by Paul Gilster | Dec 10, 2010 | Exotic Physics |
New work at the University of Michigan, now written up in Physical Review Letters, discusses the possibility of producing matter and antimatter from the vacuum. The idea is that a high-energy electron beam combined with an intense laser pulse can pull matter and antimatter components out of the vacuum, creating a cascade of additional particles and anti-particles. UM Engineering research scientist Igor Sokolov has this to say about the theoretical study:
“We can now calculate how, from a single electron, several hundred particles can be produced. We believe this happens in nature near pulsars and neutron stars…”
That would make the vacuum a lively place indeed, as Sokolov acknowledges:
“It is better to say, following theoretical physicist Paul Dirac, that a vacuum, or nothing, is the combination of matter and antimatter—particles and antiparticles.Their density is tremendous, but we cannot perceive any of them because their observable effects entirely cancel each other out.”
Of course, to produce these hundreds of particles from a single electron, we need not only an ultra-high-intensity laser beam but a two-mile long particle accelerator. But the model is intriguing. We’re deep in the realm of quantum electrodynamics (QED), which describes how electrically charged particles interact by exchanging photons. Richard Feynman called QED ‘the jewel of physics’ because of its predictive capabilities. Feynman’s QED: The Strange Theory of Light and Matter (1986) is based on a lecture series designed for the general public, and without it, those of us who are mathematically challenged wouldn’t have a chance with QED ideas.
Reading the Sokolov paper, I can see that it’s time for me to return to Feynman, or maybe this Wikipedia entry, which calls QED ‘a perturbation theory of the electromagnetic quantum vacuum,’ and goes on to provide a useful backgrounder on the theory’s evolution. But let’s say this: Normally, matter and antimatter destroy each other when they come into contact. The UM team is interested in how this annihilation may be averted under certain conditions. Thus Sokolov colleague John Nees:
“…in a strong electromagnetic field, this annihilation, which is typically a sink mechanism, can be the source of new particles. In the course of the annihilation, gamma photons appear, which can produce additional electrons and positrons.”
One experiment in the 1990s produced effects that the Sokolov paper calls ‘weak and barely observable,’ creating gamma photons and electron/positron pairs, but the new work suggests that ramping up the laser pulse intensity should produce a more definitive result. The UM team’s equations model how a sufficiently strong laser could cause the creation of more particles than were initially injected into the experiment through a particle accelerator. Sokolov again:
“If an electron has a capability to become three particles within a very short time, this means it’s not an electron any longer. The theory of the electron is based on the fact that it will be an electron forever. But in our calculations, each of the charged particles becomes a combination of three particles plus some number of photons.”
The HERCULES laser at the University of Michigan is one way to test this work in a small-scale laboratory setting, but a similar laser would have to be built at a particle accelerator like the SLAC National Accelerator Laboratory at Stanford to work out all its implications, and because no such construction is planned, it may be some time before we can take these ideas forward. The team speculates that their theory may have applications for inertial confinement fusion — I bring this up particularly because of the interest of the Project Icarus team in ICF — but it’s a case of intriguing theory awaiting the experimental infrastructure to test it adequately.
The paper is Sokolov et al., “Pair Creation in QED-Strong Pulsed Laser Fields Interacting with Electron Beams,” Physical Review Letters Vol. 105, 195005 (2010) Abstract/Preprint. The paper is so dense in mathematics that I want to be sure to give you this news release as well.
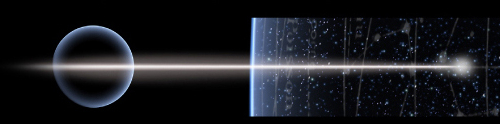
by Paul Gilster | Dec 9, 2010 | Exoplanetary Science |
Yesterday’s successful launch of a SpaceX Falcon, and the subsequent safe return of the Dragon spacecraft after a three hour ride, puts an exclamation point on Dana Andrews’ paper on space and commercial viability, which was discussed here yesterday. We’re a long way from a sustainable space infrastructure — many reports note the fact that what SpaceX did yesterday roughly parallels where humans were in space about fifty years ago, with the early Soviet and American flights — but we are seeing the most promising signs yet of a viable launch business emerging from the commercial sector, with all that implies about eventual use of space resources and future colonization.
