by Paul Gilster | Dec 9, 2009 | Outer Solar System |
Although we have no direct observations of objects in the Oort Cloud, we may be able to change that with space missions like Kepler and CoRoT. So argue Eran Ofek (Caltech) and Ehud Nakar (Tel-Aviv University) in a recent paper. If they’re right, we’ll improve our understanding of the Solar System’s planetary accretion disk and get a better feel for the dynamics of planet formation. Right now even the largest telescopes can’t find Oort Cloud objects. Where do Kepler and CoRoT fit in?
The answer is that they may be able to observe occultations of background stars, a method that has been put to use for Kuiper Belt Objects already, although to date there is only one reported occultation by a KBO. Ofek and Nakar look at the rate of occultation events, creating an estimate that shows the possibility of Kepler detections of Oort objects and presenting statistical methods that can be used to verify that any occultations are real events and not simply noise in the data. Moreover, they think we should be able to differentiate between Oort objects and KBOs using these methods.
The odds of detection favor Kepler more than CoRoT, but searching the data may surprise us. From the paper:
Considering Kepler capabilities and reasonable Oort Cloud parameters, we find that Kepler may detect 0 to ~ 102 stellar occultations by Oort Cloud objects. We find that Kepler is unlikely to detect Kuiper Belt objects (mainly because of its high ecliptic latitude pointing). Moreover, CoRoT is unlikely to detect Kuiper Belt or Oort Cloud objects. However, the exact properties of the Oort Cloud and the Kuiper Belt are not known. Therefore, such searches are warranted.
True enough, especially when you consider that the occultation rate depends on many things we don’t know, including the mass of the Oort Cloud, the distance to its ‘inner edge’ (now thought to be between 1000 and 3000 AU), and the size distribution of the objects within it. Whether or not we do get Oort object detections from Kepler, these results could be helpful for future space-based exoplanet detection missions, which could add useful observations of events in the outer reaches of our own system to their primary purpose.
The paper is Ofek and Nakar, “Detectability of Oort cloud objects using Kepler,” submitted to Astrophysical Journal Letters and available as a preprint.
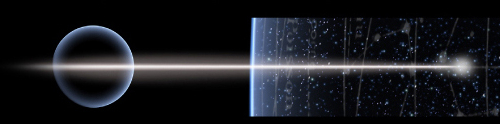
by Paul Gilster | Dec 8, 2009 | Deep Sky Astronomy & Telescopes |
That ignorance can cause cascading errors is a lesson never better taught than through the experience of George Brown, British foreign secretary in Harold Wilson’s government (this was about forty years ago). One day Brown was in Peru for a reception and, indulging in the local spirits, became thoroughly ripped. Enjoying the music, he was quick to act when a guest in flowing purple robes went by. An entranced Brown followed and asked the mysterious person to dance.
The New York Times recalls the result: The purple-robed figure refused the overture. “First, you are drunk,” the guest is said to have replied. “Second, this is not a waltz; it is the Peruvian national anthem. And third, I am not a woman; I am the Cardinal Archbishop of Lima.”
Cascading errors are always fun except when they happen to you. This morning I’ve had several computer glitches of increasing intensity. Finally, when I thought I had them all worked out, a window popped up advising me that my C: drive was about to fail. Hmmm… I use multiple drives, but I boot Linux off C:, so I’m hoping I can get today’s entry finished before anything further happens. One more problem and Centauri Dreams is through for the day.
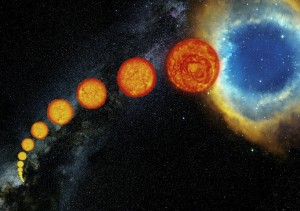
But before that happens, let’s see if I can squeeze in a new study of Sun-like stars made with ESO’s Very Large Telescope, work which has pointed to our own ignorance of a continuing mystery. Here’s the problem: Stars with the mass of the Sun normally swell into cool red giant stars that show regular variations in luminosity at timescales of several years. The process is thought to be well understood: The star is swelling and shrinking, becoming brighter and dimmer on a regular basis. But about a third of Sun-like stars at this point in their lives demonstrate an additional variation, one astronomers are calling a Long Secondary Period (LSP). The problem is to figure out what causes the LSP.
