by Paul Gilster | Dec 16, 2014 | Exoplanetary Science |
A new paper out of George Mason University tackles the subject of planets deformed by tidal effects in close proximity to their star. It’s a useful study for reasons I’ll explain in a moment, but first a digression: I once had the chance to talk physics with the late Sheridan Simon, who besides being a popular lecturer on astrophysics at Guilford College (Greensboro, NC) also had a cottage industry designing planets for science fiction writers. Simon loved oddly shaped planets and because the Super Bowl was coming up, he had taken it upon himself to design a planet in the shape of a football, just to see what would happen if a place like this actually existed.
“And you know what? It works,” the bearded, exuberant Simon said with a grin. “But when you model what it looks like from space, the atmosphere is a problem. It looks plaid!”
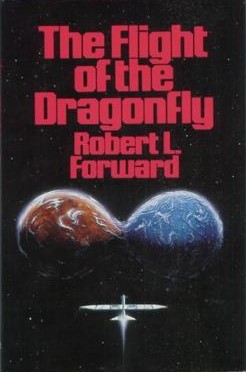
Simon played around with planets of every description, and if you’d like to see him at work on a planetary system around Tau Ceti, check what he developed back in 1992 for James K. Hoffman. I don’t know if he ever worked with Robert Forward, but of course the ultimate deformed planet in science fiction would be Rocheworld, from the novel of the same name (in an early version, Flight of the Dragonfly), where Forward envisions two worlds close enough to each other that they are deformed into egg-shapes and actually share an atmosphere.
Deformed planets in the realm of hot Jupiters have been studied for some time, for some of these worlds are close enough to their star to experience significant distortion in shape. One that Prabal Saxena and his George Mason University team mention in their paper is WASP-12b, which has been shown in earlier studies to have ellipsoidal variations in its transit depth that suggest a 3:2 ratio between the planet’s longest and its shortest axes. It’s an important effect because misunderstanding the distortion in shape caused by rotational and tidal effects can lead to mistakes in calculating the radius of the planet, and thus parameters such as density.
What Saxena and team are interested in is how rocky worlds orbiting red dwarf stars may experience stresses that can change their shape. Saxena comments:
“Imagine taking a planet like the Earth or Mars, placing it near a cool red star and stretching it out. Analysing the new shape alone will tell us a lot about the otherwise impossible to see internal structure of the planet and how it changes over time.”
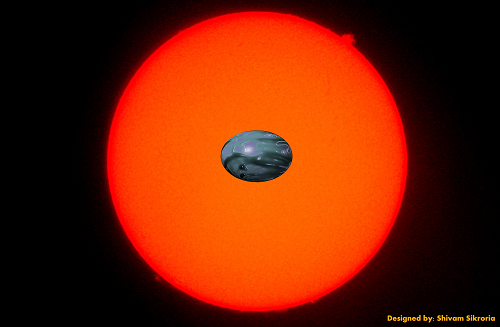
Image: An artist’s impression of a stretched rocky planet in orbit around a red dwarf star. So close to the star, there is a difference in the strength of the gravitational field on each side of the planet, stretching it significantly. Credit: Shivam Sikroria.
The paper, which appears in Monthly Notices of the Royal Astronomical Society, goes to work on how to take both tidal and rotational forces into account. The French astronomer Édouard Roche (1820-1883) was the first to calculate the distance within which an orbiting body will disintegrate because of the tidal forces induced by its primary. Inside what we now call the Roche limit, material in orbit will become dispersed into rings, while outside the limit, it can coalesce. The process varies depending on the innate rigidity of the body in question. A more fluid world deforms gradually, a process that compounds the tidal forces that will destroy it, while a more rigid planet may hold its shape until being broken apart by these same forces.
All of this could be useful as we try to learn more about the planet’s characteristics. From the paper:
The variation of rigidity of a planet may produce a small but detectable signal in the cases that were tested as one gets very close to the fluid Roche limit, and again it is important to remember planets have also been detected interior to the fluid Roche limit (the inner distance bound). Merely the constraining of tidal bulge amplitude along with Roche limit considerations may put meaningful limits on interior structure. The ability to directly constrain the shape of a planet would provide clues towards tidal theory, the orbital configuration of the system and bulk properties of the planet.
