by Paul Gilster | Feb 5, 2016 | Outer Solar System |
If there’s one thing Pluto turned out to have beyond all expectation, it’s geological activity. New Horizons is now showing us what researchers are calling ‘hills of water ice’ floating in a sea of frozen nitrogen, much like icebergs moving through our own Arctic Ocean. The isolated hills are thought to be fragments of the water ice in the surrounding upland regions. Measuring several kilometers across, they are found in Sputnik Planum, a plain within Pluto’s ‘heart.’
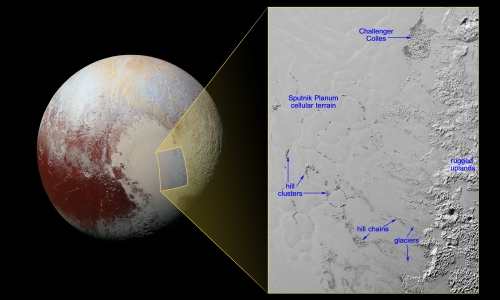
Image: The image shows the inset in context next to a larger view that covers most of Pluto’s encounter hemisphere. The inset was obtained by New Horizons’ Multispectral Visible Imaging Camera (MVIC) instrument. North is up; illumination is from the top-left of the image. The image resolution is about 320 meters per pixel. The image measures a little over 500 kilometers long and about 340 kilometers wide. It was obtained at a range of approximately 16,000 kilometers from Pluto, about 12 minutes before New Horizons’ closest approach on July 14, 2015. Credit: NASA/Johns Hopkins University Applied Physics Laboratory/Southwest Research Institute.
The hills, according to this JHU/APL news release, are probably upland fragments that are being carried by nitrogen glaciers into the ice plain. When they reach the central cellular terrain of Sputnik Planum, convective motion of the nitrogen ice pushes them to the edges of the cells, where they can be found in groups. Look toward the top of the image for Challenger Colles, where a large number of hills are found in an area roughly 60 by 35 kilometers. Near the boundary with the uplands and away from Sputnik Planum’s cellular terrain, this may be a region where the hills have run aground thanks to the shallowness of the nitrogen ice.
Learning about Pluto’s terrain as New Horizons data continues to stream in, I’m remembering how we used to imagine it. Digging around recently in my collection of old magazines, I uncovered the June, 1935 issue of Astounding Stories, then in the hands of editor F. Orlin Tremaine (this was two years before John Campbell took over). A story by Raymond Z. Gallun caught my eye because of its title: “Blue Haze on Pluto.” You’ll recall that last Monday we took another look at the real blue haze on Pluto as seen by New Horizons.
In Gallun’s story, a stranded astronaut has crashed into a deep crevasse and is forced to trek to a settlement on Pluto, learning as he does about a strange form of life that, in the Plutonian night, “…glimmered like serried hosts of huge gems in whose hearts icy fire of every hue throbbed and cascaded.” All of this in a landscape of “steep crags, part ice, part frozen atmosphere” that could stand in pretty well for parts of Pluto we see today. It’s not a bad tale for its day (our hero is, of course, rescued, with the ‘blue haze’ playing an inadvertent role), but for me its value is in that interesting interplay between the distant thing imagined and the distant thing observed, that generative place where our preconceptions are transformed by the incoming flow of data.
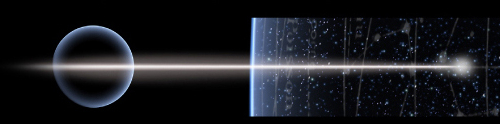
by Paul Gilster | Feb 4, 2016 | Outer Solar System |
Given that we have four planets in our Solar System with rings, it’s a natural thought that if so-called Planet Nine does exist, it might likewise show a system of rings. After all, Caltech’s Konstantin Batygin and Mike Brown are talking about a planet with a mass on the order of ten times that of the Earth. Neptune is about 17 Earth masses, while Uranus is 14.5 as massive. If Planet Nine is an ejected ice giant, perhaps it joins Uranus, Neptune, Jupiter and Saturn in having a ring system of its own, along with a thick atmosphere of hydrogen and helium.