Image: Born from clouds of gas and dust, stars like our Sun spend most of their lifetime slowly burning their primary nuclear fuel, hydrogen, into the heavier element helium. After leading this bright and shiny life for several billion years, their fuel is almost exhausted and they start swelling, pushing the outer layers away from what has turned into a small and very hot core. These “middle-aged” stars become enormous, hence cool and red — red giants. All red giants exhibit a slow oscillation in brightness due their rhythmic “breathing” in and out, and one third of them are also affected by additional, slower and mysterious changes in their luminosity. After this rapid and tumultuous phase of their later life, these stars do not end in dramatic explosions, but die peacefully as planetary nebulae, blowing out everything but a tiny remnant, known as white dwarf. Credit: ESO.
Peter Wood, the team leader of a recent program monitoring 58 Sun-like stars in the Large Magellanic Cloud, used the FLAMES/GIRAFFE spectrograph on the Very Large Telescope combined with images from other telescopes to study the properties of these stars over two and a half years. One explanation for LSPs had been pulsations, but that theory seems to have been disproven. Says Wood:
“The newly gathered data show that pulsations are an extremely unlikely explanation for the additional variation. Another possible mechanism for producing luminosity variations in a star is to have the star itself move in a binary system. However, our observations are strongly incompatible with this hypothesis too.”
In a paper on this work, Wood (Australian National University) and colleague Christine Nicholls explain their interest in studying these stars to see whether unusual amounts of circumstellar dust are involved. A snippet from the paper (internal references omitted for brevity):
The change in visible light associated with the LSP is typically of a rather irregular nature, and the light variation can be large up to a factor of two. In the binary model, it has been suggested that the light change could be due to a large dust cloud orbiting with the companion and obscuring the red giant once per orbit… Another possible explanation for the LSPs… involves semi-periodic dust ejection events such as those predicted by theoretical models of AGB stars.
And indeed, the stars under investigation show a mid-infrared flux excess, which would be the result of stellar light being absorbed by circumstellar dust and re-radiated at these wavelengths. That indicates that the LSP fluctuations cause the red giant to lose mass, leading to increased dust formation, but the paper points out that we still don’t know what causes the LSP phenomenon in the first place. Frustrating? You bet. But the recent search does offer up a lengthy set of properties for stars with LSPs, and more or less throws down the gauntlet to researchers to solve the mystery. Sometimes knowing what we don’t know is the only way to revitalize the search for genuine knowledge.
Another error message. I’ve got to get this posted fast.
The papers are Nicholls et al., “Long Secondary Periods in Variable Red Giants,” Monthly Notices of the Royal Astronomical Society Vol. 399 Issue 4 (November, 2009), pp. 2063-2078 (preprint available). See also Wood and Nicholls, “Evidence for mass ejection associated with long secondary periods in red giants,” Astrophysical Journal Vol. 707 (10 December, 2009), pp. 573-579 (preprint).
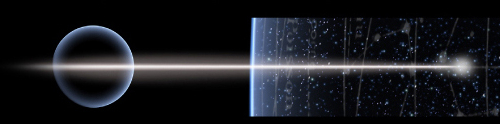
by Paul Gilster | Dec 7, 2009 | Sail Concepts |
What happens to a solar sail as it flies through space? Made of the most diaphanous materials possible, the sail gradually begins to degrade. Roman Kezerashvili and Gregory Matloff (CUNY) have looked closely at problems like these and have considered everything from sail thickness and performance to the merits of different metallic films. The sail material of choice seems to be beryllium, three times lighter than aluminum, and with a usefully high melting point. One interesting configuration is a twin-walled, hydrogen-inflated sail with walls ten to twenty nanometers thick. But build such a craft carefully. If solar radiation causes the constituent beryllium to become degraded, the structural integrity of the sail is at risk and hydrogen begins to escape.
