by Paul Gilster | Apr 21, 2007 | Sail Concepts |
We’re at such an early stage in solar sail development that it will not be surprising if laboratory results lead us in entirely new directions. Consider James Benford’s work at the Jet Propulsion Laboratory, where he and brother Gregory experimented with an ultralight 7.5 g/m2 carbon sail to test out microwave beam concepts. If the Planetary Society’s Cosmos 1 sail mission had been successful, the Benfords would have been involved in a microwave experiment using the Deep Space Network’s Goldstone antenna, a beamed propulsion test that a failed booster precluded.
But the JPL work did lead to the interesting phenomenon of sublimation (also known as desorption). Put a microwave beam on the sail and you wind up with more acceleration than you expect. It’s the result of the evaporation of absorbed molecules from the hot side of the sail. In a 2005 paper in Acta Astronautica that we reviewed in these pages, the Benfords looked at putting desorption to work by painting a sail with a compound whose desorption properties are known and can give the sail an extra boost. A microwave beamed sail might be boosted out of low Earth orbit and, having expended its desorbed materials, spend the rest of an interplanetary journey operating as a normal solar sail.
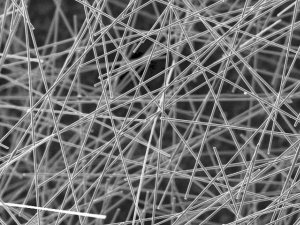
Giving the idea punch is the Benford’s estimate that a desorbing sail under microwave beam might make the journey to Mars in no more than a month. But doesn’t desorption produce damage to the sail material? That question has come up in earlier discussions here, and James Benford has been kind enough to answer it by sending along these photographs.
In the image above, you’re looking at the front of the sail material used in the Benford’s JPL experiment; the second image shows the back of the same sail.
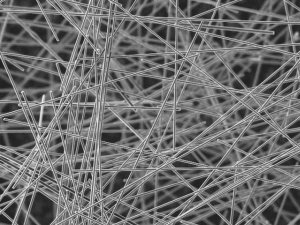
Let me just quote from the material Benford sent to describe what we’re looking at:
The experimental data don’t show sail damage from desorption. The molecules are not an integral part of the lattice, but embedded in it during its fabrication. If damage were to occur due to desorption, one would expect that the nodes where the excess heating occurs to have a changed appearance, such as singeing and blackening. No such signs are evident from the microphotography of the sails, however.
Image: Front (above) and back (below) for sail #1 post-flight. No local burning of the sail is evident. Nor is there mass loss from weighing. The sail accelerated at about 2 gees. Credit: James Benford.
The conclusion is that asymmetric (one-sided) sublimation pressure is a viable propulsion mechanism for microwave-driven sails. Based on thermal diffusion calculations, here is a clear case for a temperature gradient to support differential evaporation.
Note that laser/sail interactions are very different because the far larger frequency gives a skin depth ~1% of the sail thickness. This can support large temperature differences and large sublimation pressure effects; Leik Myrabo has observed them to onset at ~0.1 gee. Since skin depth scales as the square root of frequency and the laser work is four orders higher in frequency, his skin depth must be <1% of the sail thickness, so ablation will certainly occur. More generally, sublimation could be a severely limiting factor for lasers with sails.
That last comment is interesting. Looking at sublimation as a propulsion mechanism reveals it as a potential problem for laser sail concepts while an advantage in the microwave realm. Moreover, microwaves are not only less damaging to sail materials but more efficient in their ability to heat the sail. Add to this that lasers experience atmospheric distortion problems that microwaves do not. The conclusion: beaming to a sail in low Earth orbit from the ground is more feasible in the microwave realm. And that may one day make a fast sail to Mars a reality.
by Paul Gilster | Apr 20, 2007 | Exoplanetary Science |
Investigating brown dwarfs is not for the faint of heart. With a mass below what’s needed to sustain hydrogen-burning fusion, they’re hard to see, and they may be far more numerous than we’ve previously estimated. Nor do we have a good handle on how they function, to judge by a new study showing that these objects can possess powerful magnetic fields. It turns out that the ‘failed star’ designation may be a bit inapt. Sure, they’re dim objects but in recent years, it has been discovered that some brown dwarfs put out beams of radio waves that are thousands of times brighter than any detected from our Sun.