Exoplanetary Puzzles
Now we wait for news from NanoSail-D, whose sail deployment should, if my sources are right, be today, but the @NanoSailD Twitter feed has grown quiet. We’ll think good thoughts and, while waiting, move on to the exoplanet hunt, the latest news from which is the discovery that the planet WASP-12b, found last year by the Wide-Angle Search for Planets project, has an atmosphere dominated by molecules containing carbon. That’s a sharply different scenario from our Earth, which contains much smaller amounts of carbon and a great deal more oxygen.
The find makes for interesting speculation about other planets that might exist in the same system. Although WASP-12b is a ‘hot Jupiter,’ orbiting its star in a mere 26 hours, it’s possible that smaller, rocky worlds could form around the star, which is about 1200 light years away. Just what such worlds might be like is a question that intrigues Joe Harrington (University of Central Florida), who analyzed the Spitzer data for the study:
“A rocky planet in such a planetary system could have an interior abundant in diamonds and a surface littered with graphite and diamonds. The theorists will have fun with this one. Could life thrive in such an environment, with little oxygen or water? That might not be so far-fetched given last week’s announcement by NASA of bacteria that can survive by using arsenic in place of phosphorus, previously thought to be essential.”
A brief pause to note that the NASA work on arsenic is taking flak from many quarters (see the comments on Centauri Dreams‘ recent story on the announcement), leaving significant doubts about its credibility. The broader principle — that life may surprise us, though perhaps not in this case — is obviously still in play.
It took an analysis of WASP-12b’s infrared spectrum through Spitzer Space Telescope data to flag the significance of carbon, revealing that the world is the first planet found where the oxygen/carbon ratio is reversed over what we see in our own Solar System. Our Sun’s carbon-to-oxygen ratio is about one to two (half as much carbon as oxygen), and at least among the inner planets, none have been found to have more carbon than oxygen (the ratio for the outer planets has not yet been established). WASP-12b is the first planet to have a carbon-to-oxygen ratio greater than one measured, a significant change from Earth normal.
“When the relative amount of carbon gets that high, it’s as though you flip a switch, and everything changes,” said Marc Kuchner, an astronomer at NASA Goddard Space Flight Center, Greenbelt, Md., who helped develop the theory of carbon-rich rocky planets but is not associated with the study. “If something like this had happened on Earth, your expensive engagement ring would be made of glass, which would be rare, and the mountains would all be made of diamonds.”
A Riddle Much Closer to Home
Not that WASP-12b wasn’t unusual to begin with. It’s close enough to its star that gravity has elongated it, pulling mass off the planetary atmosphere into a thin disk that orbits with the planet. Temperatures in the range of 2600 Kelvin (2325 degrees Celsius) make it one of the hottest exoplanets yet discovered. But the next step in the WASP-12b story may take place in a colder place. For to understand what’s happening there, we need to know whether planets like Jupiter have a carbon/oxygen ratio we can measure. It’s tricky to make that reading because much of Jupiter’s oxygen is trapped in water and thus not available for spectroscopic observation because it has condensed out of the atmosphere due to the cold at Jupiter’s orbital distance.
All eyes thus turn to Juno, a Jupiter mission that will launch in 2011 and reach the giant planet five years later, where it will map water and oxygen to determine whether Jupiter is as carbon-rich as WASP-12b. Getting the answer for Jupiter will tell us just how unusual WASP-12b really is, as this background story in Nature makes clear. The paper is Madhusudhan et al., “A high C/O ratio and weak thermal inversion in the atmosphere of exoplanet WASP-12b,” published online by Nature 8 December 2010 (abstract).
A Challenge to Formation Theories
Be aware as well of the interesting gas giant found around HR 8799. HR 8799e is one of four gas giants in this system, orbiting its star at 14.5 AU and in the process distinguishing itself from its more distant brethren (at 24, 38 and 68 AU respectively). Gravitational instability can be invoked to explain the formation of the three outer gas giants, but HR 8799e is in too warm a position at 14.5 AU to make it likely, leaving us with core accretion as the formation model.
But core accretion doesn’t fit the outer worlds, the process taking so long to complete that solid planetary cores would not have had sufficient gas from the dissipating protoplanetary disk to form a gas giant. Different formation models in the same system? It seems unlikely, because the masses of these planets are similar and they’re showing clear signs of orbital resonance, leading Christian Marois (Herzberg Institute of Astrophysics) to suggest the planets got where they are today through orbital migration. HR 8799, some 129 light years from Earth, is going to preoccupy planetary formation theorists for some time to come. The paper is Marois et al., “Images of a fourth planet orbiting HR 8799,” published online in Nature 8 December 2010 (abstract).
