by Paul Gilster | Mar 8, 2016 | Astrobiology and SETI |
Running a site like Centauri Dreams means adapting and reconfiguring on a daily basis. The best laid plans and all that… When I wrote recently about the SETI efforts at KIC 8462852, my plan had been to follow up that discussion with a broader SETI issue — where is the best place in the sky to search for a SETI signal? Then life intervened, first with my preparations to go to the Tennessee Valley Interstellar Workshop in Chattanooga, and then with the illness that cancelled those plans and left me with a thoroughly disrupted train of thought.
I’m now ready to tackle that SETI question with particular reference to a new paper by René Heller and Ralph E. Pudritz, but I still want to put the discussion into context. With the KIC 8462852 SETI effort, we looked at a targeted observation campaign using the Allen Telescope Array to see if researchers could find any evidence of unusual activity associated with the star. As we saw in Jim and Dominic Benford’s recent work (see Power Beaming Parameters & SETI re KIC 8462852), no evidence of microwave beaming could be found in the brief observational window, although our equipment would be capable of detecting many forms of it.
The unusual light curves in our Kepler data made KIC 8462852 a high-profile target. What the ATA search was looking for was ‘leakage’ radiation, associated with the activities of a technological civilization but not intended as deliberate attempts to communicate with anyone else. And the fact that we found nothing shouldn’t be taken too far in any particular direction. A thorough study of KIC 8462852 in a more systematic manner and at other frequencies would be called for if we wanted to investigate the star in depth and had the resources to do it long-term.
But what about this matter of leakage’ radiation? It’s an intriguing fact that there have been receptions of signals of a one-off nature (the Wow! signal is one) that could conceivably be the result of a beam sweeping past us from a distant system. Or, at least, consistent with it — these are pulsed, intermittent signals that were reported, for example, in a 1997 survey of the Milky Way’s center (citation below). We also have sources like GCRT J1745-3009, a transient bursting radio source that fails our expectations for flare stars, binary pulsars and much else.
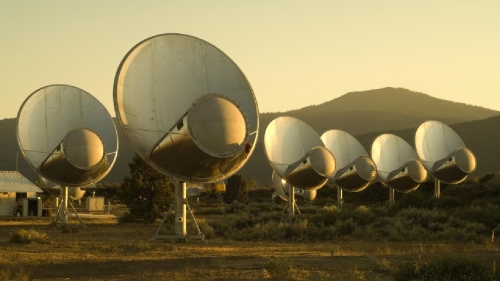
Image: The Allen Telescope Array, recently used for a SETI effort at KIC 8462852. Credit: ATA.
A Communicating Civilization
SETI got started in the experimental way with Frank Drake’s work at Green Bank in 1960, targeting the close stars Tau Ceti and Epsilon Eridani. Here the intention was to listen out for a directed signal, a ‘hello’ announcement from another star system, and for one brief, unforgettable moment, Drake thought he had found one (the signal, we now know, was local). Given the nature of such directed beaming, this would theoretically be a much easier signal to detect, as it would stay fixed upon us and would be at sufficient power levels that, unlike our own radio and television broadcasts, it would survive the long interstellar journey.
Most of the SETI searches since — there have been more than 100! — have looked at nearby systems or in some cases stellar clusters. The SETI Institute’s Project Phoenix worked at different sites between 1995 and 2004 and, according to Heller and Pudritz (both at McMaster University, Ontario) covered more than 800 stars as distant as 240 light years. We’ve done targeted searches of the galactic center and highly focused looks at specific stars like Gl 581, and in 2015 we looked for laser emissions from more than a thousand Kepler Objects of Interest. And let’s not forget the SETI@Home project that draws on Arecibo data.
We have yet to find directed beacons or leakage radiation unless some of the signals discussed above happen to be instances of one or the other — the eponymous ‘Benford beacon’ would actually sweep past us as a transient which we couldn’t identify without further observations.
