by Paul Gilster | Jan 12, 2009 | Exotic Physics |
Place two parallel plates close to each other in vacuum and a strange thing happens, as Dutch physicist Hendrik Casimir learned. The Casimir effect that he described draws the plates together, an effect that was successfully measured first in 1958 and, with greater precision, by Steve Lamoreaux in 1996. The effect becomes important at distances less than 100 nanometers. And if it seems like little more than a curiosity, be aware that Robert Forward looked at the possibilities of engineering to put this energy to use in an intriguing 1984 paper.
That paper (“Extracting Electrical Energy from the Vacuum by Cohesion of Charged Foliated Conductors” — see reference below) looks at the attraction between two parallel plates in a vacuum as the result of vacuum fluctuations of the electromagnetic field. As the two plates close on each other, longer electromagnetic waves no longer fit between them. The result: The total energy between the plates is less than the amount pushing them together from the vacuum that surrounds them. The Casimir effect increases as distance decreases, and if you get to atomic-scale distances, it has been measured at tons per square meter.
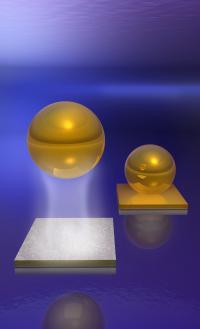
From a propulsion perspective, the idea that empty space contains energy in the form of these fluctuations of electric and magnetic fields is quite interesting, but we are in the earliest stages of understanding how the effect works. Interesting news, however, has just come out of Harvard University, where researchers have experimented with replacing the vacuum with a fluid. They were then able to measure a repulsive form of the Casimir effect. Working with a gold-coated microsphere attached to a mechanical cantilever in a liquid, the team measured its deflection as they varied the distance from a nearby silica plate.
Image: This is an artist’s rendition of how the repulsive Casimir-Lifshitz force between suitable materials in a fluid can be used to quantum mechanically levitate a small object of density greater than the liquid. Figures are not drawn to scale. In the foreground a gold sphere, immersed in Bromobenzene, levitates above a silica plate. Background: when the plate is replaced by one of gold levitation is impossible because the Casimir-Lifshitz force is always attractive between identical materials. Image courtesy of the Capasso lab. Credit: Harvard University.
A repulsive Casimir effect gets us into some practical uses for these strange phenomena, as Federico Capasso (Harvard School of Engineering and Applied Science) points out:
“Repulsive Casimir forces are of great interest since they can be used in new ultra-sensitive force and torque sensors to levitate an object immersed in a fluid at nanometric distances above a surface. Further, these objects are free to rotate or translate relative to each other with minimal static friction because their surfaces never come into direct contact.”
By contrast, attractive Casimir forces could obviously hamper extreme miniaturization. At the nanoscale, then, developing bearings that use this ‘quantum levitation’ is helpful in reducing friction among tiny components. Nanotechnology itself is a major interstellar driver — if we can reduce payload sizes down to nanotechnological scales, the propulsion problem becomes much more tractable. We can envision sending, for example, tiny probes that can use assembler technology upon arrival to build a robotic research station in a new planetary system, needing to accelerate far less mass than that of a conventional probe.
To the extent, then, that this work takes us deeper into a workable nanotechnology, it becomes useful for interstellar purposes. But it is also notable in that it suggests possible (though highly problematic) long-range uses of Casimir forces in propulsion, as Brian Wang notes in NextBigFuture. Brian flags Jordan Maclay’s “Study of Vacuum Energy Physics for Breakthrough Propulsion,” available here, which grew out of work for the Breakthrough Propulsion Physics project, and which takes a detailed look at ways this energy might be exploited.