Of course, we have to discover Planet Nine first, a process that may take some time if, indeed, it is successful. Meanwhile, we have interesting developments in the Solar System’s most intriguing ring system. As compared with those of other planets, Saturn’s rings are visually stunning. The B ring is the brightest and most opaque of the planet’s rings, but now we’re finding out that brightness and opacity have little correlation with how dense a ring may actually be. In fact, more opaque areas in the rings do not necessarily contain more material.
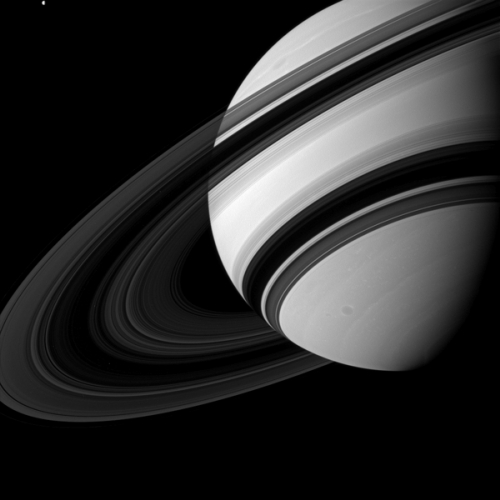
Image: Even Saturn’s rings appear to dwarf Tethys (1,062 kilometers across), which is in the upper left of the image, although scientists believe the moon to be many times more massive than the entire ring system combined. This view looks toward the unilluminated side of the rings from about 18 degrees below the ringplane. The image was taken in green light with the Cassini spacecraft wide-angle camera on Aug. 19, 2012. The view was acquired at a distance of approximately 2.4 million kilometers from Saturn and at a Sun-Saturn-spacecraft, or phase, angle of 63 degrees. Image scale is 138 kilometers per pixel. Credit: NASA/JPL-Caltech/Space Science Institute.
This is work using Cassini data that goes about the complicated process of ‘weighing’ the opaque center of the B ring, which involves analyzing spiral density waves within the structure. These fine-scale ring features are pulled into existence by Saturn’s gravity and that of individual moons tugging on ring particles, their structure reflecting the amount of mass in given parts of the rings. While different levels of opacity are obvious, the results on mass are puzzling.
Matthew Hedman (University of Idaho), who is lead author on the work and a participating scientist in the Cassini mission that collected the needed data, frames the problem this way:
“At present it’s far from clear how regions with the same amount of material can have such different opacities. It could be something associated with the size or density of individual particles, or it could have something to do with the structure of the rings.”
So we have a prominent B ring whose opacity varies markedly across its width, while the amount of material in it does not seem to vary greatly from one part of the ring to another. Cassini co-investigator Phil Nicholson (Cornell University) notes that appearances aren’t always what we think. “A good analogy is how a foggy meadow is much more opaque than a swimming pool,” says Nicholson, “even though the pool is denser and contains a lot more water.”
Just what it is about Saturn’s rings that sets them so far apart from the other rings in our Solar System is an open question. As this JPL news release points out, an earlier study by Cassini’s composite infrared spectrometer team has suggested that there was less material in the B ring than researchers once thought. This new work measures the density of mass in the ring for the first time to corroborate the idea. The researchers were able to use data from Cassini’s visible and infrared mapping spectrometer as the craft looked through the rings toward a bright star. The method helped to identify spiral density waves that were otherwise not apparent.
From the paper in press:
… this new estimate of the ring’s total mass is based on just a few locations in the B ring, and so one could argue that most of the B-ring’s mass is hidden in the truly opaque parts of the rings. However, the measurements considered here include regions with optical depths between 3.5 and 4.5, so these massive regions would have to be those with opacities above 4 or 5. Note that only about 27% of the ring’s surface area has an optical depth greater than 4, and less than 16% has an optical depth above 5, so there is not much space to hide a large amount of mass.
Understanding how the rings are constituted is a step toward understanding their age and origin. While the B ring is thought to contain the bulk of material in the ring system, it’s still surprising that the B ring may have only two to three times the mass of the A ring. Parts of the B ring are, after all, up to ten times more opaque than the A ring. We can look to one of Cassini’s last acts, in 2017, to help extend our data. The spacecraft, enroute to an ultimate descent into Saturn’s clouds, will fly just inside the rings, offering measurements that will help us separate the mass of the rings in the aggregate from the previously measured combined mass of planet and rings.
