was not only the producer and writer of Destination Space, he was also the co-screenwriter of Destination Moon. This helps to explain the efforts made for realism we see in this television pilot/film.
There are other similarities between the two productions: The story revolves around the first manned expedition to the Moon – the original mission destination in Conquest. The spaceship has been put together by crews living and working on the nearby space station. The crew work and social structure is modeled on the military format. Even a meteoroid storm hits the space station early on in Destination, using the exact same footage from Conquest. This cosmic disruption delays the lunar mission and is the catalyst for the rest of the plot.
What sets Destination Space apart from its cinematic inspiration are the places its story goes that Conquest never really touched upon. These places include a serious effort for realism and a maturity in its main characters, who also contain a profound lack of stereotypical ethnic behavior.
For example, the space station and lunar mission are not just threatened by cosmic and technological forces but also terrestrial ones: Certain politicians in Washington, D.C., think “Benedict’s Billions” is a huge waste of taxpayer dollars. Cutting funding for this space program will also make these particular politicians look fiscally responsible in the eyes of some of their constituents. A good portion of Destination involves the U.S. space program Director Jim Benedict confronting Congress to defend his work and give the reasons for humanity’s needs to expand into outer space.
Here is the transcript of the key part Benedict’s speech before these politicians. It is quite relevant 65 years later. You may decide whether that is a good or bad thing in terms of our progress as a civilized species:
Sir, there are any number of hazards and dangers, ranging all the way from radiation to innumerable possibilities of mechanical failure. But if you demand my frank appraisal of what I consider to be the greatest danger, it’s the vacillation. The continuing swings of certain sections of public opinion.
From the earliest days of Cape Canaveral, when we were first trying to outdo the first Sputniks, many people have teeter-tottered between anguish and triumph. Whenever the enemy made the slightest advance, loud voices proclaimed that we should beat them at all costs. And whenever the slightest gain was made, this unfailingly was followed by periods of apathy or complacency.
And through it all, a few kept up the weeping and the wailing over the cost of survival. And there have always been political opportunists ready and waiting to grab a headline by jumping on the crest of each wave, whether it be one of enthusiasm or one of despair.
Those of us on these projects are well aware of our responsibilities. And we recognize the value of committees such as this one, calling us in from time to time for a reckoning. And it’s good that they take us to task and keep us on our toes. It’s of little importance whether one by the name of Benedict bears the principal responsibility, or someone else.
I do not speak for myself except to say that if I seem to be failing, I should be replaced immediately. But let us make up our minds once and for all whether we want an all-out space program or not. And if we decide that we do, as I pray we will, then let us remain constant to that purpose.
And in the name of the future of our country, let us stop being like a changeable wind, blowing hot one day and cold the next. Instead let us go forward in the American way. Pioneering new frontiers without fear. Taking pride in accomplishment, yet facing dangers and disappointments with resoluteness. And without qualms and complaint.
Budgets for the space program were not a focus in Conquest, as the space effort in that world was handled by an international community and operated by combined military services, all with seemingly unlimited supplies of money and resources for exploration beyond Earth.
Although set in its own version of a retro-future, Destination Space takes a more relatable approach to our world. Most of the real history of the government-sponsored U.S. space program has been one of wrangling with politicians over funding and an often apathetic public often neither able nor willing to see the larger picture.
I would love to tell you that, this time around, women play actual roles in the exploration of space in Destination. Unfortunately, they exist here to create a melodramatic side story involving a love triangle. On the plus side, the women in Destination are mature and intelligent partners to the main characters, not the two-dimensional showgirls we mainly saw in Conquest.
At least when it came to the space-venturing people shown in this pilot, what they lacked in diversity they made up for in being competent professionals who deeply desired to be in outer space and truly appreciated why they were “pioneering new frontiers without fear.” It was also apparent, without even the need for a mention, that any religious qualms about humanity entering the heavens were long decided upon in favor of America and its values leading the way into the Final Frontier.
Destination Space might have worked as a television series, except that, in essence, the pilot was too grown up for its own good. For one, Destination was probably seen as too “talky” in a time and place where most people associated space with zooming rocket ships, laser guns, exotic worlds, and alien monsters. The audience did not even get to see the Moon mission finally happen as a serious technical issue brought things to a halt the second time they prepared to launch the ship.
That the makers of this flick repeatedly reused spaceship scenes from Conquest tells me they did not have the budget for anything too flashy and dramatic in terms of exploration. They couldn’t even borrow from their cinematic source to create any lunar visit scenes as Spaceship One had been diverted from the Moon to Mars.
