by Paul Gilster | Dec 9, 2014 | Outer Solar System |
No spacecraft has ever traveled further to reach its primary target than New Horizons, now inbound to Pluto/Charon. From 4.6 billion kilometers from Earth (four hours, 26 minutes light travel time), the spacecraft has sent confirmation that its much anticipated wake-up call from ground controllers was a success. Since December 6, New Horizons has been in active mode, a state whose significance principal investigator Alan Stern explains:
“This is a watershed event that signals the end of New Horizons crossing of a vast ocean of space to the very frontier of our solar system, and the beginning of the mission’s primary objective: the exploration of Pluto and its many moons in 2015.”
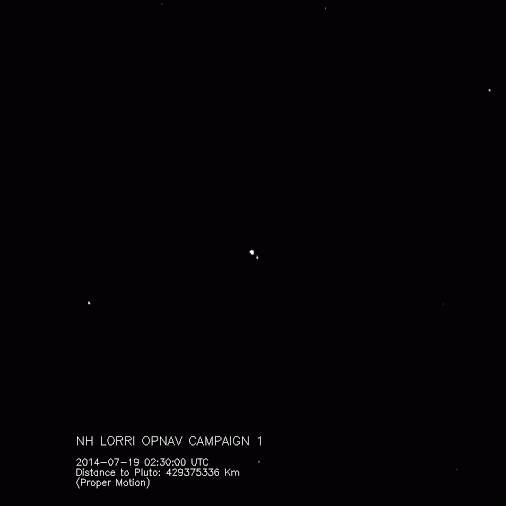
Image: Pluto and Charon, in imagery taken by New Horizons in July of 2014. Covering almost one full rotation of Charon around Pluto, the 12 images that make up the movie were taken with the spacecraft’s best telescopic camera – the Long Range Reconnaissance Imager (LORRI) – at distances ranging from about 429 million to 422 million kilometers. Credit: NASA/Johns Hopkins University Applied Physics Laboratory/Southwest Research Institute.
Not that hibernation is an unusual event for the spacecraft. We’ve followed several cycles here on Centauri Dreams, but it’s startling to realize that New Horizons has gone through eighteen hibernation periods, with two-thirds of its flight time in that state. A weekly beacon stayed alive during these periods, as did the onboard flight computer that broadcast its status tone, but much of the spacecraft was powered down to protect system components. For this special awakening, English tenor Russell Watson recorded a special version of ‘Where My Heart Will Take Me,’ which was played in New Horizons mission operations upon wake-up confirmation.
Pluto observations begin on January 15, with closest approach on July 14, and by mid-May, we will begin receiving views of Pluto and its moons that are higher in quality than anything the Hubble Space Telescope has yet given us. More on the awakening of New Horizons on this JHU/APL page. Meanwhile, the private effort to upload a message from Earth to New Horizons following the end of its science mission continues. If NASA gives the go-ahead, Jon Lomberg’s team will crowdsource content for the One Earth New Horizons Message. A workshop discussing message methods and content just concluded last week at Stanford.
The View from the Asteroid Belt
Speaking of images better than Hubble, we’ve certainly managed that with the Dawn mission, which brought us spectacular vistas from Vesta during its fourteen months in orbit around the asteroid. Now we can look forward to topping Hubble’s views of Ceres, the spacecraft’s next target. Below is an image that, while not yet better than Hubble can manage, does give us an idea of the spherical shape of the asteroid (click to enlarge). Dawn is now 1.2 million kilometers out, about three times the Earth-Moon distance from its target.
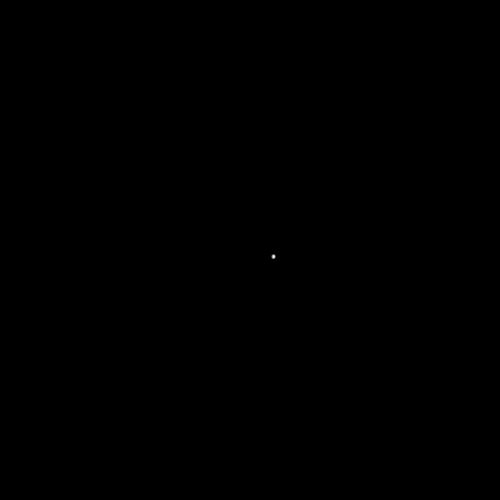
Image credit: NASA/JPL-Caltech/UCLA/MPS/DLR/IDA.
Dawn will be captured into orbit around Ceres in March, but by early 2015, we’ll be seeing images at higher resolution than Hubble has yet provided. The approach phase begins on December 26, with the nine-pixel-wide image just released serving as a final calibration of the spacecraft’s science camera. With both missions, we are pushing into unknown territory, about to see things in greater detail than our best telescopes can offer. A bit of the old Voyager and Pioneer feeling has me in its grip, a confirmation of our human need to explore and a validation of all the hard work that got us here.