The paper is Hedman and Nicholson, “The B-ring’s surface mass density from hidden density waves: Less than meets the eye?” in press at Icarus and available online 22 January 2016 (abstract).
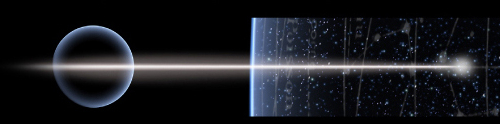
by Paul Gilster | Feb 3, 2016 | Deep Sky Astronomy & Telescopes |
Somewhere a decade or so back in these pages a Centauri Dreams commenter described the event that formed our Moon as ‘the big whack.’ Although I hadn’t run across it before, the phrase turns out to have been common parlance for what is now thought to be a massive collision between the Earth and an early planetesimal. But whatever the case, we know a bit more about the cataclysm thanks to new work out of UCLA, as reported in the journal Science.
The impactor, which struck about 4.5 billion years ago, is commonly called Theia. So how do we analyze such a remote event? The key, as discussed in this UCLA news release, is oxygen, which makes up 90 percent of the volume of lunar rocks the team of geochemists studied, and 50 percent of their weight. Usefully, oxygen can manifest itself in various isotopes, the most common on Earth being O-16, meaning each atom holds eight protons and eight neutrons.
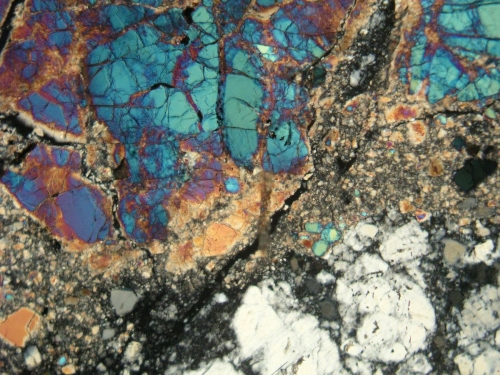
Image: Light image of a lunar rock from the Apollo 17 mission. Credit: NASA.
Heavier isotopes like O-17 (with one extra neutron) and O-18 (with two) occur, though on Earth 99.9 percent of the oxygen is O-16. But each of the planetary bodies in our Solar System has a unique ratio of O-17 to O-18, making for a characteristic signature.
To analyze these ratios, the team studied seven moon rocks from Apollo missions 12, 15 and 17, while also working with five volcanic rocks from Hawaii and one from Arizona. Led by UCLA’s Edward Young, the team found no difference between the Earth’s oxygen isotopes and those of the Moon. This contradicts a 2014 study from Germany (also published in Science) that argued for a distinct ratio of oxygen isotopes on the Moon as opposed to the Earth.
Theia was probably a growing object that would have become a planet in its own right. It may have been as large as the Earth, though some believe it was closer in size to Mars. If the UCLA work is correct, the oxygen isotope data tell us that the collision would have been head-on, for if it had been a glancing blow, the great bulk of the Moon would have been made up of Theia, and would thus have shown different oxygen isotope ratios than the Earth’s. A head-on collision should have produced a similar chemical composition on both Earth and Moon.
“Theia was thoroughly mixed into both the Earth and the moon, and evenly dispersed between them,” Young said. “This explains why we don’t see a different signature of Theia in the moon versus the Earth.”
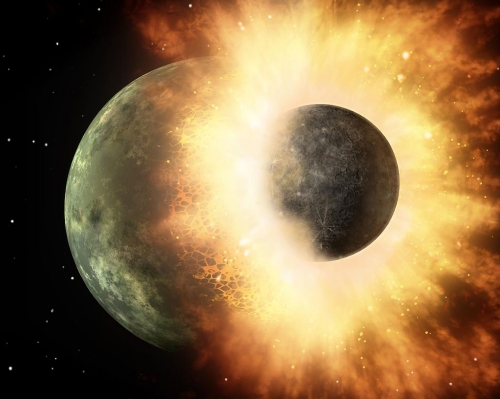
Image: Artist’s depiction of a collision between two planetary bodies. Such an impact between the Earth and a Mars-sized object likely formed the Moon. Credit: NASA/JPL-Caltech.
