by Paul Gilster | Mar 20, 2006 | Deep Sky Astronomy & Telescopes
Centauri Dreams follows studies about dark matter with great interest, given the mysterious nature of the stuff and the fact that it apparently has so much to say about how galaxies form. One way to get a handle on dark matter is to study the positions and velocities of stars in the galaxies themselves, thus learning what forces must account for them and how unseen matter may be affecting where they travel.
A new study in this vein has now uncovered a ‘river’ of stars of considerable size that arcs over the disk of the Milky Way. “We were blown away by just how long this thing is,” says Carl Grillmair, an associate research scientist at the California Institute of Technology. “As one end of the stream clears the horizon this evening, the other will already be halfway up the sky.”
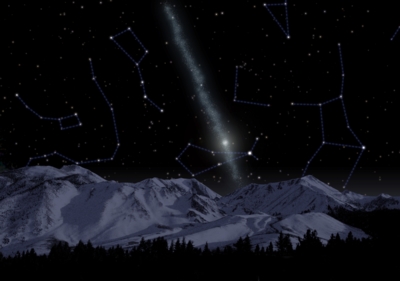
Image (click to enlarge): On an evening in early April, the new stream rises 45 degrees from the eastern horizon, passing just under the bowl of the Big Dipper. The North Star Polaris is at far left.” Credit: California Institute of Technology.
Grillmair, who is co-author of a paper on the stream that is about to appear in the Astrophysical Journal Letters, has good reason to be amazed. The star stream begins just south of the bowl of the Big Dipper and continues to a point about 12 degrees east of Arcturus in the constellation Bootes. It emerges from a cluster of some 50,000 stars known as NGC 5466; tidal forces appear to be stretching the cluster, which would otherwise be largely spherical, and the outlying stars are drifting into their own orbits, both ahead and behind the cluster.
To find the stream, Grillmair and graduate student Roberta Johnson studied the color and brightness of more than 9 million stars in the Sloan Digital Sky Survey public database. “The new stream may be even longer than we know, as we are limited at the southern end by the extent of the currently available data,” Grillmair adds. “Larger surveys in the future should be able to extend the known length of the stream substantially, possibly even right around the whole sky.”
But don’t expect to see the star river with the unaided eye. The stars that make it up are said to be about three million times fainter than the dimmest stars you can see on a clear night. What counts, though, is that astronomers may be able to use it as another clue that will help reveal how much dark matter the Milky Way contains, and whether that matter is distributed evenly or in clumps. Centauri Dreams would not be surprised to see more such formations emerge as we gain the tools to discern their presence through the intervening galactic disk.
by Paul Gilster | Mar 18, 2006 | Deep Sky Astronomy & Telescopes
Inflation has always been hard to get one’s thoughts around. If nothing can travel faster than light, then how can the universe itself have expanded from submicroscopic to astronomical size in mere moments? The solution — that while nothing can move through space faster than light, space itself knows no such restriction — is still unsatisfying, for we don’t understand how inflation happens, or how it may still affect the accelerating expansion of today’s universe.
The best recent work on these matters has been done through analysis of data from the Wilkinson Microwave Anisotropy Probe (WMAP), using three years worth of observations of the cosmic background radiation. The temperature of that afterglow tells us much about the universe’s age and how it developed, and WMAP can read such temperature fluctuations down to grades finer than a millionth of a degree. The new measurement gives us clues not only about that crucial first trillionth of a second, but also about how and when the first stars began to form. The pattern being studied is less than a hundredth of the strength of the temperature signal that made news three years ago when the early WMAP results came in.
The tiny patterns discernible in the WMAP data, it is believed, eventually emerged into galaxies; at this stage of the infant universe, however, they appear simply as temperature variations within what is otherwise a uniform background light. The new data support the idea that inflation indeed occurred, leading to the formation of the first stars some 400 million years later.
“This is brand new territory,” said WMAP team member Lyman Page of Princeton University in Princeton, N.J. “The polarization data will become stronger as WMAP continues to observe the microwave background. WMAP’s new results heighten the urgency of seeking out inflation’s gravitational wave sign. If gravitational waves are seen in future measurements, that would be solid evidence for inflation.”