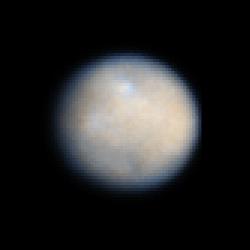
At left is Ceres in the best photo we currently have, a color Hubble image. These observations were made in both visible and ultraviolet light between December 2003 and January 2004, showing brighter and darker regions that may be impact features or simply different types of surface material. 950 kilometers across, Ceres may have an interior differentiated between an inner core, an ice mantle, and a relatively thin outer crust.
Every time I see this image I remember Alfred Bester’s The Stars My Destination, a 1956 novel in which protagonist Gulliver Foyle takes the pseudonym Fourmyle of Ceres as part of the unfolding of his ingenious plan for revenge. The asteroid pops up in many science fiction tales (Larry Niven’s ‘Known Space’ stories come particularly to mind), but perhaps none as compelling as the one Dawn is about to tell us.
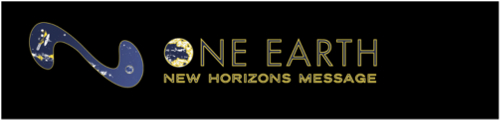
by Paul Gilster | Dec 8, 2014 | Exoplanetary Science |
We’re going to be bringing both space- and ground-based assets to bear on the detection of rocky planets within the habitable zone in coming years. Cool M-class stars (red dwarfs) stand out in this regard because their habitable zones (in this case defined as where water can exist in liquid form on the surface) are relatively close to the parent star, making for increased likelihood of transits as well as a larger number of them in a given time period. Ground observatories like the Giant Magellan Telescope and the European Extremely Large Telescope should be able to perform spectroscopic studies of M-class planets as well.
So consider this: We have a high probability for planets around these stars (see Ravi Kopparapu’s How Common Are Potential Habitable Worlds in Our Galaxy?), and then factor in what Ramses Ramirez and Lisa Kaltenegger have, the fact that before they reach the main sequence, M-class stars go through a period of ‘infancy’ that can last up to 2.5 billion years. It’s an important period in the life of any planets around them because, as we saw last week in a paper by Rodrigo Luger and Rory Barnes, huge water loss can occur and there is the possibility for runaway greenhouse events that complicate a planet’s habitability.
But we need to keep digging at this issue because early M-dwarf planets are interesting targets for detection. In their new paper, Ramirez and Kaltenegger (Cornell University) point out that in the period before entering the main sequence, these stars have a luminosity much higher relative to their early main sequence values than F or G stars. In fact, an M8 star can be 180 times as bright during its contraction stage as it will be when entering the main sequence. Our G-class Sun, by contrast, was only twice as bright during this period.
What we get is a habitable zone that is located further away from the young star than we would otherwise expect, in a pre-main sequence period that, for some of the smallest stars, can last for over two billion years, meaning there is the potential for life to develop on the surface, perhaps moving below ground as the star’s luminosity gradually declines. The paper computes the luminosities of stars in classes F through M as they go through the contracting phase and onto the main sequence, with clear effects on the orbital distance of the habitable zone around the star.
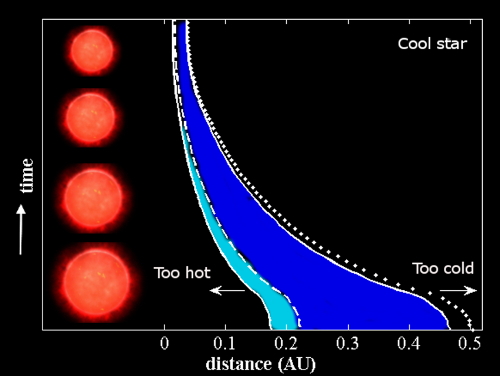
Image: Changes in the position of the habitable zone over time. Credit: L. Kaltenegger, R. Ramirez/Cornell University.
The coolest of these stars, an M8 dwarf, shows an inner zone of the HZ at 0.16 AU that moves to 0.01 AU at the end of the 2.5 billion year pre-main sequence phase. In the same period, the outer edge of the HZ moves from 0.45 AU to 0.3 AU. Contrast that with our Sun, where the inner HZ edge changes from 1 AU to 0.6 AU at the end of the pre-main sequence stage after a comparatively swift 50 million years. The Sun’s outer HZ edge moves from 2.6 AU to 1.5 AU. Variations in planetary mass appear to have only a small effect on these results.
The potential for water loss on the planets of young M-class stars is enormous, much higher for this than other stellar types. Ramirez and Kaltenegger shows that planets orbiting an M8 star at distances corresponding to the inner and outer main sequence habitable zone could lose up to 3800 and 225 Earth oceans of water respectively. An M5 planet loses 350 and 15 oceans respectively, while an M1 world loses 25 and 0.5 oceans. If we look at K5 and G2-class stars, their planets at the inner and outer habitable zone lose 1.3 and 0.75 Earth oceans respectively. These figures, remember, are for planets that end up being in the habitable zone later in the star’s life, when it is firmly established on the main sequence.