In terms of sheer numbers, we would expect leakage signals would be the most abundant, because they would be generated by many technological civilizations and not just those intent on communicating with us. In any case, where to look seems clear. Looking toward regions of sky with higher stellar densities makes abundant sense. If ETI is out there, we would expect to see more signal activity where there are more worlds that might be habitable.
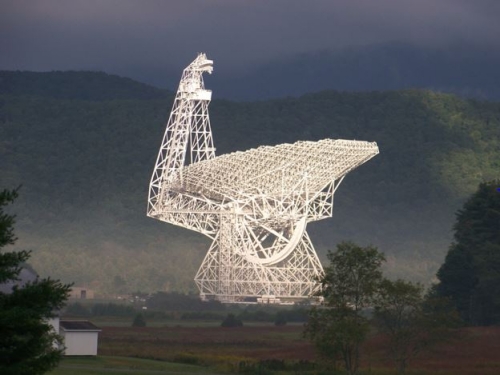
Image: The world’s largest fully steerable radio telescope, at Green Bank, West Virginia. Frank Drake launched observational SETI from Green Bank in 1960. Credit: NRAO.
Toward the Galactic Center
Thus the galactic center search strategy laid out by Gregory, Dominic and Jim Benford in an older paper, 2010’s “Searching for Cost Optimized Interstellar Beacons,” which calls for a search in the plane of the spiral disk because 90 percent of the galaxy’s stars are within 9% of the sky in the plane and hub of the galaxy. From the analysis:
Whatever forms might dwell further in from us toward the center, they must know the basic symmetry of the spiral. This suggests the natural corridor for communication is along the spiral’s radius from Galactic Center or toward it, a simple direction known to everyone. (A radius is better than aiming along a spiral arm, since the arm curves away from any straight-line view of view. On the other hand, along our nearby spiral arms the stars are roughly the age of ours.) This avenue maximizes the number of stars within a telescope’s view, especially by staring at the galactic hub. Thus, a Beacon near the center should at least broadcast outward in both directions, while societies at the far reaches may save half their cost by not emitting outward, since there is much less chance of advanced societies there.
But we’re not through yet. In fact, we’re just beginning, as this paper goes on to explain. In 2004, Robert A. Rohde & Richard A. Muller (UC-Berkeley) suggested a 62 million-year cycle followed by marine life on Earth, a suggestion developed by subsequent researchers to suggest that the movement of our Sun vertically above and below the galactic plane (a 62-million year oscillation) would mean that the galaxy’s bow shock produces additional cosmic ray flux when the Sun reaches its extended position north of the galactic plane. This enhanced flux could damage the biosphere and would presumably do so for any inhabited world.
We may, then, have a plane near the center of the galactic disk perhaps 500 light years deep within which intelligent life is more likely to be found. It’s interesting to note that the highest power transient sources reported by Carl Sagan and Paul Horowitz in a 1993 paper lie close to the galactic plane, and the idea of a vertical oscillation of about 500 light years within which intelligent life is more likely gives us another way to focus our search on likely targets.
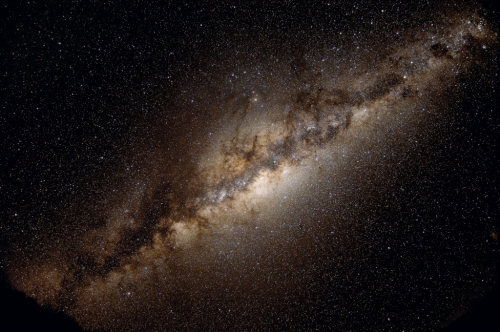
Image: The Milky Way in stars and dust, showing us the most likely regions of the sky in which to search for SETI signals. Credit & Copyright: Serge Brunier.
Now back to Heller and Pudritz, who have drawn on an idea first mooted in the 1980s. The two scientists suggest that we focus our attention to an even smaller swath of stars, as defined by planetary systems from which it is possible to see the Earth transiting in front of the Sun. Think of a thin strip around the ecliptic and project that strip onto the galaxy — Heller and Pudritz call this strip the Earth’s transit zone, or ETZ. The idea is that extraterrestrials observing the Earth from within the ETZ will have direct evidence that there is life here, which would make them more likely to attempt communications.