Not many M-dwarf planetary systems are likely to show the signature of worlds near the Roche limit, but the paper argues that the general physical principles in play here may also help us interpret the signatures of planets in particular orbital resonances or other configurations. Several dozen ‘hot Jupiters’ have been found that should, by virtue of their proximity to the Roche limit, show observable effects. For solid planets, the large transit depth may make red dwarf planets near the Roche limit an excellent realm for further study as we learn to interpret what any planetary deformations can tell us about their internal characteristics.
The paper is Saxena et al., “The observational effects and signatures of tidally distorted solid exoplanets,” published online by Monthly Notices of the Royal Astronomical Society 14 December 2014 (abstract / preprint).
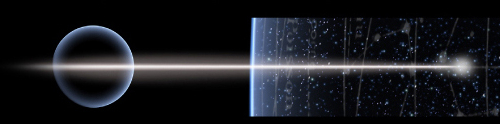
by Paul Gilster | Dec 15, 2014 | Asteroid and Comet Deflection |
The impact at Tunguska, Siberia on June 30,1908, evidently a small asteroid, devastated 1300 square kilometers, which works out to be the equivalent of a large metropolitan area. June 30, 2015 is thus an appropriate date to launch Asteroid Day, a global awareness campaign to put the issue of dangerous impacts in front of as many people as possible. An early December press conference at the London Science Museum, hosted by Lord Martin Rees, UK Royal Astronomer, announced the campaign and released a declaration of needed action:
- Employ the available technology to detect and track near-Earth asteroids that threaten human populations
- A rapid hundred-fold (100x) acceleration of the discovery and tracking of NEOs
- Global adoption of Asteroid Day on June 30, 2015, to heighten awareness of the asteroid hazard and our efforts to prevent future impacts
The list of scientists, business leaders and artists behind the 100x Declaration, as it’s being called, is an impressive one that includes Jill Tarter, Kip Thorne, Stewart Brand, Richard Dawkins, Google’s Peter Norvig, astrophysicist and guitarist Brian May, Alexei Leonov, Jim Lovell and Rusty Schweickart, a group numerous and diverse enough that I’ll send you to the list of signatories available on the Asteroid Day website for more. Founding partners of the event include The Planetary Society, Astronomy Magazine, the Association of Space Explorers (ASE), and the California Academy of Sciences. ASE chairman Tom Jones explains:
“Finding hazardous asteroids early through an accelerated search program is the key to preventing future destructive impacts. The 100x Declaration will focus space policymakers on that important goal. ASE called last year for a stepped-up, global search effort; this can lead within a decade to an international deflection demonstration mission to show we know how to nudge an asteroid. Once we know what’s coming, we can design an effective space deflection campaign against dangerous objects we find.”
Visualizing Asteroid Data
In November, NASA’s Near Earth Object Program released a map showing a visualization of data gathered between 1994 and 2013. Atmospheric impacts large enough to produce a fireball occurred on 556 occasions during this period, with almost all the small asteroids disintegrating before they reached the ground. The most prominent exception is the Chelyabinsk event in 2013, the largest asteroid to cause surface damage during this period. We learn that asteroids no larger than a meter in diameter hit the atmosphere and break apart about every other week. The Chelyabinsk event was caused by the explosion in the atmosphere of an asteroid thought to be about 20 meters in size.
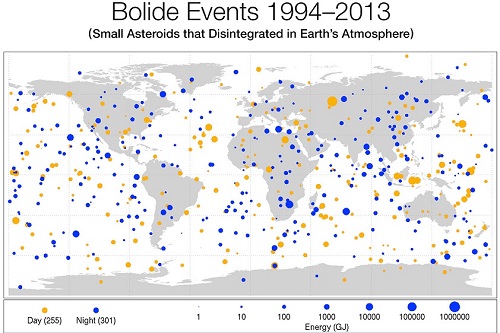
Image: This diagram maps the data gathered from 1994-2013 on small asteroids impacting Earth’s atmosphere to create very bright meteors, technically called “bolides” and commonly referred to as “fireballs”. Sizes of red dots (daytime impacts) and blue dots (nighttime impacts) are proportional to the optical radiated energy of impacts measured in billions of Joules (GJ) of energy, and show the location of impacts from objects about 1 meter (3 feet) to almost 20 meters (60 feet) in size. Credit: Planetary Science.