Another reason Destination Space never left the proverbial launch pad was the competition it had with another pilot being offered to CBS television at the same time, a program called Men into Space.
This program also portrayed realistic space missions as imagined for the then-near future, but Men into Space had a more expected spirit of adventure where its varied extraterrestrial missions usually succeeded despite the dramatic dangers they encountered. CBS went on to buy its pilot, but despite all the positive aspects on its side, Men into Space only lasted one season. One ironic reason for the series failure to continue was the need for a big enough budget to properly display all its special effects.
If Destination Space had been greenlighted as a series, I wonder how well it might have done with American audiences? On the one hand, it was quite mature and realistic, uncommon traits for a member of its genre in that era. However, then as now, most people just want to be entertained rather than lectured or be overly reminded of everyday life when they plant themselves in front of a television.
Nevertheless, if Destination Space had become a series, its makers would have had to create episodes with exciting space missions and drama outside the halls of the federal government. Even in the pilot episode, the lunar mission was beset first by a swarm of meteoroids crashing into the space station, then the threat of a nuclear meltdown and explosion.
Even if Destination decided to focus on politicians and bureaucrats as the main villains (I also have to wonder if some Cold War enemy operatives would have joined in the fray), it might have worked in the same way that legal dramas set largely in courtrooms often succeed to keep the viewer’s attention with compelling human interest stories, while framed with events in the lives of the main characters outside the halls of justice.
It was exciting enough to see that Conquest might have had a chance at some redemption through a potential television series focused on space exploration and utilization that used its special effects. Although it ultimately did not happen, we do have an interesting piece of television history inspired by its existence.
Back Draft
When I am researching my essays on classic science fiction films, one thing I find especially useful to my deeper understanding of the cinematic effort I am writing about are the drafts of the filming scripts. Here you not only get the details from stage directions to character backgrounds and motivations, but also insights that might have otherwise been elusive in the final work.
It is especially intriguing to come across scenes and dialogue that did not make it to the final cut. In the case of Forbidden Planet, a draft script from 1954 available online gave me many wonderful bits of knowledge about the film that were not in the version released to theaters just two years later. In almost every case of the science fiction films I have written about so far, there was a script on the internet for free, just waiting to be explored and appreciated.
Unfortunately, Conquest of Space is not among those films where a free draft script may be found in cyberspace, at least so far as my various searches could determine.
Oh, there are transcripts of the film’s dialogue online in a state of freeness, but they contain only what the characters said in the final released edit and nothing else, not even labels for who said what. Now these transcripts do serve a useful purpose and help a fair deal when it comes to finding exact quotes. However, their lack of any adornments in terms of what I listed above makes them only so useful compared to a script draft that the very studios making a film had created for their team.
You may find links to the transcript for Conquest of Space in the “References” section at the end of this essay.
Normally, the links to an online draft script of the film I am essaying about also end up in the “References” section for easy finding and enjoyment by my readers. However, the circumstances by which I did find an actual film script for Conquest lead me, for the first time, to give the subject its own section in one of my essays. I admit up front that part of my motivation for this was frustration, as you will see.
When I initially went searching for the script draft of Conquest, I thought it might be a fairly easy find, as I came upon similar writings for science fiction films that were much bigger and more popular than this example of George Pal’s creation.
Soon, however, I learned that this was not the case. A number of sites devoted to archiving films scripts, both free and for sale, did not even have Conquest among their inventories. It dawned on me that the lack of popularity for this film might make finding a real draft more difficult than many other films, just as I had trouble finding any blueprints or other diagrams of the Wheel and especially Spaceship One online, compared to many other fictional spacecraft and vehicles.
COMMENT: Such vessel layouts would certainly have helped me with the questions I had as to where various sections of Spaceship One needed to exist for its human crew, which included where the men ate, slept, and removed bodily waste. Yes, there are those earlier publications by Willy Ley, von Braun et al with their beautiful diagrams, but they envisioned much larger and more complex expeditions in terms of crewmen, ships, and materials. Even their later revised mission plans with fewer vessels were still more than the film budget of Conquest could handle.
When I finally found a real printed draft script for Conquest online, I was shocked: It was for sale, and not cheaply. The document eventually sold for $1,800 dollars! Perhaps needless to say, I was not the one who purchased it.
The site which offered the script, whose business includes selling physical draft scripts of classic films to collectors, may be found below, with the link going directly to the Conquest page:
https://www.walterfilm.com/shop/movie-scripts/conquest-of-space-nov-9-1958-film-script-by-james-ohanlon/
Only three pages of the Conquest script were posted on the site and only one of them showed any story details. That page contains the scene where Dr. Fenton first arrives on the Wheel. The script matches what was shown in the film, except for some very brief dialogue and actions between Siegle and Imoto framing the moment that did not make the final print. Between the outrageous sale price and this minimal example, you can imagine how disappointed I was.