Brown dwarfs, in other words, are actually pretty lively. If you think about other sources of flashing radio signals, pulsars come immediately to mind. Their powerful magnetic fields and incredibly fast rates of rotation make figuring out how they produce their signals a difficult matter. Now we find brown dwarfs emerging as a second class of stellar objects that produce such powerful and persistent radio signals. But their emissions come from much slower systems, somewhat easier for astronomers to model and showing similarities to radio emissions generated by planets like Jupiter.
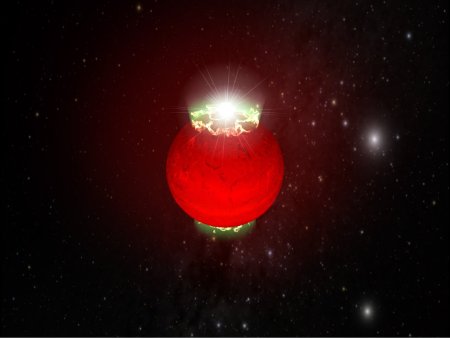
Image: Artist’s impression of the “super-aurorae” present at the magnetic poles of these radio emitting dwarfs that are responsible for the radio pulsations. Credit: Gregg Hallinan/National University of Ireland.
The new work rules out the processes of radio emission found in the Sun — the brown dwarf pulses are far too bright to be explained in this way. What brown dwarfs seem to represent, in fact, is a kind of missing link between pulsar-style emissions and those we find around Jupiter (the giant planet’s emissions can sometimes exceed those of the Sun). Says Gregg Hallinan (National University of Ireland, Galway):
“Brown dwarfs tend to be seen as a bit boring – the cinders of the galaxy. Our research shows that these objects can be fascinating and dynamic systems, and may be the key to unlocking this long-standing mystery of how pulsars produce radio emissions.”
Of the three brown dwarfs Hallinan’s team studied with the Very Large Array, the dwarf TVLM 513 points best to the similarity between what we see at Jupiter and the distant pulsar flashes. One possible source of the Jovian emissions: rapid rotation that promotes electron acceleration when electrically charged gas from Io is accelerated by the planet’s magnetic field. The observed radio ‘flash’ around the brown dwarf occurs every 1.96 hours, but there seems to be no binary system here, nor have any planets been detected. Just what makes the high energy electrons accelerate into the dwarf’s magnetic poles remains a mystery.
These radio emissions are powerful enough to flag the size of the brown dwarf’s magnetic field. So what percentage of known brown dwarfs is a pulsing radio source? That question, now due to be studied by Hallinan’s team, is an important one. Assuming pulsing sources are frequent, they could become the key to detecting more of the difficult to observe dwarfs. That would make brown dwarfs a little less exotic and provide a real boost in fleshing out our catalogue of stellar systems in nearby space.
by Paul Gilster | Apr 19, 2007 | Sail Concepts |
It should come as no surprise that Eric Drexler has an interest in solar sails. Normally thought of for his contributions to nanotechnology, and especially his groundbreaking The Engines of Creation (Anchor, 1986), Drexler once discussed sail technologies in a short essay called “The Canvas of the Night.” Sails present an obvious problem — how do we stow such thin films for launch, then deploy them in space without damage. Wouldn’t these issues be best resolved by building the sails in space? Drexler had this to say about the idea:
Lightsails are what solar sails seem likely to become when we build them in space. They differ considerably from the deployable, plastic-film sails designed for launch by rocket from the ground. Not needing the toughness to survive folding, launch and deployment, lightsail reflectors need no plastic backing tens of thousands of atoms thick: they can be unbacked aluminum films just a few hundred atoms thick. Such thin foil cannot be made by smashing a bar of aluminum between rollers, as kitchen foil is made. Instead, thin films are made by piling up atoms on a smooth surface.