Solar sails need flight testing (and The Planetary Society’s plans for a solar sail seem to be developing nicely), but we’ll doubtless learn huge lessons from those early tests that will substantially revise our thinking. What happens, for example, when ultraviolet radiation ionizes the sail, creating a positive charge? The ionized sail then begins to deflect protons in the solar wind, increasing its speed. That sounds like a welcome effect, but too much electrostatic pressure carries the potential for rupturing a hollow-body sail. We need to experiment with these things in space as well as applying our best minds to the problem of deployment and performance.
Working Close to the Sun
It’s no surprise to the Centauri Dreams community that both Matloff and Kezerashvili have been actively at work on solar sail questions. We’ve examined many of Matloff’s papers and books in these pages, and Kezerashvili’s presentations at the summer confeence in Aosta, covering the effects of General Relativity on sail operations, were also discussed here. But how welcome to see them given publicity outside the space community. The colleagues made the cover of CUNY’s Salute to Scholars, a glossy publication for the university community featuring the two men, along with relativist and string theorist Justin F. Vázquez-Poritz and fiber-optics researcher Lufeng Leng.
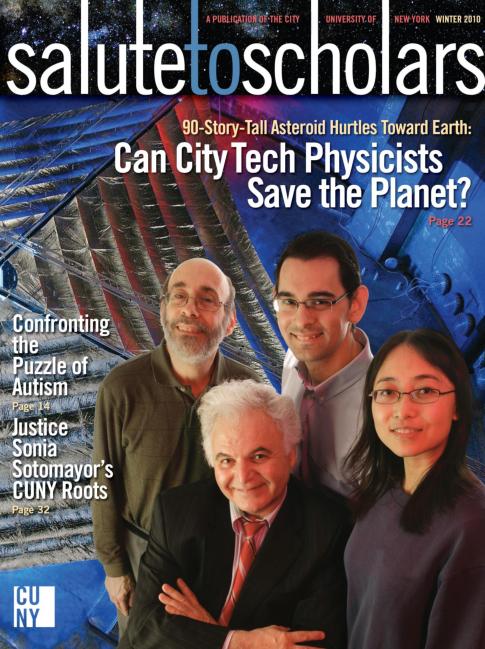
The article is available online. That’s Matloff at top left, Vázquez-Poritz at top right, Kezerashvili bottom left and Lufeng Leng bottom right.
Vázquez-Poritz joined Kezerashvili at Aosta in examining sails from a unique perspective. Newtonian physics works fine for most current space operations, but things change dramatically when you start talking about bringing a solar sail in close to the Sun, as would occur in ‘sundiver’ mission concepts that seek to maximize the effect of solar photons and drive up the acceleration of the sail. Kezerashvili and Matloff calculate that a sail deployed at 0.5 AU could reach the heliopause in a mere 2 1/2 years — compare that to the thirty years the trip took the Voyager probes.
CUNY’s Neill Rosenfeld quotes Kezerashvili:
“You ask me why do I want to come so close to the sun with a solar sail?” says Kezerashvili. “I want to know what happens beyond the solar system, and if I launch something, I should know the result during my lifetime.”
Absolutely right, if we can find a way to do it, and a close solar pass seems to be the ticket, provided we can make the needed advances in materials science to withstand the environment. The article goes on:
He and Vázquez-Poritz considered Kepler’s third law, a mathematical formula conceived in the early 1600s that describes the relationship between the longer orbital periods of planets far from the sun and the shorter orbital periods of planets close to the sun. In two papers, they argue that deviations from Kepler’s law occur when the curvature of spacetime and solar radiation pressure act simultaneously on a solar sail-propelled satellite. In short, if you open the sail close to the sun and don’t account for Einstein, you might miss your target by more than 1 million miles.
“This could be an ideal way to test the effects of general relativity,” Vázquez-Poritz says. “With a solar sail, we could measure effects that otherwise would be too small to measure.”
Yes, and another way besides GPS to get the effects of General Relativity into an actual technological application.
