by Paul Gilster | Nov 8, 2005 | Astrobiology and SETI, Exoplanetary Science |
If you’re looking for life similar to Earth’s — based, that is, on carbon chemistry and water — you have to determine what kind of stars might have produced such planets. Certain factors weigh heavily in this analysis. The star must be a long-lived, solar-type star with constraints on its luminosity; it must offer an environment within which a planet with liquid water at its surface can exist. This Continuously Habitable Zone (CHZ) is defined this way in a new paper called “Astrobiologically Interesting Stars within 10 parsecs of the Sun,” now available on the arXiv site:
The inner edge of habitability is the region where water is lost through photolysis and hydrogen escape; the oceans simply evaporate;
The outer edge of habitability is the region where C02 clouds form, cooling the planet by lowering its albedo.
Also critical is planetary mass. A reasonable upper limit on mass seems to be a few Earth masses; planets larger than this are likely to be entirely covered with oceans, disrupting the carbonate-silicate cycle vital to atmospheric stability. Planetary mass is related to stellar metal content, and also to the mechanics of the planetary formation process, which leaves us with the uncomfortable realization that we know all too little about how Earth-like planets form.
The authors of this paper, Gustavo Porto de Mello, Eduardo Fernandez del Peloso and Luan Ghezzi (Observatório do Valongo, Brazil), argue that the Continuously Habitable Zone will be found around mid-K to late-F type stars. They go on to make some fascinating observations about how stellar type may affect Earth-like planet formation. M-type stars, for example, put their planets inside the tidal lock radius if they are to be warm enough to have liquid water at the surface, creating potential problems for climate stability. Mid-F type stars have wide habitable zones, but these zones move outward rapidly as the star evolves into subgiant status, leaving a time for development — less than 4 billion years — perhaps too short for the evolution of complex life.
Also considered is the orbit of stars around the galactic core. From the paper:
The stellar Galactic orbit is a rarely mentioned factor of stability which is drawing more interest as one regards how important it may be concerning the average interval between global catastrophes that a planet may undergo. The Sun lies very near the so-called co-rotation radius (Balázs, 2000, Lépine et al., 2001), where stars revolve around the Galaxy in the same period the density wave perturbations sweep across the Galactic disk. In such a situation the passages across the spiral arms, and consequently the potential encounters with star-forming regions and giant molecular clouds, are presumably minimized. The first are thought to present the danger of biologically lethal X- and gamma-ray irradiation episodes by supernova explosions (Gehrels et al., 2003); the latter, to trigger heavy cometary bombardment in the inner planetary system by perturbing the Oort cloud dormant population (Clube & Napier, 1982). Leitch & Vasisht (2001) have presented some evidence on the correlation of past significant mass extinctions with crossings of the spiral arms by the Sun.
All these criteria go into the paper’s analysis of nearby stars that are suitable for the development of complex life. Those stars that have a reasonable habitable zone (and one which is old enough to have maintained a habitable planet within the last few billion years) are likely to be interesting targets for upcoming interferometer studies in the infrared. Terrestrial Planet Finder is designed to be such a mission, and it is noteworthy that the New Worlds Imager concept discussed in an earlier post seems capable of making the initial spectroscopic studies of terrestrial planet atmospheres without the need for multiple spacecraft. Alternative proposals for the mission would require formation-flying spacecraft for such studies and would likely add a decade to the possible launch window.
The authors find 13 good candidates for nearby ‘biostars’ with three ranking especially high: HD 1581 (Zeta Tucanae), HD 109358 (Beta Comae Berenicis) and HD 115617 (61 Virginis): “We suggest these objects as high priority targets in SETI surveys and in future space interferometric missions aiming at detecting life by the ozone atmospheric infrared biosignature of telluric planets.” Future research should weigh more detailed models of planetary climate stability and (relatedly) a possible reassessment of M-class stars if tenable ecosystems seem to be allowed. And Centauri Dreams strongly seconds the paper’s emphasis on more intense study of galactic orbits, which may be the largest constraint of all on habitability.