There was one ray of light in what I had found, however: The person who sold the Conquest draft did go into some useful background on what was in the work, which was authored by James O’Hanlon (1910-1969) and dated November 9, 1953 (FYI, O’Hanlon co-wrote the screenplay for Destination Moon).
Allow me to quote the following:
The O’Hanlon screenplay dated November 9, 1953 is fairly close to what Pal and his director, Byron Haskin, actually filmed — with one major twist. When the ship’s captain (named Lewis in this draft) goes mad, turning into a religious maniac, and attempts to destroy the vehicle before it can reach its destination, he is stopped by the first mate Merritt who kills him in defense. The surviving crew members, honoring the good the captain had previously done, decide to tell the world that he died heroically (as in the John Ford films where someone decides to “print the legend”). The major change between this draft and the completed film is that in the movie the captain has been renamed Merritt and is supposed to be the first mate’s father! This particular aspect of the movie — the father turning into a religious fanatic, and his son having to kill him — was enough to secure the film a “Condemned” rating from the Catholic Legion of Decency.
Speaking of the director of Conquest of Space, Byron Haskin (1899-1984), I learned some very interesting things regarding this man’s long and fruitful cinematic career: Conquest was neither Haskin’s first nor his last directing of a science fiction film. His resume, which goes back to 1927 in this duty, includes The War of the Worlds (1953), From the Earth to the Moon (1958), and Robinson Cruise on Mars (1964). Haskin also directed six episodes of The Outer Limits television series (1963-1965), including some of its best examples, and co-produced the original Star Trek pilot “The Cage” in 1964!
Among this sampling of Haskin’s career listed above, I want to highlight the film From the Earth to the Moon, which was based (to a degree) on the landmark 1865 novel by author Jules Verne (1828-1905).
The original novel, which described a manned trip to the Moon, was to the Nineteenth Century what Destination Moon was to the mid-Twentieth Century: An accurate as possible rendition of a mission to Earth’s natural satellite based on the scientific knowledge and technologies of the day.
Whereas Verne’s novel was a straightforward story of three men journeying to the Moon in a space vessel (shot out of a 900-foot-deep cannon from central Florida), the makers of the 1958 cinematic adaptation took a number of liberties with the 1865 story, which suspiciously mirror a certain science fiction film that Haskin also directed just a few years earlier…
When the spacecraft is on its way to the Moon, one of its crewmen, Stuyvesant Nicholl, informs his fellow astronauts that he has sabotaged the vessel, for he sees what they are doing as an unauthorized violation of God’s realm. His attitude changes when he discovers that his daughter Virginia has stowed away onboard the ship to be with crewman Ben Sharpe, the assistant of mission leader Victor Barbicane. The two men decide to separate the vessel into two parts, sending the lovers back to Earth while they head on to the Moon, never to return (but they are able to let the couple know that they made it to the lunar surface safely).
To be blunt, From the Earth to the Moon makes the flaws in Conquest quite bearable in comparison. As you can see from the brief description above, somebody on the production team was definitely influenced by the former film, for those plot elements of religious fanaticism and a stowaway do not exist in the Verne novel.
For good measure, the three brave astronauts make it back to Earth alive and well in Verne’s sequel novel, Around the Moon (1870), splashing down in the ocean and being recovered by a United States Navy ship just like the Apollo astronauts would do for real over one century later.
As for Byron Haskin’s other directing job, Robinson Crusoe on Mars, I noted the following: The “massive” meteor that forced the crew of Mars Gravity Probe 1 (MGP-1) to abandon their ship and crash-land separately on the Red Planet bore more than a little resemblance to the glowing orange asteroid that imperiled Spaceship One in Conquest, complete with trailing debris. In addition, the design of the alien vessels that were later seen hunting our two main characters are basically taken from the Martian war machines in The War of the Worlds, minus the copper cobra-head death rays.
My Overview and Final Thoughts
Conquest of Space was George Pal’s attempt to follow up on his hit film Destination Moon, about the first manned expedition to Earth’s celestial neighbor. This time he was sending astronauts to the planet Mars with the goal of nothing less than saving all humanity from resource depletion. In addition to a fabulous, winged spaceship to bring the crew to their distant destination, the plan included a huge white wheel-shaped space station in Earth orbit meant to help construct and supply the vessel and then monitor its cosmic voyage.