The paper is Munday et al., “Measured long-range repulsive Casimir-Lifshitz forces,” Nature 457 (8 January 2009), pp. 170-173 (abstract). A Harvard news release is available. The reference for the Robert Forward paper is “Extracting Electrical Energy from the Vacuum by Cohesion of Charged Foliated Conductors,” Physical Review B 30, no. 4 (August 1984), pp. 1770-73.
by Paul Gilster | Jan 9, 2009 | Deep Sky Astronomy & Telescopes |
Finding something unexpected adds immeasurably to the pleasure of doing science. Yesterday we looked at an anomalous transient in Boötes, one that has already spawned a number of theories to explain it. Today let’s look at some of the radio noise that pervades the cosmos, and an intriguing experiment that discovered more of it than expected. The story makes this writer marvel again at how the universe continues to change the game. I like how Philip M. Lubin (UCSB) puts it:
“It seems as though we live in a darkened room and every time we turn the lights on and explore, we find something new. The universe continues to amaze us and provide us with new mysteries. It is like a large puzzle that we are slowly given pieces to so that we can eventually see through the fog of our confusion.”
Indeed. Lubin is on the team behind the NASA balloon-borne experiment called ARCADE (Absolute Radiometer for Cosmology, Astrophysics, and Diffuse Emission), which discovered this particular static back in 2006. Like all but one of the stories we’ve considered this week, this one grows out of material presented at the AAS meeting in Long Beach, a conclave that has been unusually productive this time around. Productive, that is, if publicizing unusual results inspires scientists to renewed efforts to explain them and focuses further research on the question.
You could combine the radio emissions from all the radio galaxies in the universe and it would not be enough to equal the noise found by ARCADE. Known radio sources of all kinds simply don’t explain it, nor does an origin in primordial stars. Indeed, the potential signature of the very first stars is more or less masked by the unexpectedly loud noise, complicating our plans to study it. What astronomers now hope is that the cosmic static may offer clues to the development of galaxies in a later epoch, information that would help us understand early radio sources.
All told, four papers have been offered to the Astrophysical Journal on these findings in addition to the AAS presentation, and according to this New York Times story, the discovery team has not ruled out the idea that the signal comes from black holes produced by the earliest stars. But that’s a longshot, as Alan Kogut (NASA GSFC) is quick to note: “I emphasize that this interpretation is just speculation at present — no one has yet done any real calculations to see if this holds up under closer scrutiny or not.”
That’s a valuable reminder of scientific method at work, as results are presented through meetings and journals and widely discussed and critiqued. Findings this odd may take quite a while to sort out, but they’re a spur toward putting more brain power onto the question. We get such surprises because of new instrumentation — in this case, the wavelength in question has simply not been well studied until ARCADE, falling as it does between the range examined by Earth-based radio telescopes and the shorter wavelengths that are the domain of satellites like COBE, the Cosmic Background Explorer.
We have a long way to go here, but it’s remarkable that we’re pushing back the frontiers of study past so-called Population II stars, which are older than our Sun and poorly endowed with metals, into the hypothetical Population III, the earliest stars, formed from pure hydrogen and helium. Studying the latter has been rendered considerably more tricky by these results, but a new phenomenon may be emerging whose uses have yet to be determined. As I’ve opined before, what a time to be studying cosmology!
Among the papers submitted to the Astrophysical Journal, you might want to begin with Kogut et al., “ARCADE 2 Observations of Galactic Radio Emission” (available online). You can run a search on the arXiv site for the accompanying work, including a description of the ARCADE instrument. NASA GSFC offers a news release.
by Paul Gilster | Jan 8, 2009 | Deep Sky Astronomy & Telescopes |
We continue to follow the American Astronomical Society’s meeting in Long Beach with fascination. This has, indeed, become AAS week in these pages. But amidst the news of brown dwarf discoveries, a more massive Milky Way than previously thought, and asteroids around white dwarf stars, the story of a genuine mystery stands out. Such a mystery is the optical transient known as SCP 06F6, a flash of light picked up by the Hubble Space Telescope back in 2006. Have a look at the images below:
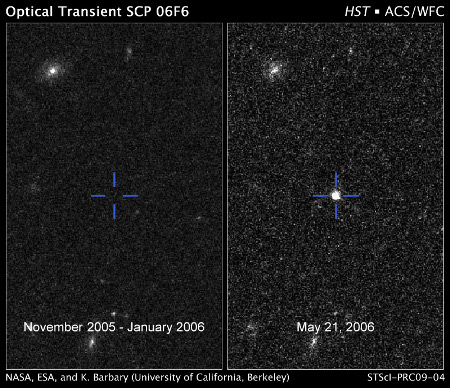
Image: This pair of NASA Hubble Space Telescope pictures shows the appearance of a mysterious burst of light that was detected on February 21, 2006. The event was detected serendipitously in a Hubble search for supernovae in a distant cluster of galaxies. The light-signature of this event does not match the behavior of a supernova or any previously observed astronomical transient phenomenon in the universe. Credit: : NASA, ESA, and K. Barbary (University of California, Berkeley/Lawrence Berkeley National Lab, Supernova Cosmology Project).