Thus a head-on collision, as theorized in 2012 by Matija ?uk (SETI Institute) and Sarah Stewart (UC-Davis), and also by Robin Canup (SwRI) in the same year, now has more weight. The catastrophic impact’s possible role in removing any water found in the early Earth is not known, though water from small asteroid and comet impacts would eventually be plentifully available.
The paper is Young et al., “Oxygen isotopic evidence for vigorous mixing during the Moon-forming giant impact,” Science Vol. 351, Issue 6272 (2016), pp. 493-496 (abstract). For the German work in 2014, see D. Herwartz, A. Pack, B. Friedrichs, A. Bischoff, Science 344, 1146–1150 (2014).
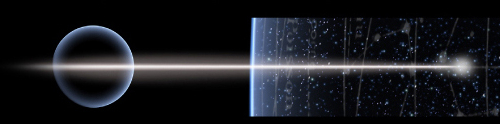
by Paul Gilster | Feb 2, 2016 | Deep Sky Astronomy & Telescopes |
If you’re looking for detailed imagery of a distant astronomical object, VLBI (Very Long Baseline Interferometry) can deliver the goods. As witness the image below, which the National Radio Astronomy Observatory (NRAO) is calling “the highest resolution astronomical image ever made.” Here we see radio emission from a jet of particles moving close to the speed of light. The particles are being accelerated by a supermassive black hole at the core of the galaxy BL Lacertae, a highly variable ‘active galaxy’ some 900 million light years from the Earth.
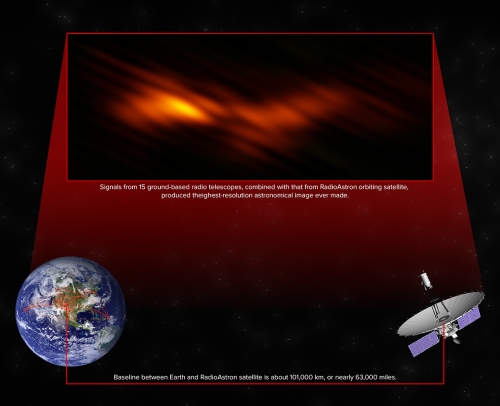
Image (click to enlarge): Signals from 15 ground-based radio telescopes, combined with data from the RadioAstron orbiting satellite, produced the highest resolution astronomical image ever made. Credit: Gomez, et al., Bill Saxton, NRAO/AUI/NSF.
What fascinates me about this work is the technique. Very Long Baseline Interferometry works by collecting a signal at multiple radio telescopes, the distance between them being calculated from the different arrival times of the radio signal at the different installations. The calculations are made using a local atomic clock, with the data correlated with data from other antennae in the array to produce an image.
The upshot is that VLBI methods allow us to work with an emulated ‘telescope’ that has a size equal to the maximum separation between the constituent telescopes in the array. Conventional interferometry does much the same thing, but VLBI does not demand the physical coaxial cable or optical fiber connections of the former, allowing for greater distances between the telescopes.
In this case, we have fifteen ground-based radio telescopes combining their data with signals from the Spektr-R satellite of the RadioAstron mission, a Russian spacecraft with a 10-meter radio telescope. Launched in July of 2011, Spektr-R can work with large Earth-based radio telescopes to form interferometric baselines that extend up to 350,000 kilometers. In this case, working with radio telescopes in Europe and nine antennae from the Very Long Baseline Array in the US, the image produced was one with the resolving power of a telescope 100,000 kilometers wide, roughly eight times the diameter of the Earth. From the paper:
RadioAstron provides the first true full-polarization capabilities for space VLBI observations on baselines longer than the Earth’s diameter, opening the possibility to achieve unprecedentedly high angular resolution in astronomical imaging. In this paper we present the first polarimetric space VLBI observations at 22 GHz, obtained as part of our RadioAstron KSP [Key Science Program] designed to probe the innermost regions of AGN and their magnetic fields in the vicinity of the central black hole.