A useful exercise: run the biostar candidates through the index of Notable Nearby Stars at the SolStation site, where the details of recent observational work are discussed.
by Paul Gilster | Nov 7, 2005 | Breakthrough Propulsion |
What could inflation theory have to do with the Fermi paradox? Quite a lot, if at least one recent paper is to be believed. The question ‘where are they’ about extraterrestrial visitation becomes even more pointed when faster-than-light technologies move out of the realm of the impossible to something that may be seriously investigated by physicists. Inflation theory, which holds that the early universe underwent a vast expansion as spacetime itself stretched far beyond the velocity of light, opens the door to technologies that might use this effect to create spacefaring civilizations spanning entire galaxies.
Just how fast did inflation occur? In a space of time lasting about 10-35 seconds, the universe could have expanded by a factor of 1030 to 10100. As Brian Greene puts it in The Fabric of the Cosmos:
An expansion factor of 1030 — a conservative estimate — would be like scaling up a molecule of DNA to roughly the size of the Milky Way galaxy, and in a time interval that’s much shorter than a billionth of a billionth of a billionth of the blink of an eye… In the many models of inflation in which the calculated expansion factor is much larger than 1030, the resulting spatial expanse is so enormous that the region we are able to see, even with the most powerful telescope possible, is but a tiny fraction of the whole universe.
Enter Bernard Haisch (National Aviation Reporting Center on Anomalous Phenomena), Hal Puthoff (Institute for Advanced Studies at Austin) and colleagues. The authors argue in a recent issue of the Journal of the British Interplanetary Society that while General Relativity is valid, there are several approaches within it that may permit bypassing the speed of light limit (even if any civilizations that could build them might have to be more advanced than ours by millions of years):
The use of wormholes, engineered through the use of exotic matter. The mathematical requirements for creating a traversable wormhole have entered the scientific literature in the works of, among others, Kip Thorne.
The Alcubierre warp drive concept, which notes that there is no limit to the speed at which space itself might stretch. Faster than light relative motion is built into inflation theory. Alcubierre’s work shows that a spacecraft could theoretically make use of expanding spacetime behind and a similar contraction in front of the vehicle to overcome the restrictions of General Relativity.
Superstring theory suggests the possiblity that adjacent universes could be all around us. The added dimensionalities of M-brane and superstring theory might allow a sufficiently advanced technology to move into an adjcent universe where the speed of light limit is different than our own.
From the paper:
Clearly when it comes to engineering warp drive or wormhole solutions, seemingly insurmountable obstacles emerge, such as unattainable energy requirements or the need for exotic matter. Thus if success is to be achieved, it must rest on some yet unforeseen breakthrough about which we can only speculate, such as a technology to cohere otherwise random vacuum fluctuations. Nonetheless, the possibility of reduced-time interstellar travel by advanced extraterrestrial (ET) civilizations is not, as naive consideration might hold, fundamentally ruled out by presently known physical principles. ET knowledge of the physical universe may comprise new principles which allow some form of FTL travel. This possibility is to be taken seriously, since the average age of suitable stars within the ‘galactic habitable zone’ in which the Earth also resides, is found to be about 109 years older than the Sun…
Is the answer to the Fermi paradox, then, that we are even now being visited by extraterrestrial spacecraft whose contact strategy is based on a sense of interstellar ethics we do not yet understand? The paper argues that such visitations must be considered more likely than a ‘we are alone’ answer to the Fermi question, and goes on to consider what ethical considerations might motivate a culture investigating our planet from outside Earth. The paper is Deardorff, Haisch, Maccabee and Puthoff, “Inflation-Theory Implications for Extraterrestrial Visitation,” in the Journal of the British Interplanetary Society Vol. 58, No. 1/2 (January/February 2005), pp. 43-50.