The Asteroid Deflection Question
As to Matloff, the CUNY article homes in on his work on deflecting potentially threatening asteroids like Apophis. Here’s one way to move an asteroid: Use a large, parabolic collector sail to reflect sunlight onto a smaller, ‘thruster’ sail that would concentrate a hot beam of light on the asteroid’s surface. Eventually you get vaporized rock and a controllable jet that can be used to nudge the asteroid onto a new path. Lufeng Leng’s work at CUNY’s photonics lab aims to build a mathematical model of how the process would work, beaming differently colored laser beams at meteorite samples.
A crucial question is how far the light penetrates below the surface, for a beam that penetrates too deeply will simply heat the asteroid, while a beam that penetrates just the right amount — perhaps a thousandth of a millimeter — would produce a steerable jet. It all depends on better understanding the penetration depth of electromagnetic radiation (like light) in NEO regolith.
The CUNY story goes on to discuss the matter with Robert B. Adams, who headed a NASA team that performed an asteroid deflection study in 2007:
“The solar collector is definitely on the table,” says Adams. So are ideas including a nuclear explosion away from the asteroid, a kinetic impactor that would ram into it and a gravity tractor, which would hover near the asteroid and use the gravity that naturally occurs between them to pull the asteroid slowly off its course.
“The solar sail hasn’t received as much attention, but it’s a good application with NEOs because it gives you more control over which way your thrust is generated,” Adams says.
Pushing Past the Heliosheath
Where does all this take us? A manned mission to a nearby asteroid seems a reachable goal, one that would deploy and test solar sail methodologies to provide data needed to achieve workable designs. That’s an insurance policy we need to have no matter which technology is ultimately deployed for any future deflection mission. Asteroids aside, the CUNY researchers are also showing us a realistic option for a sail mission into nearby interstellar space. Ponder a sail 1800 meters in diameter carrying a 150-kilogram scientific payload, capable of reaching the Oort Cloud in thirty years.
Writer Neill Rosenfeld’s work on this story is excellent. He covers not only the researchers’ ongoing studies but looks at the development of the sail concept in history and runs through asteroid deflection models ranging from sails to kinetic energy impactors and nuclear interceptors. We’re at that point in funding where we’re technologically ready to move sail work into space for shakedown and development, but funding constraints almost guarantee that sails like The Planetary Society’s LightSail and other private or commercial ventures will carry the concept forward.
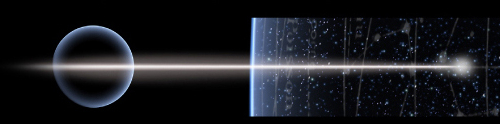
by Paul Gilster | Dec 4, 2009 | Exoplanetary Science |
We don’t exactly know what to call GJ 758 B, which may be a brown dwarf or simply a large planet of between ten and forty Jupiter masses. But the detection is being hailed as the first direct observation of a ‘planet-like object’ orbiting a star similar to our own Sun. We have the new High Contrast Coronagraphic Imager with Adaptive Optics (HiCIAO), recently attached to the Subaru Telescope and working in the near infrared, to thank for the detection.
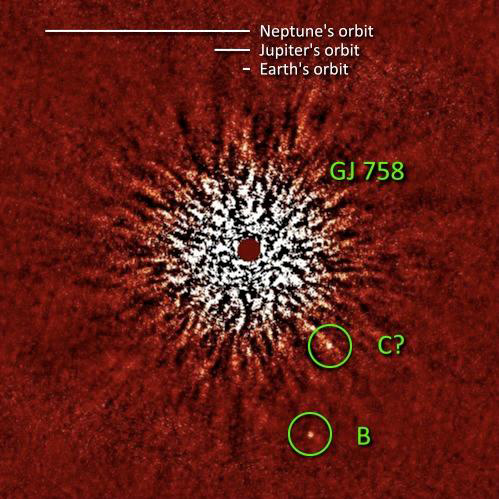
Image: The August 2009 discovery image of GJ758 B and C, taken with Subaru HiCIAO in the near infrared wavelength. Without angular differential imaging, the star’s speckle halo (burst-like feature in the center) would overwhelm the signals from the planet candidates. Credit: Subaru Telescope/NAOJ.