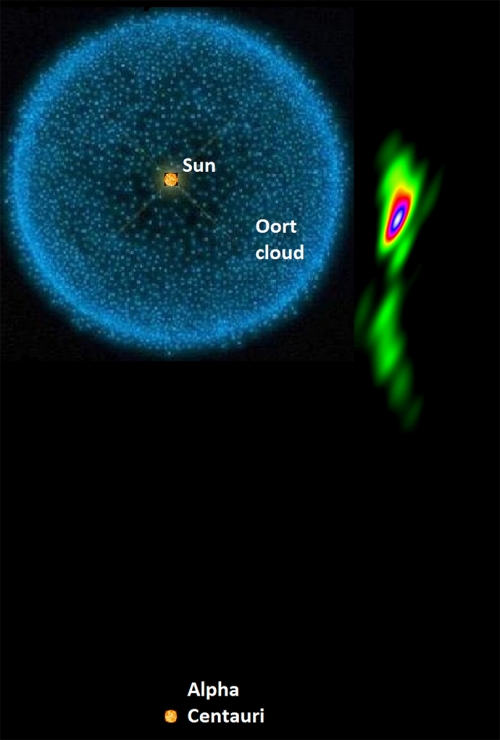
Credit: Artwork by Jon Lomberg.
Above we see the jet from BL Lacertae fitted within a diagram of our local interstellar neighborhood. Notice that if we take the Oort Cloud as the outer perimeter of our Solar System, the jet just about fits within the system or, if we imagine one end of the jet at the outer edge of the Oort, the jet would extend almost to the Alpha Centauri stars. The detail we can see here is roughly equivalent to seeing a 50-cent coin on the Moon. The image elongation results from the greater resolving power in the direction of the satellite as compared to the ground telescopes, with resolution in the orthogonal direction being exaggerated to compensate for the effect.
As for BL Lacertae itself, it was originally thought to be a variable star within the Milky Way after its discovery in 1929, but was later identified as a bright radio source. Subsequent redshift measurements showed a recession velocity of 21,000 kilometers per second, implying a distance of 900 million light years. BL Lacertae is considered an AGN, or active galactic nucleus galaxy, the latter being a designation for a galaxy with a compact region at its center that has a much higher than normal luminosity over at least part of the electromagnetic spectrum.
The paper is Gómez et al., “Probing the innermost regions of AGN jets and their magnetic fields with RadioAstron. I. Imaging BL Lacertae at 21 microarcsecond resolution,” Astrophysical Journal Vol. 817, No. 2 (26 January 2016). Preprint available.
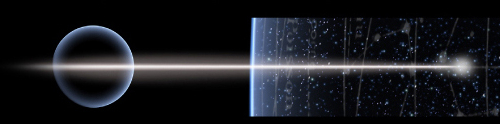
by Paul Gilster | Feb 1, 2016 | Outer Solar System |
Newly interpreted data from the New Horizons spacecraft tells us that Pluto has more water ice on its surface than we once thought. The image below tells the tale, a false-color view derived from observations by the Ralph/Linear Etalon Imaging Spectral Array (LEISA) instrument. Here we’re at infrared wavelengths and can see areas showing the spectral signature of water ice. Note the sharp contrast between the left and right sides of the image below.
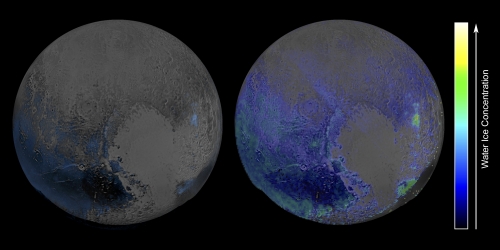
Image: This false-color image is derived from observations in infrared light by the Ralph/Linear Etalon Imaging Spectral Array (LEISA) instrument. It is based on two LEISA scans of Pluto obtained on July 14, 2015, from a range of about 108,000 kilometers. Credit: NASA/Johns Hopkins University Applied Physics Laboratory/Southwest Research Institute.
The two scans, as this JHU/APL news release explains, were taken about fifteen minutes apart and merged into a ‘data cube’ — a three-dimensional array covering the hemisphere New Horizons could see during the flyby last summer, where an image of Pluto is formed at each wavelength of light to which LEISA is sensitive. The map on the left was made by comparing LEISA spectra with a pure water ice template spectrum. When using this technique, methane ice can easily mask the spectral signature of water ice.