Drexler discusses how to do this using relatively conventional techniques (by nano-technological standards), but goes on to look at laser-driven mesh sails for interstellar missions. Now the tools of new technologies really come into play. “The molecular machines of nanotechnology,” he writes, “building atom by atom, could build such sails by the mile. (They will build much, much more than that, but that is a different story).”
All of this came to mind when reading advanced nanotechnology, where Brian Wang discusses a proposal by Devon Crowe of PSI Corporation (released as a presentation on a Phase I NIAC study from what is presumably that organization’s last meeting). Crowe proposes creating large space structures made out of bubbles or foams that can be made rigid by ultraviolet ‘curing.’ Coating the inside of such a bubble through evaporation could produce reflective sails of significant dimension.
Is this a method for making the kind of lightsail Robert Forward once analyzed as a way of getting payloads and human crews to nearby stars? Crowe envisions bubbles and foam with individual cell sizes up to 100 meters in low Earth orbit. But take the technology into deep space and you can create a single bubble 1000 kilometers wide, with foam structural elements that can be made much larger still. The presentation shows where this leads: “Structural spans could exceed 10,000 kilometers in micro-g environments.”
For some perspective, advanced nanotechnology provides helpful comparisons:
…the size of a 1000 kilometer bubble is nearly the size of Charon, the moon of Pluto. Charon is 1200 kilometers in diameter. Saturn’s moon Tethys is 1050-1080 kilometers in diameter Ceres the largest object in the asteroid belt is 970 kilometers in diameter. A single tesselation foam…of 1000 kilometer bubbles would be about the size of Earth’s moon. A Penrose tesselation…of 1000 kilometer bubbles would be in between the size of Neptune or Saturn. A Tesselation foam of 100 kilometer bubbles in earth orbit could form an object the size of our existing moon or larger.
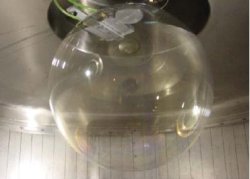
Those who have read Forward’s novel Rocheworld may remember the giant sail the crew used to get to Barnard’s Star, fully 300 kilometers wide. Forward envisioned a 3500 ton payload for that mission, including a habitat supporting twenty crewmembers. He had even worked out a way to stop the sail at destination by separating it upon arrival and letting the outer sail ring reflect Earth-beamed laser light back on the central sail, thus slowing the spacecraft over the course of a year’s time.
Image: Demonstrated 70-cm rigid bubble created in a laboratory vacuum. Credit: Devon Crowe/PSI Corporation.
And how about the Fresnel lens he proposed using to focus the laser over interstellar distances? Made out of concentric rings of one-micron thick plastic film alternating with empty rings, the lens would achieve a diameter of 1000 kilometers. Placed between the orbit of Saturn and Uranus, it would focus the beam so tightly that it would not reach the size of its transmitting aperture until fully 44 light years from its source.
Can Crowe’s ideas be adapted to build such vast structures? If we can find ways to make such things happen through nanotechnological methods, then some of Forward’s seemingly outlandish ideas move into the realm of the plausible. And that’s a heartening thought, because Forward believed in his marrow that interstellar travel was difficult but not impossible, and that human beings would one day make the journey.
I notice that Webster Cash (University of Colorado at Boulder) is interested in seeing this research taken to the next level, though with NIAC gone, Phase II funding is obviously not forthcoming. But how these concepts might fit into the New Worlds Imager mission Cash is designing is an interesting speculation, and one that Crowe examines in his presentation. Major reductions in starshade mass and launch volume could well be attainable.
The Drexler essay ran in the book Project Solar Sail, edited by Arthur C. Clarke (New York: Roc, 1990), 44-45. Also see Drexler’s “High Performance Solar Sail Concept,” L5 News 4 (May 1979): 7-9, which discusses vacuum deposition methods for creating sail films.
by Paul Gilster | Apr 18, 2007 | Communications and Navigation |
Bringing computer networking to space exploration is a major step forward. It allows us to go beyond the old model of pointing radio dishes at a specific spacecraft and downloading information — a time-consuming process as we move from one spacecraft to another — to communicate instead with a single hub vehicle that could be processing data from a cluster of sources. That maximizes precious communications resources here on Earth and allows us to connect planetary rovers, for example, with base stations, orbiting spacecraft and other nearby vehicles.