Is this water loss a fatal blow to the planet’s chances for habitability? Ramirez and Kaltenegger think not, a point that is worth emphasizing. From the paper:
Our results… suggest that planets later located in the MS HZ orbiting stars cooler than ~ a K5 may lose most of their initial water endowment. However M-dwarf MS HZ planets could acquire much more water during accretion than did Earth (Hansen, 2014), or water-rich material could be brought in after accretion, through an intense late heavy bombardment (LHB) period similar to that in our own Solar System (Hartmann 2000; Gomes et al. 2005). The second mechanism requires that both the gas disk has dissipated and the runaway greenhouse stage has ended.
The situation of our own Earth is not without interest here. Ramirez commented on the issue in this Cornell news release:
“Our own planet gained additional water after this early runaway phase from a late, heavy bombardment of water-rich asteroids,” says Ramirez. “Planets at a distance corresponding to modern Earth or Venus orbiting these cool stars could be similarly replenished later on.”
We can also factor in possible planetary migration, bringing planets rich in volatiles into the habitable zone after the star has reached the main sequence. Bear in mind that the pre-main sequence period can last 195 million years for an M1 star and up to 2.42 billion for a cool M8, meaning that a planet that has lost its water through this ‘bright young star’ period could regain water and become habitable even before the star reaches the main sequence.
In terms of searching for small, rocky planets with the potential for life, then, we need to keep in mind how much farther out the habitable zone is during a young star’s pre-main sequence phase, for the larger separation between planet and star will make such planets easier to find with next-generation telescopes. This work shows that planets around stars in spectral type K5 and cooler — those that will later be located in the habitable zone during the star’s main sequence phase — can easily receive enough stellar flux to lose much or all of their water. They will need to accrete more water than Earth did or else rely on water delivered through later bombardment. They are prime candidates for remote observation and characterization.
Moreover, we learn here that planets outside the habitable zone during the star’s stay on the main sequence may once have had conditions right for life to develop, with the potential for continuing beneath the surface as the star cools. “In the search for planets like ours out there, we are certainly in for surprises,” says Kaltenegger, and it’s clear that young stars, especially M-dwarfs, offer rich targets for observation as we parse out what makes a planet habitable.
The paper is Ramirez and Kaltenegger, “The Habitable Zones of Pre-Main-Sequence Stars,” in press at Astrophysical Journal Letters (preprint).
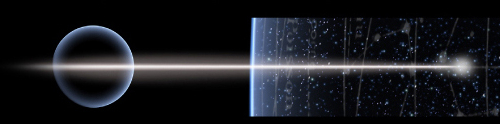
by Paul Gilster | Dec 5, 2014 | Culture and Society |
What trajectory will our civilization follow as we move beyond our first tentative steps into space? Nick Nielsen returns to Centauri Dreams with thoughts on multi-generational projects and their roots in the fundamental worldview of their time. As the inspiring monuments of the European Middle Ages attest, a civilizational imperative can express itself through the grandest of symbols. Perhaps our culture is building toward travel to the stars as the greatest expression of its own values and capabilities. Is the starship the ultimate monument of a technological civilization? In addition to continuing work in his blogs Grand Strategy: The View from Oregon and Grand Strategy Annex, Nielsen heads Project Astrolabe for Icarus Interstellar, described within the essay.
by J. N. Nielsen
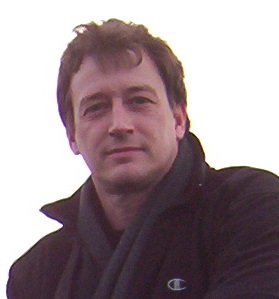
If interstellar flight proves to be possible, it will be possible only in the context of what Heath Rezabek has called an Interstellar Earth: civilization developed on Earth to the degree that it is capable of launching an interstellar initiative. This is Earth, our familiar home, transformed into Earth, sub specie universalis, beginning to integrate its indigenous, terrestrial civilization into its cosmological context.
Already life on Earth is thoroughly integral with its cosmological context, in so far as astrobiology has demonstrated to us that the origins and processes of life on Earth cannot be fully understood in isolation from the Earth’s cosmic situation. The orbit, tilt, and wobble of the Earth as it circles the sun (Milankovitch cycles), the other bodies of the solar system that gravitationally interact with (and sometimes collide with) Earth, the life cycle of the sun and its changing energy output (faint young sun problem), the position of our solar system with the galactic habitable zone (GHZ), and even the solar system bobbing up and down through the galactic plane, subjecting Earth to a greater likelihood of collisions every 62 million years or so (galactic drift) – all have contributed to shaping the unique, contingent history of terrestrial life.
What other distant unknowns shape the conditions of life here on Earth? We cannot yet say, but there may be strong bounds on the earliest development of complex life in the universe, other than the age of the universe itself. For example, recent research on gamma ray bursts suggests that the universe may be periodically sterilized of complex life, as argued in the paper On the role of GRBs on life extinction in the Universe by Tsvi Piran and Raul Jimenez (and cf. my comments on this), which was also the occasion of an article in The Economist, Bolts from the blue. Intelligent life throughout the universe may not be much older than intelligent life on Earth. This argument has been made many times prior to the recent work on gamma ray bursts, and as many times confuted. A full answer must await our exploration of the universe, but we can begin by narrowing the temporal boundaries of possible intelligence and civilization.