I’m running out of time this morning, but tomorrow we’ll look at the notion of the Earth’s transit zone as it applies to practical decisions about targets. It turns out there are numerous stars for us to look at, but it’s also a well-defined population of extremely high-priority targets.
The paper is Heller & Pudritz, “The Search for Extraterrestrial Intelligence in Earth’s Solar Transit Zone,” Astrobiology Vol. 16, No. 4 (2016) (preprint). The Benfords’ paper is “Searching for Cost Optimized Interstellar Beacons,” Astrobiology 10 (2010), 491-498 (abstract / preprint). The 1997 paper mentioning the transients I discuss above is Sullivan et al., “A Galactic Center Search For Extraterrestrial Intelligent Signals,” Astronomical and Biochemical Origins and the Search for Life in the Universe, IAU Colloquium 161, Publisher: Bologna, Italy, p. 653.
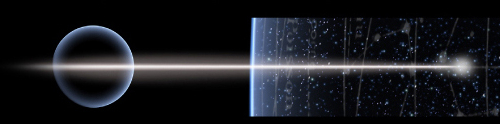
by Paul Gilster | Mar 7, 2016 | Deep Sky Astronomy & Telescopes |
You probably recall how tricky it was to find 2014 MU69, the small Kuiper Belt Object that will be the next destination for our New Horizons probe. The actual extended mission to 2014 MU69 awaits a summer 2016 review within NASA, but because trajectory changes to get there could not be delayed, the New Horizons craft performed four maneuvers late last year to set the course. The search for a suitable KBO began in 2011 and it was not until 2014, working with data from the Hubble Space Telescope, that scientists were able to find their best candidate.
Now consider that 2014 MU69 has a diameter of about 45 kilometers, making it ten times larger than the average comet. How difficult, then, to assess the true population of smaller Kuiper Belt Objects. We’d like to know a lot more about much smaller KBOs, because assessing the abundance and collisional processes of these objects is way of understanding the debris disks we’re seeing around other stars. Current theory suggests that KBOs below about 10 kilometers in radius are readily broken up, but we haven’t been able to test the idea with observations because objects this small are too faint to be in detected in reflected light.
Now we have an improved way of looking. CHIMERA (the Caltech HIgh-speed Multi-color camERA) works by studying occultations, monitoring thousands of stars simultaneously to see if a KBO of any size moves in front of them for a fraction of a second. All of this calls for a fast camera, but this wide-field telescope system seems up to the job. Leon Harding is CHIMERA instrument scientist at the Jet Propulsion Laboratory, and lead author of the paper on this work:
“Each of CHIMERA’s cameras will be taking 40 frames per second, allowing us to measure the distinct diffraction pattern in the wavelengths of light to which they are sensitive. This high-speed imaging technique will enable us to find new Kuiper Belt objects far less massive in size than any other ground-based survey to date.”
Scientists working with CHIMERA, which is now installed on the Hale telescope at Palomar, believe that the first 100 hours of data from the instrument should produce dozens of small KBOs. Usefully, the instrument is also effective for detecting near-Earth asteroids down to 10 meters in size. In fact, its wide-field imaging techniques can be applied to everything from nearby debris in space to distant eclipsing binary star systems, pulsing white dwarfs, aurorae on brown dwarfs and transiting planets. Adds Harding: “Not only can we image over a wide field, but in other modes we can also image objects rotating hundreds of times per second.”
As witness the image below, showing the Crab Pulsar as seen by CHIMERA. Spinning at 32 times per second, the pulsar is a young neutron star that is the remnant of the supernova observed on Earth in the year 1054, and is one of the few pulsars that can be observed optically. CHIMERA focused on the pulsar for a 300-second exposure, imaging the entire field of view at a rate of 40 frames per second. The result speaks for itself.