The good news is that the Earth’s atmosphere screens small asteroids from the surface quite effectively, but Lindley Johnson (NASA NEO Observations Program) says that data like these will help us figure out how often asteroids large enough to cause ground damage do get through. According to this JPL news release, the NEO Observations Program has identified 96 percent of the estimated population of one-kilometer or larger asteroids, with a goal of finding 90 percent of NEO’s larger than 140 meters in diameter. These are estimated to be as much as 25 times more numerous than the one kilometer asteroids. The NEO Observations Program finds and tracks asteroids whose orbits bring them within 50 million kilometers of Earth’s orbit.
Two other notes: A 2013 paper from Peter Brown and team reports that existing telescopic surveys have discovered about 500 near-Earth asteroids that are 10-20 meters in diameter — that’s Chelyabinsk size — out of an estimated near-Earth asteroid population of roughly 20 million, “… implying that a significant impactor population at these sizes could be present but not yet cataloged in the discovered near-Earth asteroid population.” All of which gives the 100x Declaration some punch and underscores how much we still have to do.
And in a paper just released on the arXiv site, Clemens Rumpf (University of Southampton, UK) unveils ARMOR, the Asteroid Risk Mitigation Optimization and Research tool currently being developed at the university. The tool calculates the impact location and probability distribution on Earth’s surface (the so-called ‘risk corridor’), using (in this paper) a sample size of ten asteroids. Future iterations of ARMOR may prove useful in assessing the risk of individual asteroids.
The Brown paper is “The flux of small near-Earth objects colliding with the Earth,” Nature, Vol. 420 (21 Nov. 2002), pp. 294-296 (abstract). The Rumpf paper (thanks to Ashley Baldwin for the tip) is “Global Asteroid Risk Analysis” (abstract).
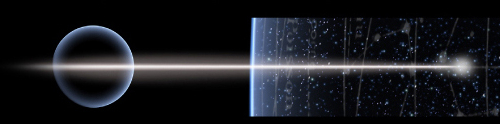
http://www.nature.com/nature/journal/v420/n6913/abs/nature01238.html
http://neo.jpl.nasa.gov/news/news186.html
http://www.asteroidday.org/signatories-list
by Paul Gilster | Dec 12, 2014 | Uncategorized |
Lunar Mission One is an interesting private attempt to put a payload on the lunar surface, a crowdsourced project aimed at doing good science and deepening public participation in spaceflight. Remembering the Apollo days, I’m always interested in seeing what can be done to renew interest in space, and having the chance to make a contribution toward such a self-starting space mission is undeniably attractive. As witness Lunar Mission One’s pitch on Kickstarter, which has aimed for an ambitious £600,000 and has already raised £520,341.
That figure is as of this morning, with five days to go in the attempt, and it’s clear enough that £600,000 won’t buy a lunar mission of considerable complexity, as this one is. But it’s enough to take an effort that has been seven years in the building to the next level, which means establishment of working management teams and the beginning of procurement planning and risk assessment. That turns what has been a part-time volunteer project into a full-time effort.
Writing about a lunar mission is a bit out of the norm for Centauri Dreams, where I decided from the beginning to adopt a focus on the outer planets and beyond, meaning deep space technologies that could lead to an eventual interstellar effort and all that entails. But what caught my eye about Lunar Mission One is that there is a ‘deep time’ aspect to the whole thing, one that looks past short-term results to think about the future of the species and how we can connect to it. The connection grows out of Lunar Mission One’s ambitious science agenda.
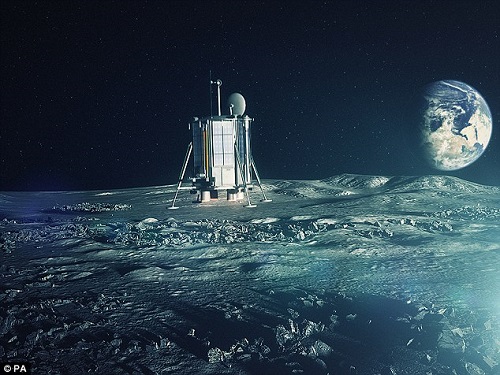
Going Deep at the Pole
To explain that, let me discuss the larger plan: The Lunar Mission One probe will land at the lunar south pole. Aboard it will be a 2-meter drill connected by cable to the spacecraft, with which controllers will remotely drill a 5-centimeter borehole. The plan here is to retrieve cylindrical rock cores for analysis by instrumentation aboard the craft, and the drilling time is envisioned as lengthy — three to four months — until a target depth of 20 meters has been achieved, although the planners speculate that the drill might reach depths up to 100 meters.