Masking the star’s intense light and using a technique known as angular differential imaging, HiCIAO seems to promise great things in the way of future direct detections of exoplanets, where the object is directly seen rather than having its existence inferred through study of the parent star. Says Marc Kuchner (NASA GSFC): “It’s amazing how quickly this instrument has come online and burst into the forefront. I think this is just the beginning of what HiCIAO is going to do for the field.”
GJ 758 is a G9-class star of about 0.97 solar masses some fifty light years from the Sun. As to the newly discovered companion, it’s currently about 29 AU out, about the orbital distance of Neptune, but we do not yet have further information on the size and shape of its orbit. At 315 degrees Celsius, the object is the coldest companion yet imaged around a G-class star. A second companion of similar mass is suspected at about 19 AU, roughly the orbital distance of Uranus from the Sun. The presence of massive planets at this distance from their primary challenges our current thinking on planetary formation and may help theorists to refine their models.
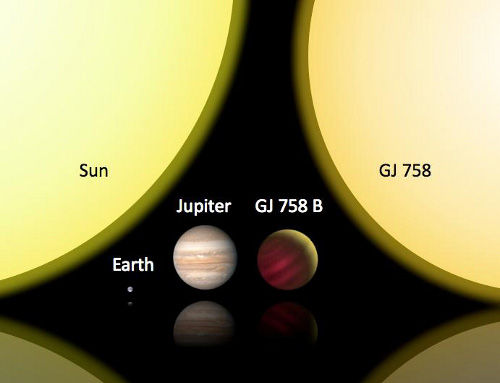
Image: Size comparison between representatives of our Solar System (Sun, Jupiter, and Earth) and the GJ 758 system. GJ 758 B’s temperature of about 315 C makes it glow cherry-red.
“This challenging but beautiful detection of a very low mass companion to a Sun-like star reminds us again how little we truly know about the census of gas giant planets and brown dwarfs around nearby stars,” said Alan Boss, an astronomer at the Carnegie Institution for Science in Washington, D.C., who was not involved in the research. “Observations like this will enable theorists to begin to make sense of how this hitherto unseen population of bodies was able to form and evolve.”
The paper is Thalmann, “Discovery of the Coldest Imaged Companion of a Sun-Like Star,” Astrophysical Journal Letters, published online 18 November 2009 (abstract). More in this Princeton University news release.
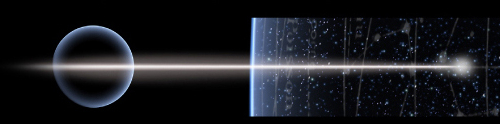
by Paul Gilster | Dec 3, 2009 | Exoplanetary Science |
You won’t want to miss an interview with Debra Fischer now available on the MarketSaw site. The latter is a blog focused on 3D motion pictures, and thus the interest in Fischer’s work on Alpha Centauri draws from a cinematic base. Specifically, James Cameron’s new movie Avatar depicts a gas giant with a habitable moon around it, and the MarketSaw editors are interested in whether such a planet could exist around one of the Centauri stars. The interview that follows, discussing Fischer’s ongoing hunt for Centauri planets, is prime reading. I’ll quote from it, but you’ll want to read the whole thing (thanks to Vincenzo Liguori for the tip).
As to the gas giant question, we can answer that one quickly. Neither Centauri A nor Centauri B is orbited by a gas giant. We know this because enough data have accumulated on the question to rule such planets out. Stable orbits, says Fischer, don’t reach out much further than 2 AU around either star, and the lack of gas giants leaves smaller worlds a serious possibility, with the always tantalizing thought that there might be a terrestrial planet in the habitable zone. Fischer clarifies the orbital distances possible this way:
The stars themselves are in a very elongated, eccentric, elliptical orbit. At the widest part of their orbit, they are almost 40 AU (40 times the earth to sun distance) apart. That’s a comfortable separation for orbiting planets. But, when the stars, A and B, make their closest approach, they are only 11 AU away from each other. The stars are moving into the domain of the planets, and they will gravitationally rip away any planets that aren’t closer than 2 AU around “A” or “B”. Several computer simulations have demonstrated this. If you start the simulation with a system that has more distant planets, as the other star comes in closer, the planets are gravitationally disrupted, dislodged from their stable orbits. The orbits first become eccentric, and then the planet shoots right out of the system.