Pal and his film production team also gave the characters of Conquest more personality than we saw in the cast of Destination Moon. As if this were not enough, Conquest would be based on the then-recent publicized concepts and designs for future space exploration by famous real experts in the relevant fields. If nothing else, it was an ambitious endeavor seldom considered for a Hollywood science fiction film of the day.
Unfortunately, Conquest of Space as envisioned was a bit too much to put as hoped on the silver screen. The ambitious plans were there, but the whole didn’t quite make it. Yes, we had a plot and the characters did achieve their goals in the end, but there were lots of missteps along the way. Conquest was considered a failure both at the box office and with critics and the public alike. The film was often left out of lists of the best science fiction works of its era and began its decline into obscurity.
Despite all his previous successes with the genre, Pal turned away from both science fiction cinema and Paramount Pictures and would not make another such film until five years later with The Time Machine, based on the 1895 novella by H. G. Wells and released by Metro-Goldwyn-Mayer (MGM) Studios.
I was among those who initially did not care for Conquest. There were ridiculous technical elements such as switching the target of a deep space mission from the Moon to Mars as if one were simply changing a commercial plane flight. Even more grating, we had characters who, if some weren’t being bland and sometimes hard to tell apart, the others were often portraying ethnic stereotypes mainly for laughs, which also didn’t quite work.
To add fuel to the fire, most of the men going to Mars paradoxically didn’t want to be living and working in space in the first place. Then the mission commander has a breakdown and tries to destroy the ship and crew because he thinks God doesn’t want people to be in space. On Mars we watch the survivors struggle with each other and what appears to be a very dry and dead planet, until a series of lucky events allows them to finally blast off for home with the physical evidence that, yes, space can be conquered for all mankind.
I wanted to give Conquest another chance with the hope that this time I might find some gems in among the cinematic dirt – and I did. Oh, the film did not suddenly get better as either as a work of celluloid art or a realistic look at deep space exploration with repeated viewings as I conducted my research for this essay. The missed opportunities and inconsistencies were even more frustrating as I gave them a deeper examination. I did find some redemption in most of the main characters regarding their flaws both as people and as written, with one glaring exception who came out even worse than before.
I did admire how Pal at least tried to make something that was not typical for contemporary Hollywood with a real message for the world that space utilization is our future and will save our technological civilization. After all, as astronomer and science popularizer Carl Sagan (1934-1996) would later state in his 1994 book Pale Blue Dot: “All civilizations become either spacefaring or extinct.”
As for how we will go about expanding into the Sol system, Conquest can at least serve with important examples of how likely not to conduct this business. Are concentrated food pills the way to go for keeping astronauts fed on years-long deep space adventures? Perhaps not, but something needs to be done to resolve this vital issue.
What are the best ways to determine who can go on these missions and how will we keep everyone alive, content, sane, and functioning? Conquest took the military culture approach: This may work for short-term durations, but much longer expeditions will need to modify these restrictions and psychological invasions. Eventually, it will have to be a whole new ballgame when people begin settling space permanently.
I was truly impressed at how the planet Mars of Conquest seemed so close to the reality we now know of that distant place almost seven decades later, far better than many renditions even years after the Space Age began.
For example, returning to Robinson Crusoe on Mars: Although the film premiered just one year before Mariner 4’s historic flyby and the depiction of Sol 4 was considered to be at least scientifically plausible, this version of the Red Planet has numerous unexplained fires on its surface, an atmosphere just dense enough to walk about in with shirtsleeves and the occasional replenishment of oxygen (via native rocks that burn like coal), and open caves that harbor both liquid water and edible sausage-like plants.
Later on in Robinson, things really take a turn towards the fantastical when our hero encounters humanoid aliens from Alnilam, the bright blue supergiant middle star in the Belt of Orion, extraterrestrial spaceships with blasting ray weapons who utilize organic slaves for mining the Red Planet, and a huge natural subsurface canal system that connects to the polar cap.
It was also pleasing to learn that Conquest inspired a number of other science fiction films and media with its styles and scenes, including the one that is often considered the best of them all, 2001: A Space Odyssey. I did not use this film at the beginning of my essay to describe how Conquest could have been for nothing.
If all else fails, let us agree that Conquest of Space is a representation of how certain folks in circa 1955 imagined we would be heading out into the void, flaws and all. The film has made its positive contributions to both the real Space Age and science fiction cinema, enough so that I think more attention and better documentation of its history and those who worked on it need to be conducted by the space and cinema historians and scholarly science fiction communities. This must be done so that Conquest does not disappear into space and time, for it is part of our human culture.
References
The following hyperlinks take you to selected articles, news, and other media for your further appreciation and enjoyment of the 1955 film Conquest of Space. These links were functional at the time of this essay’s publication.