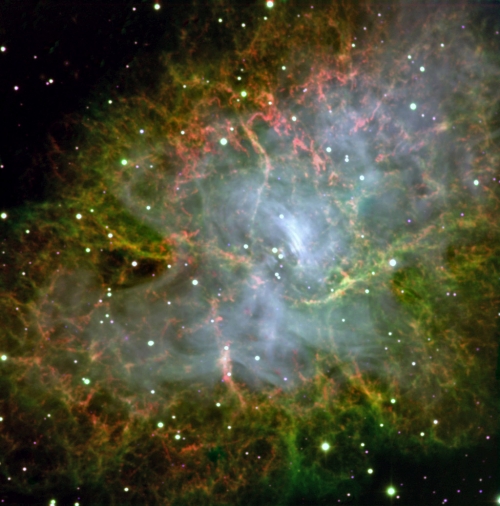
Image: This image of the Crab Pulsar was taken with CHIMERA, an instrument on the Hale telescope at the Palomar Observatory, which is operated by the California Institute of Technology. This pulsar is the end result of a star whose mass collapsed at the end of its life. It weighs as much as our sun, but spins 32 times per second. The instrument focused on the pulsar for a 300-second exposure to produce a color image. CHIMERA zoomed in on the pulsar and imaged it very fast, then imaged the rest of the scene slowly to create this image. Credit: NASA/JPL-Caltech.
But as the first paper on CHIMERA, published in Monthly Notices of the Royal Astronomical Society notes, the instrument was conceived and designed to search for KBOs, given the fact that 31,500 star hours of archival data from the Hubble Space Telescope produced so few discoveries. Ground-based surveys with the right equipment should ramp up those numbers.Commissioned in July of 2014, CHIMERA is now at work on Kuiper Belt detections. A substantial upgrade extending its capabilities is already in the works.
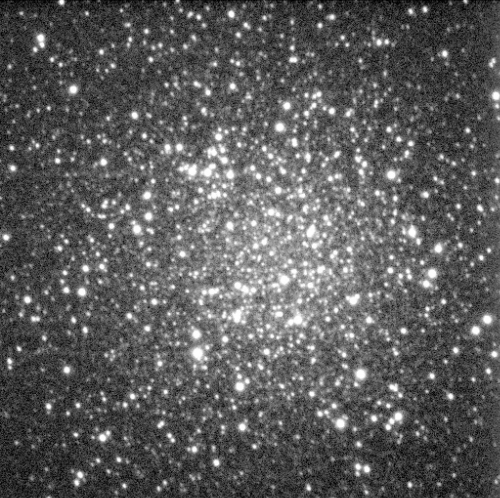
Image: The globular cluster M22, located in the constellation Sagittarius, was imaged by the CHIMERA instrument. The team will observe M22 and other star fields to look for signs of Kuiper Belt objects. Credit: NASA/JPL-Caltech.
The paper is Harding et al., “CHIMERA: a wide-field, multi-colour, high-speed photometer at the prime focus of the Hale telescope,” published online by Monthly Notices of the Royal Astronomical Society 2 March 2016 (full text). A JPL news release is also available.
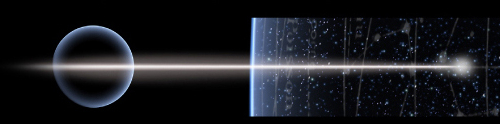
by Paul Gilster | Mar 4, 2016 | Exoplanetary Science |
David Kipping and colleagues have discovered what they describe as ‘the first validated transiting Jupiter analog,’ a planet orbiting the K-class dwarf KIC-3239945. Kepler-167e is about 90 percent the size of Jupiter and orbits its star at about twice the distance that the Earth orbits the Sun. Given the fact that the star is cooler than the Sun — an orange rather than a yellow dwarf — temperature estimates for the planet are in the 130 K range, only about 20 K warmer than Jupiter. The discovery is discussed on the Cool Worlds YouTube channel, an outreach project launched by the Cool Worlds Lab at Columbia University, and is the subject of a paper submitted to The Astrophysical Journal (citation below).