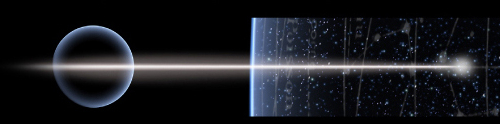
by Paul Gilster | Dec 8, 2010 | Culture and Society |
Various accounts of what happened to Japan’s Akatsuki Venus orbiter continue to come in, but it seems clear that the craft failed to achieve orbit. Sky & Telescope has been keeping a close eye on things and reports that errant thruster firings evidently caused an unexpected rotation that resulted in an on-board computer putting the vehicle into standby mode. The result: Too short a burn to ensure orbital capture, with Akatsuki now in a solar orbit that won’t take it back to Venus for another seven years. Are course changes possible for another go then? We’ll see.
Supply, Demand and Near-Earth Space
In an unaccustomed way, the Venus news has me in an inner system mode this morning, which means it’s probably a good time to talk about Dana Andrews’ thoughts on supply and demand when it comes to space colonization. Andrews (Andrews Space, Seattle) and colleagues Gordon Woodcock (Space America Inc.) and Brian Bloudek have been putting together a scenario for near-term commercialization of space, one that takes a hard-headed look at the economic drivers that humans need to make their presence beyond Earth sustainable.
A space-based infrastructure is the ultimate goal here, and if we ever build one, it will have to be because we’ve found a compelling reason to do so, one wrapped up in economics and, perhaps, species survival as we face dwindling resources. Andrews made the case at this year’s International Astronautical Congress in Prague that mining scarce resources on the moon and Near Earth Objects could be the key to commercial development that will become critical as we face future shortages. It will also offer us a chance to move past our dependence on oil:
Our hypothesis is that if we add lunar resources, we can afford to maintain the switch to plug-in hybrids throughout the world, thereby increasing productivity and reducing persistent pollution. Even though the flow of lunar resources is relatively small, these critical metals have a large impact on productivity, resulting in a potential soft landing for the world population.
What kind of resources are we talking about? Andrews enumerates quite a few, a list on which items like rhenium — used in fuel-efficient aircraft engines — stand out. The price of rhenium is now over $11,000 a kilogram, twelve times what it was just four years ago. Reserves of indium, which is used in solar cells and LCDs, are forecast to run out within ten years, and so is the hafnium we use in computer chips and nuclear control rods. Such shortages and accompanying price increases can be the driver for space commercialization. Andrews proposes moving the mining and smelting of key non-renewable resources to the Moon, providing access to high grade ores and transferring potentially polluting mining operations away from our planet.
What Space Offers
So let’s look at space resources. Two-thirds of known meteorites are iron/nickel in composition, containing mostly iron but about 5-30 percent nickel and a few tenths of one percent cobalt, along with high concentrations (at least by standards on Earth) of strategic metals, from the platinum group to gold, gallium, germanium, iridium and others. Says Andrews:
Interestingly enough the lower the Fe-Ni metal content in the meteorite, the more enriched the Fe-Ni metal is in these rare and precious metals and elements. These elements readily dissolve into the metal that exists, and the less metal that exists, the less diluted they are. Many asteroids are richer in most of these precious metals than the richest Earth ores which we mine. Further, these metals all occur in one ore when it comes to asteroids, not in separate ores.
We don’t know how abundant rare Earth elements that can be mined actually are on the Moon, but Andrews wonders whether there are parallels between craters on the Earth and the Moon. The impact crater at Sudbury, Ontario is rich in iron, nickel, cobalt, copper and platinum group metals. Are these metals debris from an ancient impactor, or did they well up from within our planet after the impact? A study of impact craters on the Moon may give us some answers, for all asteroid impacts on the Moon should have left their debris on the surface, since the Moon lacks plate tectonics. What we need to learn is whether they are found there in concentrations we can mine.
What We Need to Know
Lunar prospecting, then, is a first step in determining the existence of asteroidal metal containing nickel, cobalt and platinum-group metals on the surface. We have much to learn, including not just the quality and location of ores, but also the location of volatiles like water. We also need to learn what happens when asteroidal nickel/iron is made into metal products, and to what extent we will have to rely on engineered alloys to get the desired result. At present, of course, we cannot test the processes we might use on the lunar surface, requiring a preliminary manned base there to work through these contingencies.