Kyle Barbary (Lawrence Berkeley National Laboratory) described follow-up work on this event to the gathered astronomers in Long Beach. Flashes of light from the cosmos are, of course, not rare in themselves — think supernovae, for example — but exploding stars peak after seventy days, whereas what Hubble saw steadily rose in brightness for a remarkable 100 days and then dimmed, more or less at the same rate, until it was gone. Nor does the transient suggest a gravitational lensing event, which would be much shorter. So what was it, and where did it occur?
The questions aren’t easy to answer. Hubble was looking at a galactic cluster eight billion light years away in the constellation Boötes when the event was detected, but the object could be anywhere along that line, which means it could even be in our own galaxy. Search through astronomical catalogs and you won’t find any sign of a star or galaxy where the flash occurred. Moreover, the light from the object fails to tag a specific element, although there is some suggestion we may be looking at redshifted molecular carbon absorption lines. If so, that would imply the source is a star approximately one billion light years away.
This is a signature, in other words, like no other celestial event ever recorded. The range of possible explanations thus far advanced is wide, an indication of just how odd this event really is. One theory is that we detected a collision between a white dwarf and a black hole, but that ranks as nothing more than a hypothesis at this point. Says Barbary, “We have never seen anything like it.”
More in this Space Telescope Science Institute news release.
by Paul Gilster | Jan 7, 2009 | Deep Sky Astronomy & Telescopes |
On my walk this morning, I was musing about the ongoing AAS meeting in Long Beach when I found myself having one of those epiphanies that seem to open a window into the heart of things. The day was unusually warm but gusts of wind tossed the trees and low clouds laced with rain scudded past. And suddenly I was no longer walking along a quiet street but became aware that I walking a planet within a star system, within a cloud of stars, and that by being made up of elements from those stars, I was in some sense an expression of that universe as it observed itself.
It’s hardly an original notion, but the sense of it was palpable, an almost physical awareness that translated something known factually into something experienced. It was spurred by the recent news that the Milky Way is fifty percent more massive than we thought, maybe the twin of the Andromeda Galaxy. Increasing our sense of scale adds to the grandeur. The punch of the Fermi Paradox comes from the sheer size of galaxies — we wonder about other civilizations because we understand that there are hundreds of billions of stars around us in this celestial city, surely many of them not all that different from our own.
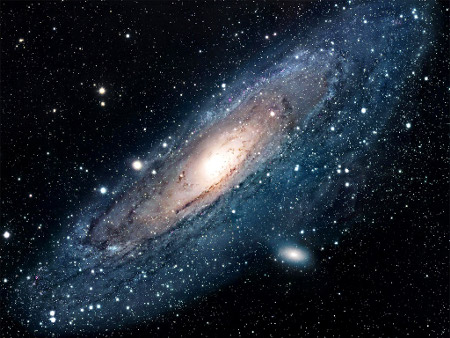
I felt awash in immensity as I walked the windy streets. For if it’s breathtaking to think that the Milky Way may hold hundred of billions of stars — 400 billion is a figure often cited — how much more so to consider it as massive as three trillion suns?
Image: The Andromeda Galaxy, perhaps a twin of our own. We can see Andromeda from without, but determining the structure of the galaxy we move through ourselves is a continuing challenge. Credit: NASA.