by Paul Gilster | Nov 5, 2005 | Breakthrough Propulsion |
When physicist Geoffrey Landis reviewed interstellar concepts at the American Association for the Advancement of Science’s 2002 meeting, his wide-ranging presentation considered where we stand on nuclear propulsion, solar and lightsail technologies, and particle-pushed sails. He also addressed the question of the Bussard ramjet, which would use an electromagnetic scoop to collect atoms from the interstellar medium to fuel a fusion reactor. Finding serious problems here (he cites, among other things, the fact that the scoop technology acts more like a brake than an accelerator), Landis went on to consider an alternative:
“These problems can be alleviated if, instead of using the ambient interstellar medium, fuel is deliberately emplaced in the path of the spacecraft before flight. In this way, the fuel (probably in the form of small ‘pellets’) can be chosen to be the optimum composition…
The ‘runway’ of fuel pellets could be emplaced, for example, by a dedicated craft which drops fuel pellets at predetermined locations along the flight path. Each ‘fuel pellet’ would have to contain more than the fusion fuel; at a minimum the pellets would have to contain passive locator beacons. The interstellar probe would adjust its trajectory as required to ingest and utilize each successive fuel pellet. Alternately, and possibly more realistically, the fuel pellets themselves would each have a small amount of propulsion capability, enough for them to station-keep in a predetermined location, for example, maintaining position along a laser used as a guide-beam.
[Jordin] Kare noted that, at the velocities proposed for interstellar flight, nuclear fusion can be accomplished at the temperatures produced by impact. If a small pellet carried on the vehicle impacts a stationary pellet of fusion fuel, the result of the impact will be ignition of the fusion reaction, and potentially the liberation of a considerable amount of energy…”
When Centauri Dreams talked to Landis in 2003 about the fusion runway concept (Kare calls the idea the ‘Bussard Buzz Bomb’), the physicist elaborated on why he found the concept so intriguing. Kare’s work had indicated that it would take a velocity of 200 kilometers per second to light the starship’s main engines using these techniques. Landis pointed out that a spacecraft making a close pass by the Sun (virtually skimming the solar surface) could reach 600 kilometers per second, with the fuel pellets lined up so that the vehicle could start using them immediately after the solar pass.
How long would a fusion runway have to be to function? The answer depends on your spacecraft. An unmanned robotic probe that could endure intense gravitational forces would require a much shorter runway. If the craft is manned, the pellets would have to be strung out over a tenth of a light year to allow the probe to reach a cruising velocity of 30,000 kilometers per second. That translates into a forty-plus year journey to the Centauri stars.
Landis’ AAAS presentation is available as “The Ultimate Exploration: Approaches to Interstellar Flight,” in Y. Kondo, ed., Interstellar Travel & Multi-Generational Space Ships (Apogee Books, 2003).
by Paul Gilster | Nov 4, 2005 | Deep Sky Astronomy & Telescopes |
We can measure interstellar distances, but can we really grasp them? The distance to the nearest stars is so immense that even the scientists who study such things have resorted to homely comparisons. The most charming to my mind is that of the English astronomer Sir John Herschel (1792-1871), the son of the famous William Herschel who discovered Uranus. A wizard at mathematics, Herschel became a leading expert on double stars and the measurement of stellar distances through parallax (the apparent change in position of a nearby star against background stars due to the Earth’s changing orbit around the Sun).
When it came to the distance to the Alpha Centauri stars, Herschel saw things in terms of ocean voyaging, thinking himself standing on shipboard dropping peas into the water. As he once wrote, “. . . to drop a pea at the end of every mile of a voyage on a limitless ocean to the nearest fixed star, would require a fleet of 10,000 ships of 600 tons burthen, each starting with a full cargo of peas.” Herschel’s fascination with double stars led to a catalog of such stars published in the Transactions of the Royal Society, and his later work on determining their orbits around a common center of gravity was honored by the Royal Society in 1833.
But back to the long road to Alpha Centauri. The useful HyperPhysics site at Georgia State University has a page that puts the Centauri distance in more abstract terms than Herschel. Imagine the Sun scaled down to the size of a period on a printed page. On this scale, the distance to Alpha Centauri is 13.6 kilometers (8 miles).
Now change the perspective again. Suppose this time it’s the Earth that is the size of a period on a printed page (which is, by the way, about 0.5 mm). On that scale, the Sun would be a little smaller than a tennis ball at a distance of some 5.9 meters (19 feet). The Alpha Centauri A and B stars would lie roughly 890 miles away.
