by Paul Gilster | May 6, 2016 | Culture and Society |
Cameron Smith is no stranger to these pages, having examined the role of evolution in human expansion into space (see Biological Evolution in Interstellar Human Migration), cultural changes on interstellar journeys (Human Universals and Cultural Evolution on Interstellar Voyages), as well as the composition of worldship crews (Optimal Worldship Populations). An anthropologist and prehistorian at Portland State University, Dr. Smith today offers up his thoughts on the emerging discipline he calls space anthropology. How do we adapt a field that has grown up around the origin and growth of our species to a far future in which humans may take our forms of culture and consciousness deep into the galaxy? What follows is the preface for Dr. Smith’s upcoming book Principles of Space Anthropology: Establishing an Evolutionary Science of Human Space Settlement, to be published by Springer later this year.
By Cameron M. Smith, PhD
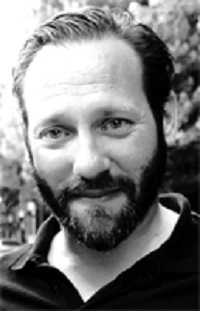
New Realms of Action Require New Domains of Expertise
In 1963, Siegfried J. Gerathewohl, NASA’s biotechnology chief, wrote the following passage early in his foundation text, Principles of Bioastronautics, outlining the need for this new field of study:
“Manned excursions into space require new types of vehicles, machines and hardware which were unknown in conventional flying. They will carry the traveler into such foreign environments as to pose serious problems of health and survival. The new field of medicine, which studies the human factors involved and the protective measures required, has been called space medicine. From its cooperation with modern technology, particularly with electronics, cybernetics, physics, and bionics, space biotechnology has branched out as a novel field of bioengineering.” [1:5-6]
At the time of that publication, less than ten people had been in space; the moon landings were yet vague plans, the robotic reconnaissance of our solar system was in its infancy and virtually nothing was known of human biology in space. Two generations later, space exploration and space sciences are at an historical apex of activity and rapid technical progress. Low Earth Orbit has been continuously occupied by at least one person, continuously, for over a quarter century, yielding thousands of scientific studies on space biology; Mars has been swarmed by robotic explorers seeking traces of life and mapping landscapes for human exploration and settlement; dozens of private companies and even individuals are re-inventing basic space exploration technologies with cheaper materials and methods than those of last century’s space age with the aim of lowering the cost of space access, and astronomy has entered a new age, with space-based technologies identifying multitudes of exoplanets now slated to be examined for traces of life with methods just coming on-line.
One significant outcome of these many efforts to better understand our stellar neighborhood will be the settlement of space by populations of humans and their domesticates. The ancient dream of setting off across space to explore and settle new lands—for freedom, exploration, economic advantage, the safeguarding of humanity by spreading out from the home planet, and a multitude of other motivations—appears more likely than ever, and its earliest steps are being taken now. For example, the SpaceX corporation was founded “…to revolutionize space technology, with the ultimate goal of enabling people to live on other planets” [2] and indeed in October 2016 Elon Musk is set to announce detailed Mars settlement plans. Such proposals involve not just individual people but populations, which have their own biological and behavioral (cultural) properties. In the same way that space exploration required Gerathewohl’s bioastronautics, space settlement planning requires a field of study to ensure that plans are designed and carried out informed by all we know of the adaptive tools and techniques of our species.
A New Branch of Anthropology
Traditionally, the study of humanity’s adaptations has been the domain of anthropology. Over the last century this field has capably documented our species’ remote origins, long and complicated evolution, and myriad manifestations in the present, but it has only occasionally (and then unsystematically) forayed into humanity’s distant future (e.g. see [3,4,5]). It is a premise of this book that that future should include the human settlement of environments beyond Earth, particularly for the purposes of safeguarding humanity’s apparently unique mode of consciousness, its hologenome and many of its domesticates, and the totality of human knowledge—accumulated over about 3,000 generations since the origins of behavioral modernity—by the method of establishing populations of humanity culturally and biologically independent of our home planet. I discuss arguments for space settlement in [5] and [6], but in the present book I focus on how the resources and expertise of anthropology may be deployed to assist in the goals of human space settlement.
While bioastronautics was established during the First Space Age (hereafter FSA) with tight focus on safeguarding the short-term health of individuals or small crews, today, plans include space settlement by communities, which raises many new issues; individual physiology is a different phenomenon than, say, population genetics, and individual psychology as short-term adaptation is different from cultural adaptation by reshaping cultural norms in accordance with new circumstances; I tabulate some other such differences below.