A mass of three trillion suns is what the work of Mark Reid (Harvard-Smithsonian Center for Astrophysics) and colleagues suggests. The team of astronomers used the Very Long Baseline Array to look at star-forming regions across the galaxy, zeroing in on natural radio emissions that are amplified by gas molecules in certain areas. These ‘cosmic masers’ are markers that can be studied by the VLBA through parallax, measuring the apparent shift of the objects against the cosmic background. The work is particularly telling because it relies on few assumptions:
“The new VLBA observations of the Milky Way are producing highly-accurate direct measurements of distances and motions,” said Karl Menten of the Max Planck Institute for Radio Astronomy in Germany, a member of the team. “These measurements use the traditional surveyor’s method of triangulation and do not depend on any assumptions based on other properties, such as brightness, unlike earlier studies.”
Using these direct measurements, the astronomers could determine that most of the star-forming regions move around the galactic center not in a circular but an elliptical orbit. They were also able to determine that the Milky Way probably has four spiral arms of gas and dust that are continually producing stars, with young stars dominating two of these. But most striking was the team’s finding that our Solar System is rotating much faster around the galactic core than previously believed, in the neighborhood of 965,000 kilometers per hour.
The higher speed of our movement around the center demonstrates the need to up our estimates of the galaxy’s mass by the fifty percent mentioned earlier. And it brings home a crucial fact: Although we live within it, we still must contend with our limited knowledge of the Milky Way’s size and structure. And if we are going to start talking about a serious upgrade to the mass of the Milky Way, as the evidence that Reid reported at the AAS session on Monday suggests, we have to remember that we still cannot see ourselves whole, the way we can examine, say, Andromeda.
Walking in the early morning winds, I reflected on all this, and on the fact that seeing something complete from within is notoriously tricky, whether that something be as vast as a swarm of stars or as miniature as a human relationship. Identifying the markers, like Reid’s cosmic masers, is crucial, but even then, the sample of markers has to be extended to broaden the picture. As we work out the science, the sense of awe inspired by our newly massive galaxy creates a freshening humility that enriches everyday experience.
More on Reid’s work in this National Radio Astronomy Observatory news release. Both the New York Times and Science News also pick up on the story.
by Paul Gilster | Jan 6, 2009 | Exoplanetary Science |
Our second transiting Neptune-mass planet has been discovered via the HAT Network of small, automated telescopes maintained by the Harvard-Smithsonian Center for Astrophysics. HAT-P-11b is described by Greg Laughlin at systemic (thanks to many who sent this link):
HAT-P-11b is quite similar in mass and radius to Gliese 436b, and it’s actually somewhat larger than Neptune on both counts. When the mass and radius are compared to theoretical models, it’s clear that, like Gliese 436, it’s mostly made of heavy elements (that is, some combination of metal, rock and “ice”) with an envelope of roughly 3 Earth masses of hydrogen and helium). It’s completely dwarfed when placed next to an inflated hot Jupiter, HAT-P-9b, for instance…
The advantages of a detected transit are great. Couple the transit light curve with radial velocity measurements and you can work out the mass and radius of the transiting planet. Moreover, the opportunity to investigate planetary atmospheres comes into play through follow-up observations that can also tell us something about planetary temperatures. And because sixteen out of the twenty ‘hot Neptune’ planets we’ve found through various methods have been part of multiple planet systems, it’s no surprise that there is at least some evidence of a second planet to accompany HAT-P-11b.
It’s helpful indeed that HAT-P-11 will be in the Kepler mission’s observational field. With Kepler designed for a minimum mission of 3.5 years, the space-borne observatory should have this Neptune-class world available for more than 250 transits. That will firm up all our parameters for the planet based on the transit light curve, and will allow new evidence to be gathered for a second planet in the system. Knowing in advance that a particular Kepler star has a transiting world around it allows detailed observations to begin from the mission’s outset.
More in Bakos et al., “HAT-P-11b: A Super-Neptune Planet Transiting a Bright K Star in the Kepler Field,” submitted to the Astrophysical Journal and available online. Related: Stringellow et al., “Transit Timing Observations of the Extrasolar Hot-Neptune Planet GL 436b,” available here.