This sets up some interesting science, for even the nominal 20 meter goal takes us into unexplored terrain — I believe the deepest we’ve reached from any spacecraft on the Moon is no more than a couple of meters. Enormously useful geological measurements could result and may help us better understand elements of the early Solar System’s history, including the late heavy bombardment period. Moreover, Lunar Mission One should be able to study the surrounding surface at the pole, analyzing any local materials that might aid a future human base.
But it’s what happens when the drilling is done that particularly interests me. When I spoke of ‘deep time,’ I was referring to the plan to use the borehole — after the science goals have been achieved — to lower a publicly assembled archive of life on Earth, the history of our species and a database stuffed with information about our biosphere, deep below the lunar surface. The substantial protection provided by tens of meters of lunar regolith should make this an unusually long-lasting capsule, one the planners believe can remain intact for perhaps a billion years.
Lunar Mission One also has a private archive, what it describes as millions of individual ‘memory boxes’ to which contributors to the project will be able to upload data. Longer-term funding for the mission, it is hoped, will grow out of what will become a ten-year opportunity to sell these memory boxes, which could contain photos, video, audio or any other digital information. The plan is to store tens of terabytes of such material within the private archive, with larger contributions to the project allowing the donor to buy larger amounts of storage.
To boost donations, individual memory boxes might do the trick, but my interest is in the development of the public archive, which forces decisions about how we view ourselves, what our priorities are, and what we choose to be preserved for an unknowable future generation to find. The assembly of that kind of content is a fascinating process, one that Centauri Dreams regular Heath Rezabek has devoted himself to developing through his Vessels project, which you can read about in posts like Deep Time: The Nature of Existential Risk.
The term ‘deep time’ invokes Gregory Benford’s 1999 book of that name (Deep Time: How Humanity Communicates Across Millennia), which in turn harkens back to a 1992 paper in which Benford discussed a program of freezing and preserving species in threatened ecospheres as a response to the loss of biodiversity in our era. What can we do, Benford asked, to create such a ‘Library of Life,’ and how should we assemble the samples? All this is discussed in the later book within the context of how humans communicate with each other across historical eras, questions raised by the great monuments of history, and by engineering projects like storage sites for radioactive materials that must long outlive their makers.
Lunar Mission One is exploring this terrain with its announced purpose of creating an archive that, if I read its Kickstarter page correctly, will be assembled using its core crowdsourcing methods. In my judgement, assembling a representation of our species is a highly productive exercise. Think of the Voyager Golden Record, or the ongoing private attempt to craft an archive that will be uploaded to New Horizons after its outer system mission is complete, if NASA signs off on the plan. We question our basic priorities when we look long-term, gaining perspective on the values that count, and perhaps learning where our strongest efforts need to be re-focused.
Long-haul archives, in other words, are not only about the future, but about our ability to make course corrections as we back off to view ourselves in the context of history and of nature.
So good luck to Lunar Mission One. We need projects that look at these matters. We need not only single ‘time capsule’ archives but a host of archival sites that are designed, like the Long Now Foundation’s 10,000 Year Clock, to last into a deep future against which our own lifetimes are acted out on the smallest of scales. If selling personal memory boxes is what it takes to get a serious public archive buried deep within the Moon, then let’s hope this one of many possible archives can be completed as planned, a gesture to the future from its myriad creators.
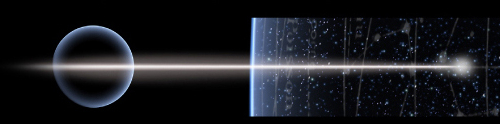
by Paul Gilster | Dec 11, 2014 | Outer Solar System |
Where did the water in Earth’s oceans come from? It’s an open question, but new data from the Rosetta mission, in particular its ROSINA instrument (Rosetta Orbiter Spectrometer for Ion and Neutral Analysis) indicate that terrestrial water probably did not come from comets like 67P/Churyumov-Gerasimenko, around which Rosetta has been orbiting since August. There is little doubt that water reached Earth through bombardment from small bodies early in the planet’s history, but the Rosetta findings sharpen the question of where these objects came from.