Andrews works out a simple cost model exploring mining, processing and shipping operations, comparing these to existing costs. With platinum, for example, selling at close to $40,000 per kilogram, a price that is itself escalating, the case for lunar mining is clearer than that for more plentiful products like cobalt. Even so, working in the lunar environment poses huge challenges:
A very important lesson learned is that most resource production equipment must be made on the Moon. Shipping it is unaffordable. For example, we assumed 90% of the mining and hauling equipment was made on the Moon. Even though this equipment is very productive (our estimates were that a miner or hauler could process on the order of 100,000 times its own mass in a year) so much of it is needed that indications are about 90% must be made on the Moon. That probably means electronics, electric motors, gears and bearings made on Earth and everything else on the Moon.
Getting from Here to There
Andrews proposes a lunar sling for launching metal products to Earth, but goes into greater detail on what any space infrastructure requires going out of the gate: A simple and inexpensive way to get to Earth orbit, what he calls FRETOS — Fully Reusable Earth-to-Orbit Systems. A fleet of five launchers supporting a flight rate of 1000 launches per year using four tethers is at the heart of the proposal. On the space side, a Skyhook capture device located at 300 kilometers orbital altitude is part of a picture that also includes a Low Earth Orbit station at 1000 kilometers, a powered winch module at 1700 kilometers and a counter-balance at 2400 kilometers. The total mass of the space segment is estimated at 190 metric tons, including 2100 kilometers of tether lines, high-speed winches, power generation arrays, counter balances and station-keeping components, all to be launched separately and docked together for assembly.
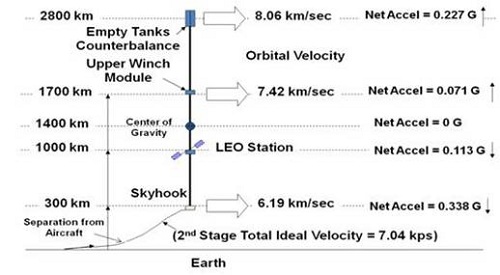
Image: Fully Reusable Earth to Orbit Stage (FRETOS) combined with Tether Upper Stage (TUS) provide cheap access to orbit. Credit: Dana Andrews/Roger Lenard.
As for getting to orbit, the launch segment is envisioned as a first stage subsonic carrier/aircraft with onboard hybrid rocket motor to achieve altitude for release of a second stage at 12.6 kilometers, with the second stage delivering a 13-ton payload to the capture point at the bottom of the 2100 kilometer long tether system. After payload capture, the second stage re-enters and glides to a landing at the launch and recovery base. Andrews’ assessment is that the entire recovery, turn around, launch and tether lift cycle could be completed within 24 hours, which works out to 1000 missions a year with the proposed fleet of 5 launchers and four tethers.
To get equipment to the Moon, outgoing payloads are released from the tether every day or so and transported to a lunar transfer station at L1, where they are assembled into lunar lander packages and then delivered to individual mining sites or research centers. The L1 station also collects and processes propellants for lunar landers and, possibly, deep space exploration missions, its operations almost entirely automated but allowing for the presence of a small crew as needed.
Economics of the Infrastructure
Will it pay for itself? The economic model Andrews built of the entire infrastructure as operational over a twenty year period shows the cost of rare metals brought back to Earth at about $2600 per kilogram, an investment he notes is ‘fairly lucrative’ given current costs, and while a flow of materials from the Moon will lower the price, demand should also increase assuming we find momentum to reduce our use of fossil fuels, leaving a sizable potential for profit.
There is more detail in the paper than I can squeeze into this post, but I’m struck by a couple of things. Andrews is working on the practicalities involved in commercializing space, and in doing so points to a model that should be economically sustainable, and one that should produce a permanent human presence not only on the Moon but other space venues. We don’t often look at near-Earth issues on Centauri Dreams, but of course the development of a Solar System-wide infrastructure is more or less a given if we are ever to produce a true interstellar probe, one that will tap the expertise and resources that infrastructure has made available.
I’m also struck by the potential of tether methods for moving large payloads around near-Earth space, and reminded of Michel van Pelt’s book Space Tethers and Space Elevators (Springer 2009) as a great introduction and backgrounder for those of you unfamiliar with the variety of tether concepts out there. You’ll find my earlier thoughts on this book here. As Andrews makes clear in his paper, getting out of the gravity well at minimal expense (and he believes his methods, if fully operational, could drop that cost to $250 per kilogram) is step one in getting the needed infrastructure built. A profitable space market must grow to make it happen.