The result: A map sensitive only to those areas particularly rich in water or depleted in methane. On the right is an image that more fully models the various ices, showing the true extent of water ice. Interestingly, we still see little water ice in Sputnik Planum (left of Pluto’s ‘heart’) and Lowell Regio (far north on the encounter hemisphere). This would indicate that the ice ‘bedrock’ is buried beneath a thick blanket of ices like methane, nitrogen and carbon monoxide.
Infrared Views of Pluto’s Atmosphere
We’re also working with data from the LEISA instrument in the image below. Captured on July 14, 2015 at a distance of 180,000 kilometers, the image covers the full spectral range (1.25 to 2.5 microns) of the instrument. North in the image is at about the 10 o’clock position.
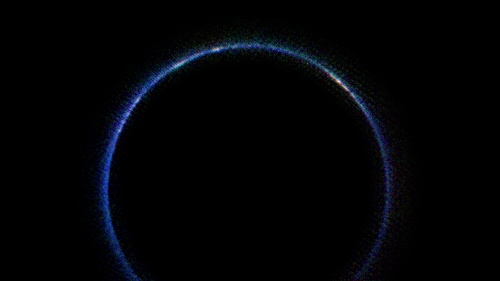
Image: This image from NASA’s New Horizons spacecraft is the first look at Pluto’s atmosphere in infrared wavelengths, and the first image of the atmosphere made with data from the New Horizons Ralph/Linear Etalon Imaging Spectral Array (LEISA) instrument. Credit: NASA/Johns Hopkins University Applied Physics Laboratory/Southwest Research Institute.
The blue ring we see in the image is the result of sunlight scattering off haze, as JHU/APL explains in this second news release:
…scientists believe the haze is a photochemical smog resulting from the action of sunlight on methane and other molecules, producing a complex mixture of hydrocarbons such as acetylene and ethylene. These hydrocarbons accumulate into small particles – a fraction of a micrometer in size – which scatter sunlight to make the blue haze.
Infrared images like these can be combined with images made at shorter wavelengths to help researchers understand the size distribution of the particles. The New Horizons team tells us that the white patches that show up around Pluto’s limb are where sunlight is bouncing off more reflective areas on Pluto’s surface. The largest of these would be the the area now being called Cthulhu Regio (the H.P. Lovecraft reference still delights me). We have much more data ahead from New Horizons, and can expect fuller views of the atmosphere in the course of the year.
A Change at the Top
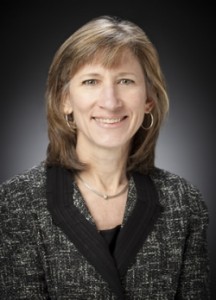
I also want to note that New Horizons has a new project manager. Helene Winters takes over the role from Glen Fountain, who had been project manager since early 2004. Winters served as project manager for the Mini-RF lunar radar instrument, and most recently as project manager for the MESSENGER mission, which orbited Mercury for four years before a planned dive into Mercury’s surface last April. Winters will also continue as MESSENGER project manager through that mission’s closeout stages. Says principal investigator Alan Stern:
“Over the past 15 years New Horizons has had two of the finest project managers in the space business, the late Tom Coughlin, who helped conceive the mission, and the legendary Glen Fountain, who built New Horizons and flew us to Pluto. Helene Winters, from a new generation and with incredible experience behind her, is going to set new milestones flying the spacecraft farther, hopefully to the first ever flyby of a Kuiper Belt object and onward toward the edge of our planetary system!”
The outer system, as we’ve learned in recent days, is getting more and more interesting. As we look forward to the New Horizons KBO flyby in January of 2019, we should also keep pondering the kind of follow-up mission that New Horizons merits. We need observations of the local interstellar medium beyond the heliosphere and we also have a wide range of Kuiper Belt targets, not to mention the eventual goal of reaching the Sun’s gravity focus at 550 AU. Tune up the technologies to reach these distances and, if it’s really out there, we might just visit the hypothesized Planet Nine.
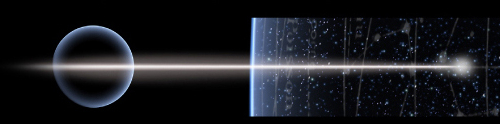