We’ve talked about interplanetary networking before in terms of the InterPlanetary Internet Project (IPN), a key player in which is Internet legend Vinton Cerf. But extend the idea further, as John Barker (University of Glasgow) is doing today at the Royal Astronomical Society’s national meeting in Lancashire (UK). What Barker has in mind is using ‘smart dust’ — tiny computer chips surrounded by a polymer sheath — to form intelligent swarms for research on a planetary surface.
Here’s how Barker explains the idea:
“We envisage that most of the particles can only talk to their nearest neighbours but a few can communicate at much longer distances. In our simulations we’ve shown that a swarm of 50 smart dust particles can organise themselves into a star formation, even in turbulent wind. The ability to fly in formation means that the smart dust could form a phased array. It would then be possible to process information between the distributed computer chips and collectively beam a signal back to an orbiting spacecraft.”
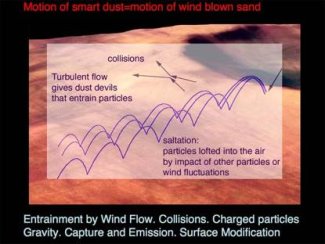
How to control the motion of smart dust in such an environment? The surface of the polymer sheath surrounding the chip can be smoothed or wrinkled depending on the application of a small voltage. Switching between rough and smooth modes adjusts the drag on the particle, allowing it to rise or settle toward the surface. Controlling these modes allows the particles to move toward their target despite ambient weather conditions.
Image: The University of Glasgow team has studied the collective movement of motes towards a target located in a portion of the Martian surface that extends over a range of several kilometers. A smart dust mote has approximately the density and volume of a coarse grain of sand. It follows that the uncontrolled motion of smart dust is determined by the same Aeolian processes of saltation, settling and surface creep that govern the movement of sand in desert regions. Adjusting the surface of smart dust particles provides one way to control their motion. Credit: John Barker/University of Glasgow.
Barker notes a key problem with current technology. Today’s chemical sensors are too large for particles the size of sand-grains that might be carried by the Martian wind for deployment. But the denser atmosphere of Venus could carry particles up to a few centimeters in size. In any case, as we move forward not many years, our chip components should reach sizes of a few nanometers across, making them, in Barker’s thinking, more like molecules diffusing through an atmosphere than dust grains.
Nanotechnology will have an extraordinary effect on space exploration, offering in a few short years options that would otherwise have been impossible. The team at Glasgow knows that and is clearly unafraid to think big. Acknowledging that smart dust is years away from deployment on an actual mission, Barker looks well down the road at interstellar implications: “Our first close-up studies of extra-solar planets could come from a smart dust swarm delivered to another solar system by ion-drive.”
by Paul Gilster | Apr 17, 2007 | Culture and Society |
Centauri Dreams has always admired gutsy women. Dorritt Hoffleit was one of the gutsiest. She spent nearly fifty years at Yale University teaching, doing research and continuing to work in her office into her 90’s. She may have been the world’s oldest active astronomer when she died on April 9 at age 100.
Here’s a bit from the Boston Globe‘s obituary:
As the author of the Yale Bright Star Catalogue, she tracked stars across the sky and published their locations. In her spare time, she studied variable stars, stars that vary in brightness with time, and taught young female astronomy students on Nantucket every summer. Awards and honors began to pile up.
She was awarded the George Van Biesbroeck Prize in 1988 for dedication to astronomy and five years later the American Astronomical Society awarded her the Annenberg Prize for science education. That same year, she was inducted into the Connecticut Women’s Hall of Fame. And still, though she had officially retired at age 68 in 1975, she kept working, often harder than colleagues half her age.
This is a loss for Yale and the astronomy community in general. Dr. Hoffleit leaves, of course, a lasting legacy in the inspiration she provided to her students, no small part of which was the sheer pluck by which she worked her way up in a historically male profession. The changes her 100 years saw both socially and scientifically are little short of astonishing.