So what happened when inflation was triggered? Quantum fluctuations at the subatomic level suddenly became converted into flucuations within matter itself. In a sense, what we see at the largest level in the cosmos around us should, by this theory, reflect the quantum-level characteristics of the early universe, the origin of everything writ large in the sky. And as Page notes above, these fluctuations should also have produced gravitational waves that would have left a signature in the background radiation. Future measurements of the cosmic microwave background from WMAP could confirm inflation, which a mere 25 years ago seemed the wildest of speculations, and now can find support within hard mission data.
Centauri Dreams‘ note: The model that is being confirmed by continuing WMAP analysis is that the normal matter we see around us makes up only 4 percent of the total. It is now thought that 22 percent is in the form of the mysterious dark matter that has yet to be identified, while fully 74 percent exists as dark energy, equally mysterious, and apparently causing a new acceleration, though on a far more sedate scale than the one at the beginning of time. Given that we have a reasonable handle on a mere 4 percent of what makes up the universe, doctrinaire statements about the nature of reality seem increasingly inadvisable!
For more on the WMAP results, click here. These results have also been submitted to the Astrophysical Journal.
by Paul Gilster | Mar 17, 2006 | Deep Sky Astronomy & Telescopes, Exoplanetary Science
Making accurate measurements of distant binary objects isn’t easy, but it helps when the two targets are edge-on as seen from Earth. That sets up an eclipsing binary, and in the case of a newly discovered duo of brown dwarfs in the Orion Nebula, provides helpful information. We now know that the the larger of the two brown dwarfs is 55 times the mass of Jupiter, while the smaller is 35 times larger (with a 10 percent margin of error in the calculation). Such measurements help firm up theoretical models of brown dwarf formation and composition.
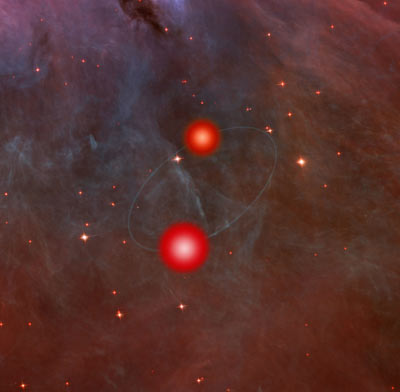
Image: An artist’s conception of a pair of eclipsing brown dwarfs. Credit: NASA, ESA, and A. Feild (STScI).
“This binary pair is a ˜Rosetta stone’ that will help unlock many of the mysteries regarding brown dwarfs,” says Keivan Stassun, assistant professor of astronomy at Vanderbilt University, who led the team of astronomers who made the new observations. “We understand how stars form in the crudest sense: They are formed when clouds of dust and gas collapse. But many of the details of the process remain a mystery, particularly the factors that determine what a star will weigh.”
The findings mark the first time we’ve measured the mass, size and surface temperature of brown dwarfs, a major stride in their study. The cutoff point below which a brown dwarf cannot be a hydrogen-burning star is 80 Jupiter masses, which is large enough to provide nuclear fusion (by comparison, the Sun is 1000 times the mass of Jupiter). And the odd thing about the new measurements, which are reported in the journal Nature this week, is that the larger dwarf is cooler than the smaller. As you would expect, this finding contradicts previous models and may mean the two are not the same age. Stassun again:
“One possible explanation is that the two objects have different origins and ages. If that is the case, then it supports one of the outcomes of the latest efforts to simulate the star-formation process. These simulations predict that brown dwarfs are created so close together that they are likely to disrupt each other’s formation.”
Centauri Dreams‘ take: Brown dwarfs become increasingly interesting as we realize they may be far more common than first thought. Indeed, the Nature article supports the notion that dwarfs begin as star-sized objects but shrink and cool as they age; we may have been observing numerous brown dwarfs without realizing their actual nature. The new observations provide hard data to refine our models. Although their small size and dim light make them hard to detect, brown dwarfs closer than the Centauri stars may eventually be found, a fascinating consideration as we ponder future missions.