Kepler-167e isn’t just another ‘hot Jupiter’, a class of worlds that is well populated. ‘Hot Jupiters’ occupy orbits extremely close to the parent star. Finding a true Jupiter analog — i.e., a planet in a close to circular orbit in a position roughly analogous to Jupiter’s in our Solar System — is a considerably more difficult challenge because of the nature of our detection methods. Radial velocity techniques readily detect planets close to their stars but become far more challenging with increasing orbital distance. Transits, meanwhile, have proven their worth through the Kepler mission and elsewhere, but a planet as far from its star as Kepler-167e will show transits separated by years. That demands patient observation, and raw luck.
Consider: Kepler-167e transits its star every 2.9 years. Kipping points out that the primary Kepler mission only looked at this star for 4.3 years. We are lucky, in other words, that we got two transits, when conceivably we might have detected just a single transit right in the middle of the observation period. A single transit, of course, would yield no idea of the orbital period of the planet. But as Kipping goes on to say in the video, the transit method has additional virtues:
“The transit method can actually do a lot more than other methods. We can not only tell [the planet’s] size and its inclination, we can even go look for moons, for rings, we can even measure the atmosphere of exoplanets. And you just can’t do that with other techniques.”
True enough, and of course Kipping’s work at the Hunting for Exomoons with Kepler (HEK) project is all about exomoon detection. We’re now getting into interesting territory indeed, for we know that in our own Solar System, the gas giants Jupiter and Saturn are accompanied by numerous icy moons. If we begin finding more and more Jupiter analogs, we may be upping the stakes for the detection of exomoons, a hunt which has so far been conducted on worlds orbiting their stars closer than the Earth orbits the Sun. Kipping calls Jupiter analogs ‘a promised land for exomoon hunting.’ And let’s not forget the possibility of rings.
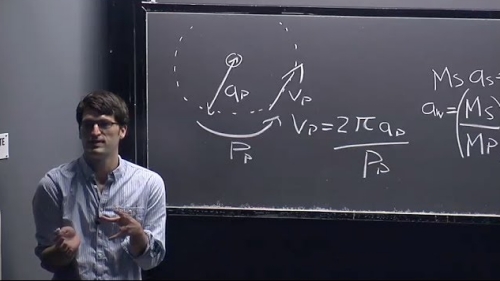
Image: David Kipping (Columbia University) delivering a guest lecture at Harvard on “Life as a Planetary Phenomenon.” Click to watch the lecture on YouTube.
Are two transits enough to declare this a planet candidate? I asked Kipping about this in an email, and this is his response:
Yes, historically the standard has been three (or more) transits. I suspect this stems from the fact early transit surveys were exclusively ground-based, which have plenty of data gaps. With just two transits and lots of gaps in between, you can’t be sure the difference in time between the two is the real period, it could a half or a third that value. For Kepler-167e though, we have continuous photometric coverage of the star (from Kepler) in the 1071 day gap between the two events, and so we can rule out the period being some integer fraction of 1071 days.
The paper on this work offers further insights. One issue at stake is the planetary architecture of the system around KIC-3239945. Inside the orbit of Kepler-167e we find three other planets, each of them about twice the size of Earth. In some sense, then, this system resembles ours except that these inner planets are close to their star. We see a compact and transiting inner system, then a large cavity and then a transiting Jupiter analog. The paper speculates that because transit surveys have poor sensitivity to long-period planets, it may be that case that outer planets like Kepler-167e will be found in many compact multi-planet systems.
The paper goes on to suggest that radial velocity surveys targeting the brighter multi-planet systems found by Kepler could eventually resolve this issue. And note this:
Additional non-transiting planets could reside in the Kepler-167 cavity, although the known four planets display remarkable coplanarity and low eccentricities, suggestive of a dynamically cold system. Amongst the inner planets, the planet sizes increase as one approaches the parent star. Using the Chen & Kipping (2016) mass-radius model, we estimate that the inner two planets are most likely gaseous worlds whilst the outer planet is most likely rocky… Whilst this pattern ostensibly jars our anthropocentric prior, as well as the expected outcome of photo-evaporation (Lopez & Fortney 2013), Ciardi et al. (2013) find that there is no preferential ordering of compact Kepler multis for planets R ? 3R?.