Now think about this one: the parallax of Proxima Centauri, the M-class red dwarf that apparently orbits Centauri A and B at about 10,000 AU, is about equivalent to that of a dime at a distance of 6 kilometers. Proxima is so faint that astronomers on a planet orbiting either Centauri A or B would probably not realize it was nearby until they took measurements of its own parallax. GSU provides a helpful backgrounder on parallax, noting its use in measuring the distance to the few stars that are close enough to the Sun to show a measurable parallax. The limit of this measurement is about 20 parsecs, which includes some 2000 stars.
by Paul Gilster | Nov 3, 2005 | Deep Sky Astronomy & Telescopes
The Spitzer Space Telescope once again dazzles us with its capabilities at infrared wavelengths. Now it’s the detection of what may be some of the earliest objects in the universe, the hypothetical Population III stars that would have formed a mere 200 million years after the Big Bang itself. These short-lived objects were probably over a hundred times more massive than our Sun. If the scientists investigating the recent Spitzer data are right, they are looking at the redshifted ultraviolet light of these ancient stars, stretched to lower energy levels by the expansion of the universe and now detected as a diffuse glow of infrared light.
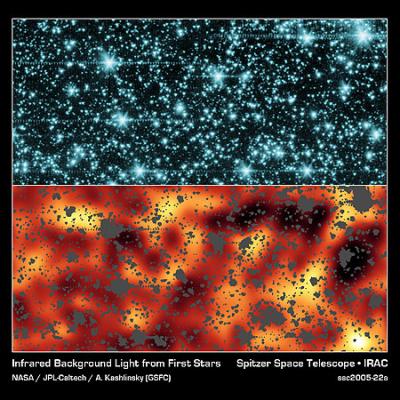
Image: The top panel is an image from NASA’s Spitzer Space Telescope of stars and galaxies in the constellation Draco, covering about 50 by 100 million light-years (6 to 12 arcminutes). This is an infrared image showing wavelengths of 3.6 microns, below what the human eye can detect. The bottom panel is the resulting image after all the stars, galaxies and artifacts were masked out. The remaining background has been enhanced to reveal a glow that is not attributed to galaxies or stars. This might be the glow of the first stars in the universe. Credit: NASA/JPL-Caltech/A. Kashlinsky (GSFC).
Described in the November 3 issue of Nature, the detection of objects in the universe of 13 billion years ago is remarkable in itself, and so is the method the science team, based at NASA Goddard Space Flight Center in Greenbelt (MD) used to make the observations. We are talking about light that is far to faint to be resolved into individual stars. Spitzer was therefore used to take a ten-hour observation in the constellation Draco. The team then eliminated all known objects to study the far more ancient light left behind. Alexander Kashlinsky, an astronomer at NASA’s Goddard Space Flight Center in Greenbelt, Maryland, and a member of the team that made the discovery, calls this afterglow “…the repository of emissions for all stellar populations that have ever existed in the Universe.”
From a Spitzer news release:
“This deep observation was filled with familiar-looking stars and galaxies,” said Dr. John Mather, senior project scientist for James Webb Space Telescope and a co-author on the Nature article. “We removed everything we knew—all the stars and galaxies both near and far. We were left with a picture of part of the sky with no stars or galaxies, but it still had this infrared glow with giant blobs that we think could be the glow from the very first stars.”
And here is where the various scientific resources come together. NASA’s Cosmic Background Explorer had produced data suggesting an infrared background glow that was not associated with known stars. Later, the Wilkinson Microwave Anisotropy Probe’s 2003 data pointed to ignition of the earliest stars in an era between 200 and 400 years after the Big Bang. Now we can look forward to integrating the data from COBE, WMAP and Spitzer with upcoming information from the James Webb Space Telescope, which may one day resolve the first individual clusters of such stars.
Centauri Dreams note: It is important to emphasize that the new Spitzer observations reveal not individual stars but variations in background radiation that seem to point to star formation. Nonetheless, the data seem clean, as discussed in this nature.com article from which the above quote from Alexander Kashlinsky comes. In fact, the team found clusters in the background radiation in four different wavelengths in different parts of the sky and at different times of year, which seems to rule out local interference.
The paper itself is Kashlinsky, A., Arendt, R.G. et al., “Tracing the first stars with fluctuations of the cosmic infrared background,” Nature 438, 45-50 (3 November 2005). Also, Juan Cabanela at Saint Cloud State University (MN) offers a fine discussion of the first stars in terms of hydrostatic equilibrium.