Image: This composite is a mosaic comprising four individual NAVCAM images taken from 19 miles (31 kilometers) from the center of comet 67P/Churyumov-Gerasimenko on Nov. 20, 2014. The image resolution is 10 feet (3 meters) per pixel. Credit: ESA.
At work here is analysis of the ratio between hydrogen and deuterium, a heavy form of hydrogen with one proton and one neutron in the nucleus (common hydrogen lacks the neutron). This D/H ratio on 67P/Churyumov-Gerasimenko turns out to be over three times the terrestrial value, among the highest values yet measured in the Solar System, making it unlikely that comets like these supplied Earth’s oceans. Back in 1986, the European Giotto mission to comet Halley found a D/H ratio twice that of Earth’s, seeming to rule out Oort Cloud comets as the source.
67P/Churyumov-Gerasimenko and Halley are two different kinds of comet, so let’s explore this. Cometary origins are complex because of interactions in the early Solar System. Long-period comets that swing into the inner system from the distant Oort Cloud are thought to have formed originally somewhere beyond the snowline in the region where Uranus and Neptune now orbit, to be scattered by subsequent gravitational interactions with the large outer planets. 67P/Churyumov-Gerasimenko is a Jupiter-family comet, a class whose origins are believed to lie beyond Neptune in the Kuiper Belt. Comets like these sometimes have their orbits disrupted so that they fall under the gravitational influence of Jupiter, hence their designation.
In contrast to 67P/Churyumov-Gerasimenko, comet Hartley 2, which was examined by the European Space Agency’s Herschel spacecraft in 2011, turned out to have a deuterium/hydrogen ratio similar to Earth’s. The discrepancy is surprising because Hartley 2 is also a Jupiter-family comet, and models of the early Solar System produce a D/H ratio for these comets more like 67P/Churyumov-Gerasimenko, even higher than their Oort Cloud cousins.
The disparity may indicate that we know less about Jupiter-family comets and their origins than we realized. The new Rosetta data tell us that this family of comets is hardly uniform, and thus nudge us away from them and toward asteroids as the primary water delivery mechanism. Kathrin Altwegg (University of Bern) is principal investigator for the ROSINA instrument and lead author of the paper on this work, which has just appeared in Science:
“This surprising finding could indicate a diverse origin for the Jupiter-family comets – perhaps they formed over a wider range of distances in the young Solar System than we previously thought. Our finding also rules out the idea that Jupiter-family comets contain solely Earth ocean-like water, and adds weight to models that place more emphasis on asteroids as the main delivery mechanism for Earth’s oceans.”
The measurements in question were made in the first month after Rosetta’s arrival at 67P/Churyumov-Gerasimenko. With the Rosetta data, we now have D/H information about eleven comets, finding that only Hartley 2 shows a ratio matching the composition of Earth’s water. We also know that meteorites with an origin in the asteroid belt show a good fit with Earth’s D/H ratio. Although the overall water content of asteroids is lower than we find in comets, a large number of asteroid impacts could account for the primary delivery mechanism.
Meanwhile, the mission continues, and will for some time. Rosetta has now been at 67P/Churyumov-Gerasimenko for 127 days. Perihelion is 244 days away.
The paper is Altwegg et al., “67P/Churyumov-Gerasimenko, a Jupiter family comet with a high D/H ratio,” published online in Science 10 December 2014 (abstract). This ESA news release is helpful.
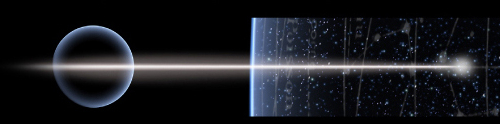
by Paul Gilster | Dec 10, 2014 | Culture and Society |
My friend Frank Taylor was in town over the Thanksgiving holiday, having flown in from South Africa. With his wife Karen, Frank has spent the years since 2009 circumnavigating the globe aboard a 50-foot catamaran called Tahina, an adventure chronicled with spectacular photography on the Tahina Expedition blog. I highly recommend the site for anyone interested in travel and the sea, not to mention how high tech has transformed the ancient art of sailing. But when we spoke recently just before Frank returned to Africa, he had another kind of high tech in mind. Specifically, what had I thought about the film Interstellar?