It’s always useful, in my estimation, to give a good jolt to our anthropocentric assumptions! Transiting Jupiter analogs may turn out to be a very helpful kind of exoplanet, leading us to our first exomoons and, of course, offering the opportunity for analysis of their atmospheres through transmission spectroscopy. All in all, Kepler-167e is a satisfying find indeed.
The paper is Kipping et al., “A Transiting Jupiter Analog,” accepted at The Astrophysical Journal (preprint).
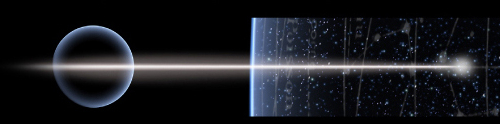
by Paul Gilster | Mar 3, 2016 | Deep Sky Astronomy & Telescopes |
A new paper in Nature offers further information about Fast Radio Bursts (FRBs), which we last looked at only a few days back. The February 24 post examined work on FRBs that were consistent with what has been seen before — transient pulses lasting mere milliseconds, while emitting huge amounts of energy (see Fast Radio Bursts: First Distance Measurement). Now we have further work out of the Max Planck Institute for Radio Astronomy (MPIfR) in Germany that describes the detection of the first source of repeating bursts, an object outside our own galaxy that is producing multiple short bursts.
Lead author Laura Spitler (MPIfR) explains that a McGill University graduate student named Paul Scholz, using data from the Arecibo radio telescope in Puerto Rico, discovered the repeat signals last November. Scholz found a total of 10 new bursts. “Not only does this source repeat, but the brightness and spectra of the bursts also differs from those of other FRBs,” adds Spitler. We may be looking at a new class of FRB, one perhaps drawing on extremely powerful flare activity in a rotating neutron star.
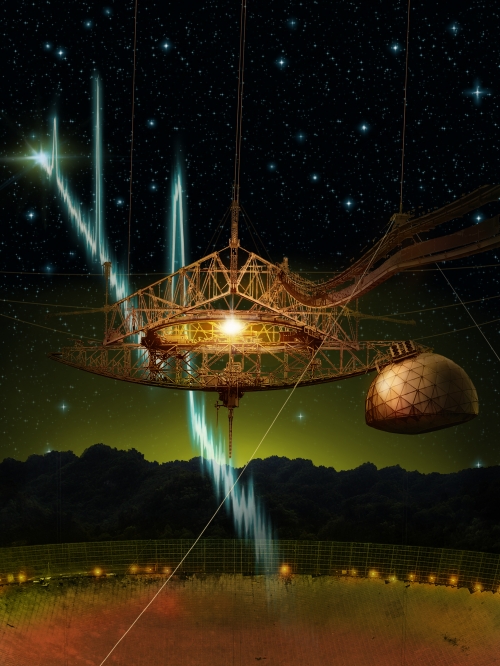
Image: The 305-m Arecibo telescope and its suspended support platform of radio receivers is shown amid a starry night in this artist’s impression. From space, a sequence of millisecond-duration radio flashes are racing towards the dish, where they will be reflected and detected by the radio receivers. Such radio signals are called Fast Radio Bursts, and Arecibo is the first telescope to see repeat bursts from the same source. Credit: Danielle Futselaar.
This MPIfR news release also points to an upcoming paper in Monthly Notices of the Royal Astronomical Society, which reports on a repeating FRB signature found by the Parkes radio telescope in Australia. Although not yet published, the preprint of this work is available, from which this:
Among the new FRBs is the largest excess DM [dispersion measure, the integrated free electron density along the line of sight] to date giving a redshift limit of <1.3. For the first time structure has been seen in the profile of FRBs with a two-component detection. This poses significant challenges to many of the models of FRB emission which rely on one-off high energy events. However the Cordes & Wasserman (2015) model of “supergiant” pulses and the Popov & Postnov (2007) model of hyperflares could both account for this structure. The rates expected by these models is highly uncertain and cannot at present be used as a discriminator.