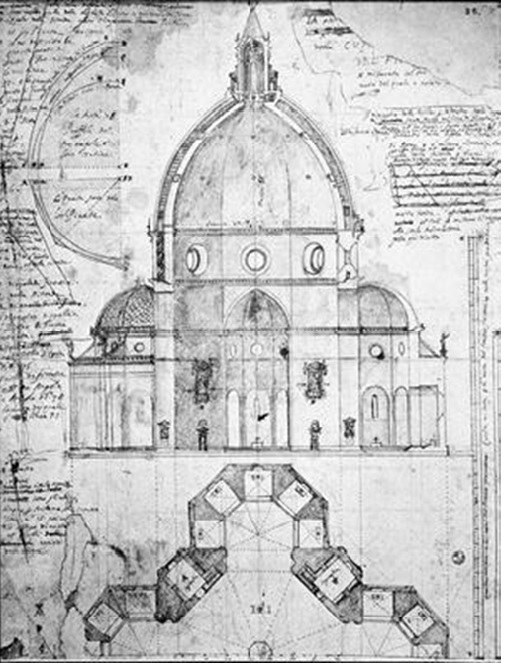
Image: Lodovico Cardi, also known as Cigoli, drawing of Brunelleschi’s Santa Maria del Fiore, 1613.
From the perspective of natural history, there is no reason that cognitively modern human-level intelligence might not have arisen on Earth a million years earlier than it did, or even ten million years earlier than it did. On the other hand, from the same perspective there is no reason that such intelligence might not have emerged for another million years, or even another ten million years, from now. Once biology becomes sufficiently complex, and brains supervening upon a limbic system become sufficiently complex, the particular time in history at which peer intelligence emerges is indifferent on scientific time scales, though from a human perspective a million years or ten million years makes an enormous difference. [1]
Once intelligence arose, again, it is arguably indifferent in terms of natural history when civilization emerges from intelligence; but for purely contingent factors, civilization might have arisen fifty thousand years earlier or fifty thousand years later. Hominids in one form or another have been walking the Earth for about five million years (even if cognitive modernity only dates to about 60 or 70 thousand years ago), so we know that hominids are compatible with stagnation measured on geological time scales. Nevertheless, the unique, contingent history of terrestrial life eventually did, in turn, give rise to the unique, contingent history of terrestrial civilization, the product of Earth-originating intelligence.
The sheer contingency of the world makes no concessions to the human mind or the needs of the human heart. Blaise Pascal was haunted by this contingency, which seems to annihilate the human sense of time: “When I consider the short duration of my life, swallowed up in the eternity before and after, the little space which I fill and even can see, engulfed in the infinite immensity of spaces of which I am ignorant and which know me not, I am frightened and am astonished at being here rather than there; for there is no reason why here rather than there, why now rather than then.” [2]
Given the inexorable role of contingency in the origins of Earth-originating intelligence and civilization, we might observe once again that, from the perspective of the natural history of civilization, it makes little difference whether the extraterrestrialization of humanity as a spacefaring species occurs at our present stage of development, or ten thousand years from now, or a hundred thousand years from now. [3] When civilization eventually does, however, follow the example of the life that preceded it, and integrates itself into the cosmological context from which it descended, it will be subject to a whole new order of contingencies and selection pressures that will shape spacefaring civilization on an order of magnitude beyond terrestrial civilization.
The transition to spacefaring civilization is no more inevitable than the emergence of intelligence capable of producing civilization, or indeed the emergence of life itself. If it should come to pass that terrestrial civilization transcends its terrestrial origins, it will be because that civilization seizes upon an imperative informing the entire trajectory of civilization that is integral with a vision of life in the cosmos – an astrobiological vision, as it were. We cannot know the precise form such a civilizational imperative might take. In an earlier Centauri Dreams post, I discussed cosmic loneliness as a motivation to reach out to the stars. This suggests a Weltanschauung of human longing and an awareness of ourselves as being isolated in the cosmos. But if we were to receive a SETI signal next week revealing to us a galactic civilization, our motivation to reach out to the stars may well take on a different form having nothing to do with our cosmic isolation.
At the first 100YSS symposium in 2011, I spoke on “The Moral Imperative of Human Spaceflight.” I realize now I might have made a distinction between moral imperatives and civilizational imperatives: a moral imperative might or might not be recognized by a civilization, and a civilizational imperative might be moral, amoral, or immoral. What are the imperatives of a civilization? By what great projects does a civilization stand or fall, and leave its legacy? With what trajectory of history does a civilization identify itself and, through its agency, become an integral part of this history? And, specifically in regard to the extraterrestrialization of humanity and a spacefaring civilization, what kind of a civilization would not only recognize this imperative, but would center itself on and integrate itself with an interstellar imperative?
Also at the first 100YSS symposium in 2011 there was repeated discussion of multi-generational projects and the comparison of interstellar flight to the building of cathedrals. [4] In this analogy, it should be noted that cathedrals were among the chief multi-generational projects and are counted as one of the central symbols of the European middle ages, a central period of agrarian-ecclesiastical civilization. For the analogy to be accurate, the building of starships would need to be among the central symbols of an age of interstellar flight, taking place in a central period of mature industrial-technological civilization capable of producing starships.