I haven’t delayed my comments on the movie intentionally, but I was slow in getting to see it, missing the opportunity at the end of the Tennessee Valley Interstellar Workshop and then getting involved in recent activities including the One Earth New Horizons Message workshop at Stanford. I also wanted to read Kip Thorne’s The Science of Interstellar (Norton, 2014) and give the movie a second viewing. All that behind me, it’s time to explain why I was surprised by Interstellar. My expectations for Hollywood science fiction are always low, as I’ve found that today’s filmmaking wizardry all too often masks serious flaws in plot and character.
I can find problems in Interstellar as well, as many reviewers have, but I think this is an important movie whose mistakes aren’t significant compared to what it accomplishes. Interstellar is a movie that will baffle a large part of its audience, the movie-going public far more comfortable with battles in space and sleek starships like the Enterprise. This is not an audience that will easily follow the twists and turns through time and space that Christopher Nolan has created. But Nolan’s attention to detail, his partnership with Thorne, and his insistence on scientific plausibility wherever possible will get through to an important subset.
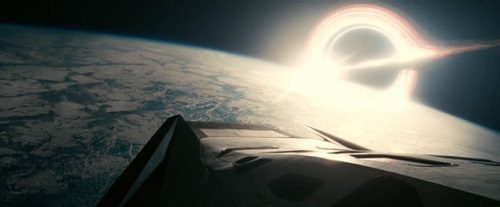
I’m talking about younger people with an interest in science for whom the movie’s stunning visuals will impel them to learn how to untangle the plot. For the intellectually curious, Interstellar is a tandem creation, a movie/book duality where each plays off the other — the motivated minority will want to immerse themselves in both. The film’s plot is demanding and operates at various degrees of believability, but there is a science puzzle to be untangled here, one to which Thorne’s book offers the key. When I was researching Centauri Dreams back in 2002-2004, I was surprised at how many scientists and aerospace engineers told me they had chosen their fields because of science fiction novels like Poul Anderson’s Tau Zero. This movie will have the same effect. In fact, I can think of no movie that is so likely to create careers in the sciences, particularly physics, than this one.
Let’s talk about why. Here I get into details and suppose I should say that there may be spoilers below for those who haven’t yet seen Interstellar, so proceed with caution. I could focus on various issues, from a worldwide blight to passage through a wormhole, but the film’s treatment of black holes is what I’ll use as my working material. The wormhole itself, out near Saturn’s orbit and an apparent way out for a desperate humanity, is richly described in Thorne’s chapters, especially with regard to the special effects that bring it to life.
Our protagonist, Cooper, must learn which of three planets in an unusual system dominated by an enormous black hole called Gargantua is most suited for humans. When he and his team need to get to one of these, Miller’s Planet, from their parking orbit near the black hole, they have to produce huge velocity changes in the range of 100,000 kilometers per second. Thorne goes through the physics of this, including the bizarre time distortion on a world so close to a black hole. The upshot is that a gravitational slingshot maneuver must be performed. The movie skims over the matter quickly, but Cooper does discuss how this must have been done later, when he talks about using a neutron star to decelerate. The neutron star is a bit of a fudge — it turns out it wouldn’t be big enough to force the needed maneuver, but an intermediate-mass black hole would do the trick. Thorne made this case to Nolan but the director stuck with the neutron star.
That’s a scientific error forced by Hollywood values. Thorne relates his objections to Nolan during the re-writing of the screenplay and goes on to explain what happened:
…he [Nolan] didn’t want to confuse his mass audience by having more than one black hole in the movie. One black hole, one wormhole, and also a neutron star, along with Interstellar‘s other rich science, all to be absorbed in a fast-paced two hour film; that was all Chris thought he could get away with. Recognizing that strong gravitational slingshots are needed to navigate near Gargantua, Chris included one slingshot in Cooper’s dialog, at the price of using a scientifically implausible deflector: the neutron star instead of a black hole.
Interstellar is closer to three hours than two (Thorne was writing before the film was finalized), but does anything else about this bother you? I’m going to argue that the combination of Nolan’s movie and Thorne’s book — and the fruitful collaboration the two engaged in throughout — induces the kind of puzzle-solving ethos that will prompt many a young mind to dig deeper into the movie’s physics. Nolan made a choice with a mass audience in mind, but the framework of that choice is laid bare in Thorne’s book, which goes on to explain how careful Nolan was to stick to scientific plausibility where he felt that he could. Filmmaking is always a matter of compromise, but Nolan was surprisingly tough. Finding where Interstellar works and where it stretches science out of shape is itself a lesson in problem solving.