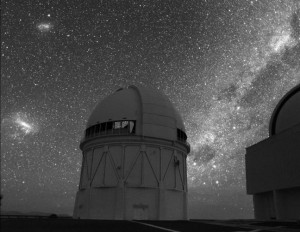
So we’re talking about planets, if they exist, that are inside the orbit of the main asteroid belt in our own Solar System (centered about 2.7 AU from the Sun). Fischer goes on to describe the work using a 1.5-meter telescope in Chile, as well as the Keck and Lick Observatory instruments. Why has a dedicated Alpha Centauri search taken so long? Fischer notes that the game has changed. Early on we were looking at lots of stars to build up a catalog of planets. Now we’re ramping up the precision to try to find Earth analogues. The ‘high cadence’ observations Fischer is making began in earnest on January 1 of 2009 and study Centauri A and B intensively:
We use one telescope, but we nod back and forth. The exposure times are 15 seconds for A, and 30 seconds for B. So we take ten observations of A, and then we move over to B and take ten observations there, then nod back to A and so on. So it’s just one telescope going back and forth. This is helpful because if we see the velocities of both stars just jump up or down for some reason, this will be a clue that there’s some kind of systematic error, and I hope that we’ll be able to track it down.
Image: The skies over the Cerro Tololo Inter-American Observatory, where Fischer’s work continues. Credit: San Francisco State University.
As to why Centauri B seems the more likely candidate for a planetary detection, Fischer has this to say:
As stars go, alpha Cen A and B are inactive. But, we don’t know if there are velocity fields at the sub-meter-per-second level. The stars are not completely smooth cue balls in space, like pool balls on your table. They have atmospheres, and those atmospheres have flares. In addition, the stars themselves pulsate. We know that Alpha Centauri “A” has a dominant pulsation period that’s about 5 minutes, just like the sun. I’m not really worried about periodic variations of minutes. We’ll be able to average right over that kind of noise. But no one knows whether or not there are long period variations in “A” or “B”. We do know that “A” is probably not as stable as “B”. People have measured pulsation periods in “A”, and in general we find that more massive stars have more active atmospheres. So yeah, I agree that “B” is a better bet. And if you told me that I could only observe one star, I’d choose B. But we’re studying both stars. And it turns out that this strategy of looking at both stars is pretty critical in ensuring a solid set of data.
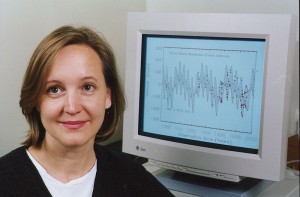
Any signal that turns up will only be what Fischer calls a ‘tiny whisper,’ but a confirmed planetary signature might well boost interest in future space missions like SIM Lite or a direct imaging Terrestrial Planet Finder mission. Interestingly, the Centauri stars seem well situated for the current tricky work from the ground, with an orbital plane of 79 degrees — any planets are assumed to inherit the same orbital tilt. The reflex velocity of the stars is thus more or less along the line of sight from Earth. Were the system perpendicular to us, the radial velocity technique would be ineffective.
Image: Planet hunter Debra Fischer. Credit: Wikipedia/Creative Commons.
Fischer’s instruments, and those of Michel Mayor’s team at La Silla, are sensitive enough to detect a Centauri planet down to Mars size, depending on the stability of the stellar atmospheres. We may well find one or more small, rocky worlds, at which point the question becomes whether or not such a planet could have oceans — we don’t know what close binaries may do to water delivery from asteroids and comets. Whatever the case, though, a Centauri planet in the habitable zone would be a potent stimulus to the imagination and an obvious target for interstellar probes of the future.
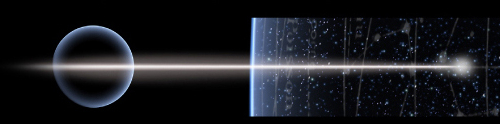