To take a particular example, the Duomo of Florence was begun in 1296 and completed structurally in 1436—after 140 years of building. When the structure was started, there was no known way to build the planned dome. (To construct the dome in the conventional manner would have required more timber than was available at that time.) The project went forward nevertheless. This would be roughly equivalent to building the shell of a starship and holding a competition for building the propulsion system 122 years after the project started. The dome itself required 16 years to construct according to Brunelleschi’s innovative double-shelled design of interlocking brickwork, which did not require scaffolding, the design being able to hold up its own weight as it progressed. [5]
It is astonishing that our ancestors, whose lives were so much shorter than ours, were able to undertake such a grand project with confidence, but this was possible given the civilizational context of the undertaking. The central imperative for agrarian-ecclesiastical civilization was to preserve unchanged a social order believed to reflect on Earth the eternal order of the cosmos, and, to this end, to erect monuments symbolic of this eternal order and to suppress any revolutionary change that would threaten this order. (A presumptively eternal order vulnerable to temporal mutability betrays its mundane origin.) And yet, in its maturity, agrarian-ecclesiastical civilization was shaken by a series of transformative revolutions—scientific, political, and industrial—that utterly swept aside the order agrarian-ecclesiastical civilization sought to preserve at any cost. [6]
The central imperative of industrial-technological civilization is the propagation of the STEM cycle, the feedback loop of science producing technologies engineered into industries that produce better instruments for science, which then in turn further scientific discovery (which, almost as an afterthought, produces economic growth and rising standards of living). [7] One cannot help but wonder if this central imperative of industrial-technological civilization will ultimately go the way agrarian-ecclesiastical civilization, fostering the very conditions it seeks to hold in abeyance. In any case, it is likely that this imperative of industrial-technological civilization is even less understood than the elites of agrarian-ecclesiastical civilization understood the imperative to suppress change in order to retain unchanged its relation to the eternal.
It may yet come about that the building of an interstellar civilization becomes a central multi-generational project of industrial-technological civilization, as it comes into its full maturity, as a project of this magnitude and ambition would be required in order to sustain the STEM cycle over long-term historical time (what Fernand Braudel called la longue durée). We must recall that industrial-technological civilization is very young—only about two hundred years old—so that it is still far short of maturity, and the greater part of its development is still to come. Agrarian-ecclesiastical civilization was in no position to raise majestic gothic cathedrals for its first several hundred years of existence. Indeed, the early middle ages, a time of great instability, are notable for their lack of surviving architectural monuments. The Florence Duomo was not started until this civilization was several hundred years old, having attained a kind of maturity.
The STEM cycle draws its inspiration from the great scientific, technical, and engineering challenges of industrial-technological civilization. A short list of the great engineering problems of our time might include hypersonic flight, nuclear fusion, and machine consciousness. [8] The recent crash of Virgin Galactic’s SpaceShipTwo demonstrates the ongoing difficulty of mastering hypersonic atmospheric flight. We can say that we have mastered supersonic atmospheric flights, as military jets fly every day and only rarely crash, and we can dependably reach escape velocity with chemical rockets, but building a true spacecraft (both HOTOL and SSTO) requires mastering hypersonic atmospheric flight, as well as making the transition to exoatmospheric flight) and this continues to be a demanding engineering challenge.
Similarly, nuclear fusion power generation has proved to be a more difficult challenge than initially anticipated. I recently wrote in One Hundred Years of Fusion that by the time we have mastered nuclear fusion as a power source, we will have been working on fusion for a hundred years at least, making fusion power perhaps the first truly multi-generational engineering challenge of industrial-technological civilization—our very own Florentine Duomo, as it were.
With machine consciousness, if we are honest we will admit that we do not even know where to begin. AI is the more tractable problem, and the challenge that attracts research interest and investment dollars, and increasingly sophisticated AI is likely to be an integral feature of industrial-technological civilization, regardless of our ability to produce artificial consciousness.
Once these demanding engineering problems are resolved, industrial-technological civilization will need to look further afield in its maturity for multi-generational projects that can continue to stimulate the STEM cycle and thus maintain that civilization in a vital, vigorous, and robust form. A starship would be the ultimate scientific instrument produced by technological civilization, constituting both a demanding engineering challenge to build and offering the possibility of greatly expanding the scope of scientific knowledge by studying up close the stars and worlds of our universe, as well as any life and civilization these worlds may comprise. This next great challenge of our civilization, being a challenge so demanding and ambitious that it could come to be the central motif of industrial-technological civilization in its maturity, could be called the interstellar imperative, and we ourselves may be the Axial Age of industrial-technological civilization that first brings this vision into focus.
Even if the closest stars prove to be within human reach in the foreseeable future, the universe is very large, and there will be always further goals for our ambition, as we seek to explore the Milky Way, to reach other galaxies, and to explore our own Laniakea supercluster. This would constitute a civilizational imperative that could not be soon exhausted, and the civilization that sets itself this imperative as its central organizing principle would not lack for inspiration. It is the task of Project Astrolabe at Icarus Interstellar to study just these large-scale concerns of civilization, and we invite interested researchers to join us in this undertaking.