The paper is Stassun, K., Mathieu R. and J. Valenti, “Discovery of two young brown dwarfs in an eclipsing binary system,” Nature 440, pp. 311-314 (16 March 2006). A multimedia version of the story is available on Exploration, Vanderbilt University’s online research magazine.
by Paul Gilster | Mar 16, 2006 | Exoplanetary Science |
A recent query about how astronomers work out the mass and radius of planets found through microlensing — such as the so-called ‘super-Earth’ recently discovered 9000 light years from our Sun — prompted Centauri Dreams to query one of the principals in that discovery. Andrew Gould, leader of the MicroFUN collaboration and professor of astronomy at Ohio State University, was kind enough to clarify how this fascinating science proceeds. Herewith his response:
We obtain a planet-star mass ratio from a fit to the light curve. This parameter (q) is a standard output from fits to microlensing curves generated by binary lenses (two point masses, e.g., star and planet). Now, for low-magnification microlensing events, such as OGLE-2005-BLG-390Lb (which was announced by the PLANET team in January), it is generally possible to estimate the mass just by looking at the light curve. And in those cases it can be explained easily to the non-expert (although PLANET did not do this in their article). However, in high-magnification events like OGLE-2005-BLG-169Lb, we get a mass ratio out of our computer codes, but there is no known simple way to make an estimate directly by looking at the light curve.
We estimate the star mass M based on some characteristics of the event plus a Galactic model. Thus we derive a planet mass, m = qM. We will be able to do better on the star mass when we get images of the host star as it moves away from the source (assuming we can get Hubble time for this).
We have no direct information about the radius. In the article, we put forward two ideas about the nature of the planet. One is that it is like Neptune, with a rock and ice core surrounded by a thick padding of gas. Then it would presumably have a radius like Uranus and Neptune, about 4 earth-radii. However, because it is in the region of its solar system that is just out past the “snow line” (where Jupiter and Saturn are in our solar system), we prefer the idea that it is a failed core of a Jupiter-like planet. Then it would be all rock and ice, probably roughly 2 gm/cm³ uncompressed (and so about 2.5 gm/cm³) compressed. This would give it a radius of about 3 earth-radii.
On the matter of terrestrial-size worlds, Gould added that he was hopeful that such planets could be detected within a few years, but noted that they would still be 10,000 to 20,000 light years away. Thus the beauty, and frustration, of microlensing. It seems the most likely way we’ll find our first Earth-mass planets, but the physics involved all but guarantees they’ll be distant indeed.
by Paul Gilster | Mar 15, 2006 | Outer Solar System |
There seems to be an emerging maxim in deep space studies: every new mission will overturn at least one enshrined assumption Thus the early Stardust results, studying the cometary debris from Wild 2. Because comets come out of the outer dark in their long arc toward the inner system, one would expect them to be made of materials that were born in cold temperatures. But Stardust has brought us cometary dust that’s packed with minerals formed at high temperatures.
What a fascinating set of challenges now face the Stardust researchers. They’ve found olivine in the Wild 2 materials; it’s a compound of iron, magnesium and various other things (the Stardust sample is primarily magnesium). Most astronomers believe that olivine crystals are formed from glass that has undergone heating near stars. So how crystals of olivine can show up in the Wild 2 samples bears scrutiny — after all, Wild 2 is thought to have formed well beyond the orbit of Neptune.
And olivine isn’t the only oddity about the Stardust samples. They also contain high-temperature minerals that are rich in calcium, aluminum and titanium. The message seems clear, as stated by principal investigator Donald Brownlee (University of Washington): “I would say these materials came from the inner, warmest parts of the solar system or from hot regions around other stars.”
About 150 scientists around the world are working on the Stardust samples. They’re studying grains that are embedded in the aerogel used to trap them during the mission’s encounter with the comet in January of 2004. And there’s no shortage of material to work with, since thousands of cometary grains are now available. Brownlee notes that his laboratory has worked on only two particles so far, and has yet to cut into the main part of the first of them.
Centauri Dreams‘ take: We now have samples from the farthest regions of the Solar System that were clearly formed at high temperatures. So perhaps we’re looking at a formation mechanism for planetary systems that involves a constant flow of materials from the center to the outer regions. Or — and this is just as interesting a possibility — it may be that cometary materials like these actually came from other solar systems through transfer mechanisms yet to be explained. Much remains to be done, especially with regard to possible organic materials in the Stardust grains. The payoff just might be a revision of key planetary formation theories. We’ll keep a close eye on this one.