A word on the term ‘dispersion measure,’ as found above. It is possible to distinguish a pulse from man-made interference because electrons in the interstellar medium cause radio waves to travel more slowly at lower radio frequencies. You’ll note above that the dispersion measure in the new observations is said to be the largest to date. All ten of the newly discovered bursts have a far higher dispersion measure than would be found in a source within our galaxy.
So now we have a bit of a conundrum. The work discussed here on the 24th was also produced by researchers at the Max Planck Institute for Radio Astronomy and likewise published in Nature. It focused on FRBs as cataclysmic events like gamma-ray bursts, which would not generate repeat occurrences. Now we are talking about a repeating FRB that results from an extragalactic neutron star. The implication: There are multiple ways to produce an FRB.
The plan going forward is to use interferometry to study the origins of these radio bursts. Jason Hessels (University of Amsterdam), a corresponding author on the Nature paper, notes the possibilities:
“Once we have precisely localized the repeater’s position on the sky, we will be able to compare observations from optical and X-ray telescopes and see if there is a galaxy there. Finding the host galaxy of this source is critical to understanding its properties.”
I may have the exact number wrong and probably do, but at best the number of FRBs thus far detected is still tiny — I make out 32 events so far. At any rate, each new event has the potential for revising our understanding of these unusual phenomena.
The paper is Spitler et al,, “A repeating fast radio burst,” published online by Nature 02 March 2016 (abstract). The upcoming MNRAS paper is Champion et al., “Five new Fast Radio Bursts from the HTRU high latitude survey: first evidence for two-component bursts.” submitted to Monthly Notices of the Royal Astronomical Society (preprint).
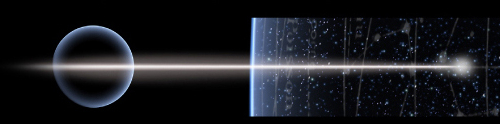
by Paul Gilster | Mar 2, 2016 | Astrobiology and SETI |
I’m still smarting about having to cancel my travel plans for the Tennessee Valley Interstellar Workshop in Chattanooga, particularly since the Tau Zero Foundation was one of the sponsors of the event. But fortunately, I do have people offering to write up the workshop for Centauri Dreams, so we’ll have some coverage and photos soon. Onward…
Hunting for Biosignatures
We only have two years before the James Webb Space Telescope is scheduled to launch. Assuming all goes well, JWST should help ease us into the era of biosignature detection, as we look for the characteristic signs of living organisms in the atmospheres of their worlds. But just how definitive are such signatures? A new paper from the University of Washington digs into potential false positives and offers specifics on the signatures that could fool us.
One way to study biosignatures is by transit spectroscopy, using data gathered from starlight as it passes through a planet’s atmosphere during a transit. This allows us to analyze the features of light that flag particular atmospheric constituents. Would a strong oxygen signature necessarily indicate life? You would think so, because the oxygen in Earth’s atmosphere, O2, is unstable over geological timeframes and would gradually be depleted through reactions with volcanic gases and oxidation at the surface.
On our planet, then, oxygen needs a replenishing source, which is provided through photosynthesis as plants and algae tap sunlight for energy. But Edward Schwieterman and team argue that while abiotic oxygen will not build up to significant levels on Earth, it can certainly arise in a number of different planetary settings. That fact should give us pause.
Working under the aegis of the university’s Virtual Planet Laboratory, Schwieterman sees oxygen as a potential ‘biosignature impostor.’ The abiotic creation of oxygen, particularly around the kind of low mass stars that are likely to be our first targets for this kind of work, can happen when ultraviolet light from the star splits carbon dioxide molecules, allowing some of the oxygen atoms to form O2. We get oxygen without any requirement for biology to sustain it.