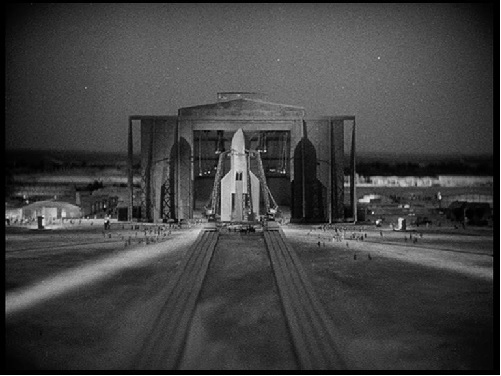
Image: Frau im Mond, Fritz Lang, 1929.
—
Jacob Shively, Heath Rezabek, and Michel Lamontagne read an earlier version of this essay and their comments helped me to improve it.
—
Notes
[1] I have specified intelligence emergent from a brain that supervenes upon a limbic system because in this context I am concerned with peer intelligence. This is not to deny that other forms of intelligence and consciousness are possible; not only are they possible, they are almost certainly exemplified by other species with whom we share our planet, but whatever intelligence or consciousness they possess is recognizable as such to us only with difficulty. Our immediate rapport with other mammals is sign of our shared brain architecture.
[2] No. 205 in the Brunschvicg ed., and Pascal again: “I see those frightful spaces of the universe which surround me, and I find myself tied to one corner of this vast expanse, without knowing why I am put in this place rather than in another, nor why the short time which is given me to live is assigned to me at this point rather than at another of the whole eternity which was before me or which shall come after me. I see nothing but infinites on all sides, which surround me as an atom and as a shadow which endures only for an instant and returns no more.” (No. 194)
[3] Or our extraterrestrialization might have happened much earlier in human civilization, as Carl Sagan imagined in his Cosmos: “What if the scientific tradition of the ancient Ionian Greeks had survived and flourished? …what if that light that dawned in the eastern Mediterranean 2,500 years ago had not flickered out? … What if science and the experimental method and the dignity of crafts and mechanical arts had been vigorously pursued 2,000 years before the Industrial Revolution? If the Ionian spirit had won, I think we—a different ‘we,’ of course—might by now be venturing to the stars. Our first survey ships to Alpha Centauri and Barnard’s Star, Sirius and Tau Ceti would have returned long ago. Great fleets of interstellar transports would be under construction in Earth orbit—unmanned survey ships, liners for immigrants, immense trading ships to plow the seas of space.” (Cosmos, Chapter VIII, “Travels in Space and Time”)
[4] While there are few examples of truly difficult engineering problems as we understand them today (i.e., in scientific terms) prior to the advent of industrial-technological civilization, because, prior to this, technical problems were only rarely studied with a pragmatic eye to their engineering solution, agrarian-ecclesiastical civilization had its multi-generational intellectual challenges parallel to the multi-generational scientific challenges of industrial-technological civilization — these are all the familiar problems of theological minutiae, such as the nature of grace, the proper way to salvation, and subtle distinctions within eschatology. And all of these problems are essentially insoluble for different reasons than a particularly difficult scientific problem may appear intractable. Scientific problems, unlike the intractable theological conflicts intrinsic to agrarian-ecclesiastical civilization, can be resolved by empirical research. This is one qualitative difference between these two forms of civilization. Moreover, it is usually the case that the resolution of a difficult scientific problem opens up new horizons of research and sets new (soluble) problems before us. The point here is that the monumental architecture of agrarian-ecclesiastical civilization that remains as its legacy (like the Duomo of Florence, used as an illustration here) was essentially epiphenomenal to the central project of that civilization.
[5] An entertaining account of the building of the dome of the Florence Duomo is to be found in the book The Feud That Sparked the Renaissance: How Brunelleschi and Ghiberti Changed the Art World by Paul Robert Walker.
[6] On the agrarian-ecclesiastical imperative: It is at least possible that the order and stability cultivated by agrarian-ecclesiastical civilization was a necessary condition for the gradual accumulation of knowledge and technology that would ultimately tip the balance of civilization in a new direction.
[7] While the use of the phrase “STEM cycle” is my own coinage (you can follow the links I have provided to read my expositions of it), the idea of our civilization involving an escalating feedback loop is sufficiently familiar to be the source of divergent opinion. For a very different point of view, Nassim Nicholas Taleb argues strongly (though, I would say, misguidedly) against the STEM cycle in his Antifragile: Things That Gain from Disorder (cf. especially Chap. 13, “Lecturing Birds on How to Fly,” in the section, “THE SOVIET-HARVARD DEPARTMENT OF ORNITHOLOGY”); needless to say, he does not use the phrase “STEM cycle.”