Here are two links to the original theatrical trailer for Conquest. In fact, there are two trailers in these offerings: The first is in color and the second in black-and-white, with the latter showing via newspaper headlines that George Pal’s cinematic prediction of satellites circling Earth, once considered “impossible”, is about to come true!
As the color trailer declares, in all capital block letters, Conquest is “the biggest true story of our century – before it actually happens!” The narrator assures us that what will see may not happen tomorrow, or next year, but definitely “sometime before the year 2000 A.D.!”
https://archive.org/details/conquest-of-space
https://www.dailymotion.com/video/x2838kc
The complete film is available online for free and uncut at these sites:
https://archive.org/details/ConquestOfSpace1955
https://www.dailymotion.com/video/x1zz5ox
This site contains the pressbook for the premiere of Conquest of Space:
https://www.zomboscloset.com/zombos_closet_of_horror_b/2015/12/pressbook-conquest-of-space-1955.html
Interesting items of note: The pressbook declares that a satellite could be put into space by 1957. They turned out to be right, they probably just expected it to be by the United States and not the USSR. Another blurb also states 1960 as the year of Conquest of Space, following some other notable achievements like the launch of the first nuclear-powered submarine in 1955. The pressbook also emphasizes the film’s declaration that settling space and mining other worlds for resources are the keys to humanity’s survival.
Here are two links to the transcript for Conquest. As I mention in the essay section “Backdraft”, they only show the dialogue from the released film without any background notes, stage directions, or who said what:
https://www.scripts.com/script/conquest_of_space_5876
https://subslikescript.com/movie/Conquest_of_Space-47947
It has been said that Stanley Kubrick was inspired by certain scenes from Conquest for his science fiction masterpiece, 2001: A Space Odyssey. This video wonderfully encapsulates that idea, comparing scenes from the two cinematic works, and is set to The Blue Danube waltz by the Austrian composer Johann Strauss II (1825-1899), composed in 1866:
https://www.youtube.com/watch?v=D9rFlvyW_Rg
Author Roy Kinnard wrote an in-depth look at Conquest for the June 1979 issue of Fantastic Films: The Magazine of Fantasy & Science Fiction in the Cinema, Volume 2, Number 2 (Chicago: Blake Publishing Corporation). The article, titled “A New Look at an Old Classic: Conquest of Space”, includes a comparison with 2001 and may be read online here, starting on page 48:
https://archive.org/details/Fantastic_Films_v2_002_1979_HL3/mode/2up
The following are links to articles, books, and videos on events and plans that influenced Conquest of Space and give one an idea on the contemporary thinking of relevant subjects:
Wernher Von Braun’s The Mars Project, 1953 edition:
https://archive.org/details/TheMarsProject-WernherVonBraun1953
“The Mars Project, 1948 to 1956” by A. A. Jackson:
http://www.aiaahouston.org/Horizons/Pages_by_Al_Jackson_from_Volume_52_AAS_History_Series_Digital_for_IAC_2019.pdf
“Wernher von Braun’s Ultimate Weapon”, by Michael J. Neufeld. Bulletin of the Atomic Scientists Volume 63, Issue 4, July 2007, Pages 50-78:
https://journals.sagepub.com/doi/epub/10.2968/063004019
“THE CHALLENGE OF OUTER SPACE” 1955, MILITARIZATION OF SPACE, WERNHER VON BRAUN LECTURE FILM 99924:
https://youtu.be/JK4RbeBxqso
Johns Hopkins Science Review: Man Will Conquer Space (Parts 1-3). October 6, 13, and 20, 1952. Live from WAAM in Baltimore, Maryland, over the DuMont Network…
Man Will Conquer Space 1952, Part 1, John Hopkins University:
https://www.youtube.com/watch?v=2032odn7eB0
Man Will Conquer Space 1952, Part 2, Rockets and Newtons 3rd Law, John Hopkins University:
https://www.youtube.com/watch?v=Eqf0I5GQM9w
Man Will Conquer Space 1952, Part 3, Werner Von Braun, John Hopkins University:
https://www.youtube.com/watch?v=S34UFqz8GlM
Eyes on the Red Planet: Human Mars Mission Planning, 1952-1970, by Annie Platoff, NASA/CR-2001-208928:
https://web.archive.org/web/20100531192655/https://ston.jsc.nasa.gov/collections/TRS/_techrep/CR-2001-208928.pdf
Humans to Mars: Fifty Years of Mission Planning, 1950—2000, by David S. F. Portree, Monographs in Aerospace History #21, NASA SP-2001-452:
https://history.nasa.gov/monograph21.pdf