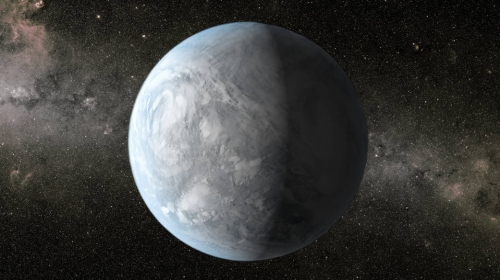
Image: New research from the University of Washington-based Virtual Planetary Laboratory will help astronomers better identify and rule out “false positives” in the ongoing search for life. Shown is an artist’s rendering of Kepler 62E, about 1,200 light-years away in the constellation Lyra. Credit: NASA
Usefully, Schwieterman’s computer models show that this process will also produce detectable amounts of carbon monoxide. Thus the presence of carbon dioxide and carbon monoxide would raise doubts about the simultaneous detection of oxygen in that planetary atmosphere. Abiotic processes rather than life might well be the agent. We can use this to our advantage:
…in the cases presented here, the spectral discriminators against abiotic O2/O3 are more detectable with a hypothetical JWST observation than the O2 or O3 signatures themselves. In our example spectra, neither O2 nor O3 would be directly detectable with just 10 transits, but the abiotic discriminators CO/CO2 and O4 could be. This provides an opportunity to maximize the utility of observing time if the ultimate goal is to characterize planets where true biosignatures are obtainable. If spectral indicators for biosignature impostors are detected with reasonable confidence, the community may wish to reallocate the remaining time to other promising targets, rather than integrate further.
The mention of O4 above is a reference to a second kind of biosignature impostor, where light from the primary star breaks down atmospheric water on the rocky planet under investigation. In this case, we get large amounts of oxygen as the hydrogen escapes, and might expect to find short-lived pairs of oxygen molecules that become O4 molecules, producing a distinctive signature of their own. A higher percentage of oxygen is produced than Earth has ever had in its atmosphere, as Schwieterman notes:
“Certain O4 features are potentially detectable in transit spectroscopy, and many more could be seen in reflected light. Seeing a large O4 signature could tip you off that this atmosphere has far too much oxygen to be biologically produced.”
Detecting this particular ‘biosignature impostor’ is best done through direct imaging, while carbon dioxide and carbon monoxide signatures are more readily identified through transit spectroscopy. The paper looks at simulations of direct imaging spectra:
This simple test case demonstrates that if the high-O2 atmospheres proposed by Luger & Barnes (2015) exist, the O4 absorption band strength in those planetary spectra would rival or exceed that of the monomer O2 bands. These spectra are qualitatively different than modern-Earth’s spectrum, even in the 0.3-1.0 µm range, with a different shape, broader O2 features, and additional features from O4. These are all signs of a much higher O2 abundance than the Earth’s atmosphere – self-regulated by negative feedbacks – has ever achieved.
Schwieterman and team believe the first potentially habitable planets whose atmospheres we study will be orbiting M-class dwarf stars, the kind of planets most likely to show both kinds of biosignature impostors discussed above. Thus we’ll be faced with the need to interpret potential biosignatures that could be confusing without these criteria. Says Schwieterman:
“The potential discovery of life beyond our solar system is of such a huge magnitude and consequence, we really need to be sure we’ve got it right — that when we interpret the light from these exoplanets we know exactly what we’re looking for, and what could fool us.”
The paper is Schwieterman et al., “Identifying Planetary Biosignature Impostors: Spectral Features of CO and O4 Resulting from Abiotic O2/O3 Production,” Astrophysical Journal Letters Vo.. 819, No. 1 (25 February 2016). Abstract / preprint. A University of Washington news release is also available.
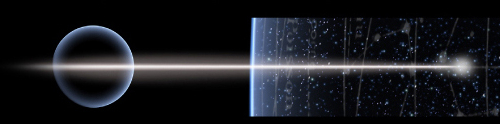