[8] This list of challenging technologies should not be taken to be exhaustive. I might also have cited high temperature superconductors, low energy nuclear reactions, regenerative medicine, quantum computing, or any number of other difficult technologies. Moreover, many of these technologies are mutually implicated. The development of high temperature superconductors would transform every aspect of the STEM cycle, as producing strong magnetic fields would become much less expensive and therefore much more widely available. Inexpensive superconducting electromagnets would immediately affect all technologies employing magnetic fields, so that particle accelerators and magnetic confinement fusion would directly benefit, among many other enterprises.
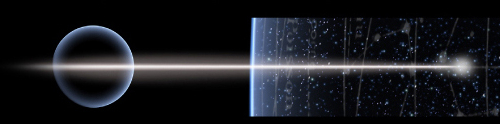
by Paul Gilster | Dec 4, 2014 | Astrobiology and SETI |
Yesterday’s post about planets in red dwarf systems examined the idea that the slow formation rate of these small stars would have a huge impact on planets that are today in their habitable zone. We can come up with mechanisms that might keep a tidally locked planet habitable, but what do we do about the severe effects of water loss and runaway greenhouse events? Keeping such factors in mind plays into how we choose targets — very carefully — for future space telescope missions that will look for exoplanets and study their atmospheres.
But the question of atmospheres on early worlds extends far beyond what happens on M-dwarf planets. At MIT, Hilke Schlichting has been working on what happened to our own Earth’s atmosphere, which was evidently obliterated at least twice since the planet’s formation four billion years ago. In an attempt to find out how such events could occur, Schlichting and colleagues at Caltech and Hebrew University have been modeling the effects of impactors that would have struck the Earth in the same era that the Moon was formed, concluding that it was the effect of small planetesimals rather than any giant impactor that would have destroyed the early atmosphere.
“For sure, we did have all these smaller impactors back then,” Schlichting says. “One small impact cannot get rid of most of the atmosphere, but collectively, they’re much more efficient than giant impacts, and could easily eject all the Earth’s atmosphere.”
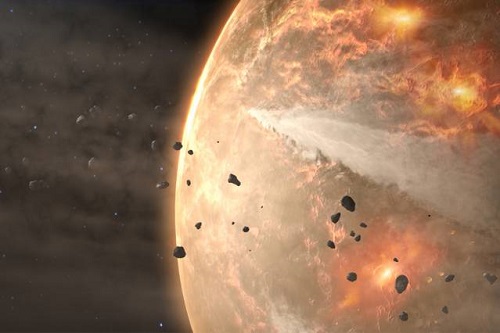
Image: An early Earth under bombardment. Credit: NASA.
A single large impact could indeed have dispersed most of the atmosphere, but as the new paper in Icarus shows, impactors of 25 kilometers or less would have had the same effect with a great deal less mass. While a single such impactor would eject atmospheric gas on a line perpendicular to the impactor’s trajectory, its effect would be small. Completely ejecting the atmosphere would call for tens of thousands of small impacts, a description that fits the era 4.5 billion years ago. Calculating atmospheric loss over the range of impactor sizes, the team found that the most efficient impactors are small planetesimals of about 2 kilometers in radius.
Schlichting believes that giant impacts cannot explain the loss of atmosphere, for recent work uses the existence of noble gases like helium-3 inside today’s Earth to argue against the formation of a magma ocean, the consequence of any such giant impact. From the paper:
Recent work suggests that the Earth went through at least two separate periods during which its atmosphere was lost and that later giant impacts did not generate a global magma ocean (Tucker & Mukhopadhyay 2014). Such a scenario is challenging to explain if atmospheric mass loss was a byproduct of giant impacts, because a combination of large impactor masses and large impact velocities is needed to achieve complete atmospheric loss… Furthermore, giant impacts that could accomplish complete atmospheric loss, almost certainly will generate a global magma ocean. Since atmospheric mass loss due to small planetesimal impacts will proceed without generating a global magma ocean they offer a solution to this conundrum.
The same impactors that drove atmospheric loss would, in this scenario, introduce new volatiles as planetesimals melted after impact. By the researchers’ calculations, a significant part of the atmosphere may have been replenished by these tens of thousands of small impactors. What happens to a newly formed planet that can lead to the emergence of life? Learning about the primordial atmosphere shows us a planet at a stage when life was about to take hold, which is why Schlichting’s team wants to move forward with an analysis of the geophysical processes that, in conjunction with small impactors, so shaped Earth’s early environment.
The paper is Schlichting et al., “Atmospheric Mass Loss During Planet Formation: The
Importance of Planetesimal Impacts,” Icarus Vol. 247 (February 2015), pp. 81-94 (abstract / preprint).
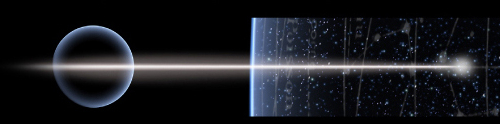
by Paul Gilster | Dec 3, 2014 | Exoplanetary Science |
A common trope from Hollywood’s earlier decades was the team of explorers, or perhaps soldiers, lost in the desert and running out of water. On the horizon appears an oasis surrounded by verdant green, but it turns out to be a mirage. At the University of Washington, graduate student Rodrigo Luger and co-author Rory Barnes have deployed the word ‘mirage’ to describe planets that, from afar, look promisingly like a home for our kind of life. But the reality is that while oxygen may be present in their atmospheres, they’re actually dry worlds that have undergone a runaway greenhouse state.