The paper is Andrews et al., “Space Colonization: A Study of Supply and Demand.” I’ll pass along the full reference when it’s published.
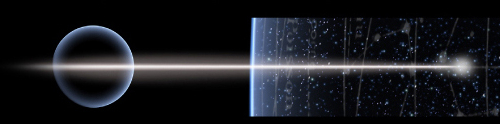
by Paul Gilster | Dec 7, 2010 | Sail Concepts |
While we wait for the NanoSail-D deployment, let’s talk about how to control a space-based solar sail. Japan’s IKAROS sail uses liquid crystal devices along the outer edge of the sail that actually allow the ground team to adjust the reflectivity of portions of the sail. Do that and you’ve created a situation where one part of the sail gets more of a boost from incoming photons than another, making it possible to maneuver the sail by light alone. It’s ingenious technology and, at least in early tests, seems to work.
Getting a sail to function seems easy. The tricky part is controlling what it does. We know that photons have no mass, but they do impart momentum, so that putting any large, reflective surface into space should result in forces acting upon it. James Clerk Maxwell demonstrated the basic principle as far back as 1873, although Johannes Kepler, observing the behavior of cometary tails, assumed they were affected by a ‘solar wind’ that could eventually be used to move a craft through space. The Echo-1 satellite was mightily manipulated by solar photons, a development startling to ground controllers. Space analyst James Oberg found a sports analogy to describe what happened, noting the need for stronger sail materials to handle the force:
…flexible materials that were light enough, mechanically strong enough, and physically resistant enough to the disintegrating effects of solar radiation just weren’t available until recent decades. As far back as 1960, photon pressure played orbital soccer with the Echo 1 thin-film balloon in orbit, pushing its orbit around with astonishing force until the balloon’s skin shattered. The shards were then flung far and wide by sunlight.
We’ll see what IKAROS has to teach us about controlling a craft through liquid crystal technologies and ponder their applicability to future missions. Meanwhile, Grover Swartzlander (Rochester Institute of Technology) has found a considerably different way to adjust the attitude of a solar sail. Swartzlander’s recent paper discusses a way to create lift — think of a cambered airfoil — that is responsive not to air but to light shining through the sail material. A future sail, then, might use not just photons striking its surface for momentum, but arrays of ‘lightfoils’ that can change the sail’s attitude.
The idea here is that an asymmeterical deflection of light moving through a transparent semi-cylindrical rod in the shape of a wing — that’s the ‘lightfoil’ — can create a lifting force. Swartzlander’s experiments involved small rods with a cambered shape that were immersed in water. A laser beam was then switched on to strike the rods, which moved away from the light source as predicted, but also showed a ‘lift’ effect, a movement perpendicular to the light. Using symmetrical spheres instead of the rods caused the perpendicular movement to disappear.
Science News discusses the result in a recent story:
Optical lift is different from the aerodynamic lift created by an airfoil. A plane flies because air flowing more slowly under its wing exerts more pressure than the faster-moving air flowing above. But in a lightfoil, the lift is created inside the object as the beam shines through. The shape of the transparent lightfoil causes light to be refracted differently depending on where it goes through, which causes a corresponding bending of the beam’s momentum that creates lift.
The shape of the lightfoil and the refractive index of the materials involved determine how much the photons change direction and ultimately control the direction of the lightfoil’s movement. The lift angle of the lightfoils turns out to be a hefty sixty degrees. Try taking an airplane off at that angle and you’ll be headed for a stall, but the paper on this work seems to show the lightfoil effect is stable. What lies ahead for Swartzlander is to test the effect with different materials, adjusting their shapes and refractive properties to find the optimal combination.
PUSHED ASIDE from Science News on Vimeo.
Are we talking about an effect that could be used for maneuvering a solar sail? In this New Scientist story, Swartzlander talks about using two perpendicular arrays of rods to control a future sail. Time will tell whether solar photons would be strong enough to generate sufficient force, and it may be that the ingenious IKAROS technology will have the upper hand. But we won’t know that until we find out how powerful an effect this is, which will call for a great deal more lab work and, if the effect proves out, eventual space testing.
The paper is Swartzlander et al., “Stable Optical Lift,” published online in Nature Photonics 5 December 2010 (abstract).