The point is, the director was thinking about these things within a framework that, in terms of its spectacular visuals, is meticulous about getting the larger details right. Think about the accretion disk around the black hole Gargantua. Here we’re seeing a magnetic field in the process of converting gravitational energy into heat and then light — the field, explains Thorne, provides the friction that slows the circumferential motion of gas in the disk even as the black hole’s gravity tries to speed up the infalling material, with kinetic energy being converted into heat and light along the way. But go to the projections of black hole accretion disks you’ll find on the Net or in technical publications and you’ll find Nolan’s depiction is far more spectacular. Another Hollywood-enforced choice?
No. What Nolan and Thorne added was the gravitational lensing of the disk by its own black hole, an effect explored through computer code developed for the film. While you would expect that portion of the disk behind the black hole to be out of sight, gravitational lensing produces two images of it, one above and the other below Gargantua. This is special effects wizard Eugénie van Tunzelmann’s work, carefully wrought to produce the nested effect Thorne describes:
Inside these primary images, we see thin secondary images of the disk, wrapping over and under the shadow, near the shadow’s edge. And if the picture were made much larger, you would see tertiary and higher-order images, closer and closer to the shadow.
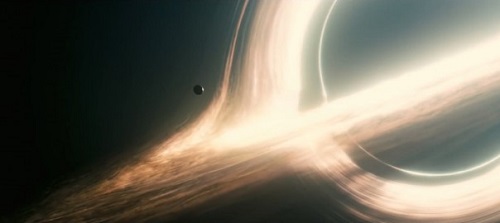
Moreover, Gargantua’s distortion of spacetime distorts the disk images, pushing the perceived disk away from the shadow on one side and toward the shadow on the other, creating an effect that appears lopsided. Here again Nolan intervened in an attempt to avoid confusion, especially as the audience tried to work out the reason for the lopsidedness of the disk and the star patterns created near its edge. So for the purposes of the imagery, he slowed the spin of Gargantua to reduce the effect, just as van Tunzelmann removed the Doppler shift created by the disk’s motion, which would have created an even more lopsided and confusing effect.
What pleases me here is that these changes and their rationale are thoroughly explored in Kip Thorne’s book, which likewise offers those intrigued with such visualizations the opportunity to explore how they could be fine-tuned and rendered with greater accuracy. Given the Hollywood culture in which he operates, I think that Christopher Nolan produced a movie with as much scientific accuracy as he could get past his producers, given the imperative for ratings and box office sales.
Most people are going to be wowed by the visuals; some will be dismayed by the all but supernatural intervention of beings from a higher dimension who may be our descendants. But a few, the ones I’m focused on, are going to use the high points of this movie — its unapologetic call for exploration, its entanglement with science as a form of quest — to choose to learn more, and there is no more engaging a guide than Kip Thorne to show them the way. Thorne says he was moved by the underlying message of Interstellar that the universe can be viewed optimistically because our species is capable of choosing its future:
But doing so, controlling our own fate, requires that a large fraction of us understand and appreciate science: How it operates. What it teaches us about the universe, the Earth, and life. What it can achieve. What its limitations are, due to inadequate knowledge or technology. How those limitations may be overcome. How we transition from speculation to educated guess to truth. How extremely rare are revolutions in which our perceived truth changes, yet how very important.
Thirty years from now there will be working scientists who explain to interviewers how Interstellar, a movie flawed by occasional mawkishness (think of Amelia Brand’s regrettable lines about love being the fifth dimension), weighed down by what may be the ultimate deus ex machina (in the form of Cooper operating through the tesseract), and reactive to Hollywood’s relentless popular ethos, nonetheless captured their imagination so that they read a book (Thorne’s) that helped to launch them down a path whose end they could not imagine. I call that a fine result, and reiterate my surprise in finding a Hollywood blockbuster I can seriously recommend.
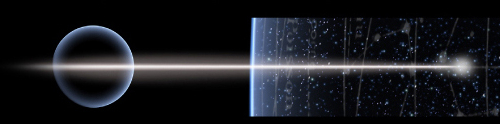