This is a startling addition to our thinking about planets around red dwarf stars, where the concerns have largely revolved around tidal lock — one side of the planet always facing the star — and flare activity. Now we have to worry about another issue, for Luger and Barnes argue in a paper soon to be published in Astrobiology that planets that form in the habitable zone of such stars, close in to the star, may experience extremely high surface temperatures early on, causing their oceans to boil and their atmospheres to become steam.
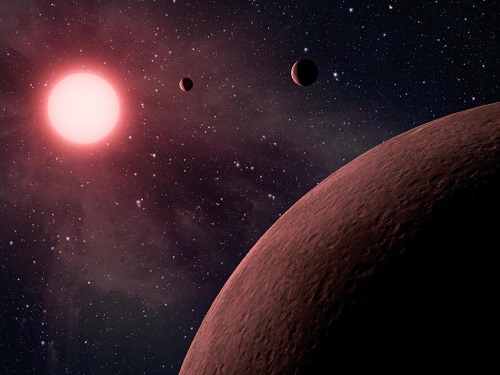
Image: Illustration of a low-mass, M dwarf star, seen from an orbiting rocky planet. Credit: NASA/JPL.
The problem is that M dwarfs can take up to a billion years to settle firmly onto the main sequence — because of their lower mass and lower gravity, they take hundreds of millions of years longer than larger stars to complete their collapse. During this period, a time when planets may have formed within the first 10 to 100 million years, the parent star would be hot and bright enough to heat the upper planetary atmosphere to thousands of degrees. Ultraviolet radiation can split water into hydrogen and oxygen atoms, with the hydrogen escaping into space.
Left behind is a dense oxygen envelope as much as ten times denser than the atmosphere of Venus. The paper cites recent work by Keiko Hamano (University of Tokyo) and colleagues that makes the case for two entirely different classes of terrestrial planets. Type I worlds are those that undergo only a short-lived greenhouse effect during their formation period. Type II, on the other hand, comprises those worlds that form inside a critical distance from the star. These can stay in a runaway greenhouse state for as long as 100 million years, and unlike Type I, which retains most of its water, Type II produces a desiccated surface inimical to life.
Rodrigo and Barnes, extending Hamano’s work and drawing on Barnes’ previous studies of early greenhouse effects on planets orbiting white and brown dwarfs as well as exomoons, consider this loss of water and build-up of atmospheric oxygen a major factor in assessing possible habitability. From the paper:
During a runaway greenhouse, photolysis of water vapor in the stratosphere followed by the hydrodynamic escape of the upper atmosphere can lead to the rapid loss of a planet’s surface water. Because hydrogen escapes preferentially over oxygen, large quantities of O2 also build up. We have shown that planets currently in the HZs of M dwarfs may have lost up to several tens of terrestrial oceans (TO) of water during the early runaway phase, accumulating O2 at a constant rate that is set by diffusion: about 5 bars/Myr for Earth-mass planets and 25 bars/Myr for super-Earths. At the end of the runaway phase, this leads to the buildup of hundreds to thousands of bars of O2, which may or may not remain in the atmosphere.
Thus the danger in using oxygen as a biosignature: In cases like these, it will prove unreliable, and the planet uninhabitable despite elevated levels of oxygen. The key point is that the slow evolution of M dwarfs means that their habitable zones begin much further out than they will eventually become as the star continues to collapse into maturity. Planets that are currently in the habitable zone of these stars would have been well inside the HZ when they formed. An extended runaway greenhouse could play havoc with the planet’s chances of developing life.
Even on planets with highly efficient surface sinks for oxygen, the bone-dry conditions mean that the water needed to propel a carbonate-silicate cycle like the one that removes carbon dioxide from the Earth’s atmosphere would not be present, allowing the eventual build-up of a dense CO2 atmosphere, one that later bombardment by water-bearing comets or asteroids would be hard pressed to overcome. In both cases habitability is compromised.
So a planet now in the habitable zone of a red dwarf may have followed a completely different course of atmospheric evolution than the Earth. Such a world could retain enough oxygen to be detectable by future space missions, causing us to view oxygen as a biosignature with caution. Says Luger: “Because of the oxygen they build up, they could look a lot like Earth from afar — but if you look more closely you’ll find that they’re really a mirage; there’s just no water there.”
The paper is Luger and Barnes, “Extreme Water Loss and Abiotic O2 Buildup On Planets Throughout the Habitable Zones of M Dwarfs,” accepted at Astrobiology (preprint). The paper by Hamano et al. is “Emergence of two types of terrestrial planet on solidification of magma ocean,” Nature 497 (30 May 2013), pp. 607-610 (abstract). A University of Washington news release is also available.
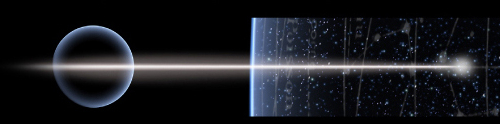