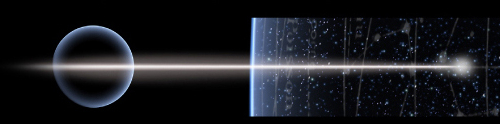
by Paul Gilster | Dec 6, 2010 | Sail Concepts |
NanoSail-D has always held a special place in my affections, probably because the solar sail effort has, until recently, been stalled. The IKAROS sail brought us into the sail age with gusto, but the sail team at NASA’s Marshall Space Flight Center has persisted through budget uncertainties and public indifference in finding a way to create a space-going sail seemingly out of thin air. No, it wouldn’t be the large, free-ranging sail we might have expected a few years back that could have so easily grown out of MSFC’s work, but it could be launched on the cheap. It could make it into space, and once there its short mission could break the trail for future sail efforts.
Remember that NASA developed two different 20 X 20-meter solar sails between 2001 and 2005, one fabricated by ATK Space Systems, the other by L’Garde Inc. Both these sails were tested in ground vacuum conditions, and MSFC’s Les Johnson pointed out in 2008 that they were solid designs, “… robust enough for deployment in a one atmosphere, one gravity environment and…scalable to much larger solar sails — perhaps as much as 150 meters on one side.” NanoSail-D, its sail made of a polymer called CP1 that is no thicker than single-ply tissue paper, finally gets NASA into actual space operations with an inexpensive demonstrator.
The First Test Passed
And so far, so good. While the first NanoSail-D perished in the launch attempt, the second has now been successfully ejected from the FASTSAT (Fast, Affordable, Science and Technology Satellite) that took it aloft, the latter equipped with six science and technology demonstration payloads when launched on November 19. The NanoSail-D ejection from FASTSAT is actually the first time NASA has ejected a small cubesat from an autonomous, free-flying microsatellite, and I’m delighted for the team and NanoSail-D principal investigator Dean Alhorn, who said this:
“This is a great step for our solar sail team with the successful ejection of the NanoSail-D satellite from FASTSAT. We had to carefully plan and calculate the ejection time, so we’d be lined up over the United States and our ground controllers to execute the next phase of the mission.”
Or in the more direct language of Twitter, in the form of two tweets from the NanoSail-D team (continuing coverage @NanoSailD):
Door is open. We have carrier!!!!!!!!!!!!!!!
followed by
That means we have ejected. Whew!
A bit of a white-knuckle moment indeed, with a bigger one to follow. That next phase will include a three-day countdown that will culminate in the crucial sail deployment, with the unfolding of NanoSail-D’s 32 square-meter polymer sail. The entire process takes about five seconds and, if successful, leaves the sail in low-Earth orbit for a period ranging from 70 to 120 days, depending on atmospheric conditions. Deploying a compact solar sail boom system is a major challenge of the enterprise, but so is measuring the effect of solar photons in an orbit low enough that atmospheric drag effects can mask the push from the Sun. The low orbit is part of NanoSail-D’s other mission, to test systems that could be used to de-orbit future Earth satellites.
Gritty Persistence and the Sail
We’ll follow NanoSail-D’s deployment fortunes with great interest. Meanwhile, ponder this: After the ground testing on two independent sail concepts, solar sail work at NASA seemed stalled. When NanoSail-D did emerge, commissioned in 2008, its team was given a mere four months for design and testing. Solar sailing was back, though with tight constraints of time and budget, followed by launch failure aboard a SpaceX Falcon 1. It’s the NanoSail-D spare we’re now testing in space, a case of making do with whatever parts were available. As Dean Alhorn says:
“It wasn’t a question of going off and doing an exhaustive study of what components to use. There was no time for that. We said, ‘Okay, this is the size of component we need, this is its function’ — and as soon as we found one that worked, we used it.”
NanoSail-D is what happens when people simply refuse to give up on a concept they believe in. Budgets get cut, people get assigned to new tasks, public interest drifts to other topics, but if you believe in what you’re doing, you continue to make the case. And if your mission isn’t as large or complex as you might have wanted, if it’s coupled with an auxiliary task (de-orbiting) that makes your measurements of solar sail effects that much harder to measure, then you find a way to make the best of it and get the sail deployed. That’s gritty, persistent engineering, and I’m hoping it pays off with a successful deployment and a green light for the next iteration of NASA sails.
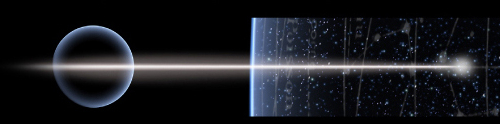