Chief Differences Between Space Exploration and Space Settlement Relating to Adaptation
| Space Exploration | Space Settlement |
Goals | specific, short-term | general, long-term |
Group Size | small (crews) | large (communities) |
Social Organization | command hierarchy | civil community |
Essential Social Units | crews | families and communities |
Adaptive Means | technological, individual behavior, and some reversible acclimatization | technological, cultural and biological adaptation |
Adaptive Timescale | short; weeks to months | long; multigenerational |
For these reasons a new field of study is required. In this book I propose, describe, and outline the scope of space anthropology or exoanthropology, and present some of my own results in this new discipline.
In the same way that Gerathewohl identified the need for his field in the quotation at the opening of this Preface, below I formally outline the need for space anthropology:
Space settlement will require novel biological and cultural adaptations to support populations of humans, on multigenerational timescales, in environments so far unfamiliar to our species even after 100,000 years of human cultural and biological adaptation to myriad Earth environments. The new field of anthropology that studies such adaptive efforts is space anthropology or exoanthropology, exo- referring to beyond Earth, in the same way it is used in the term exobiology.
Specifically, I propose space anthropology to have three main functions:
- 1. To identify the biological and cultural adaptive suite of humanity globally and to date, resulting in a catalog of our species’ adaptive tools and capacities useful to space settlement planners.
- 2. To evaluate the capacities of humanity’s various adaptive tools to adapt to reasonably forseeable space settlement plans, bettering the prospect of productive adaptations to new conditions, e.g. on Mars.
- 3. To make recommendations, some broad and some specific, that would assist in human adaptation to environments beyond Earth, particularly based on evaluations of human adaptive capacities identified in functions 1 and 2.
The scope of exoanthropology, then, will be broad. I propose it as an applied form of anthropology with the specific goal of evaluating the adaptive capacities of our species, both biologically and culturally, so that they may be best deployed to assist in successful permanent space settlement. This will guide space settlement planning in a genuinely adaptive and evolutionarily-informed way, applying the lessons of billions of years of Earth life adaptation to what I consider to be the completely natural and expected dispersal of life throughout the solar system and beyond. This book, then, will thoroughly review the phenomenon of evolutionary adaptation, particularly among our species.
Human ‘adaptive tools’ are biological and cultural (which subsumes technology) [7]; an array of such adaptations so far recognized in the Earth’s cold, high altitude and hot regions are tabulated below as examples—these will be fully explored later in this book.
Some Human Adaptations to Earth Environments
Biome | Limiting Factors | Biological, Cultural and Technological Adaptations |
Arctic / Cold | *Extremely low temperatures for long periods
*Extreme light / dark seasonal cycles
*Low biological productivity | Biological
* increased Basal Metabolic Rate
* increased shivering, vasoconstriction and cold thermoregulation activity and efficiency
* compact, heat-retaining body stature
Cultural and Technological
* bilateral kinship = demographic flexibility
* clothing insulates but can prevent sweating
* semi-subterranean housing including igloo made of local, free, inexhaustible reosurce (snow)
* high fat diet yielding many calories and vitamins
* low tolerance of self-aggrandizement
* low tolerance of adolescent bravado
* high value of educating young
* social fission
* mobile, field-maintainable, reliable tools
* population control methods including voluntary suicide, infanticide
* high value on apprenticeship
* low tolerance for complaint: 'laugh don't cry'
|
High Altitude | * Low oxygen pressures
* Nighttime cold stress
* Low biological productivity
* High neonatal mortality | Biological
* dense capillary beds shorten distance of oxygen transport
* larger placenta providing fetus with more blood-borne oxygen
* greater lung ventilation (capacity)
Cultural and Technological
* promotion of large families to offset high infertility
* use of coca leaves to promote vasoconstriction and caffeine-like alertness
* woolen clothing retains heat when wet
* trade connections with lowland populations |
Arid / Hot | * Low and uncertain rainfall
* High evaporation rate
* Low biological productivity | Biological
* tall, lean, heat-dumping body
* lowered body core temperature
* increased sweating efficiency
* lower urination rate
* increased vasodilation efficiency
Cultural & Technological
* flexible kinship & land tenure system = demographic flexibility matching shifting water resources
* intercourse taboo maintain sustainable population
* loose, flowing clothing blocks sunlight
* wide sandals block ground-reflected sunlight
* nakedness socially accepted during physical labor |
In fulfilling Function 1, exoanthropology will survey humanity’s adaptations through time and across the globe, identifying patterns pertinent to space settlement planners. In fulfilling Function 2, it will review the adaptive competence of many of our species’ adaptive tools, allowing us to evaluate our readiness for space settlement and, where we find ourselves unready, suggest courses of action; it will also characterize forseeable space settlement conditions and limiting factors as needed. In fulfilling Function 3, recommendations for space settlement planners will be formulated, varying in specificity, based on the lessons identified in the surveys serving Functions 1 and 2. Finally, in fulfilling Function 4, directly actionable engineering and other design recommendations will be made, materially assisting in space settlement planning.
References to Author’s Preface
1. Gerathewohl, S. 1963. Principles of Bioastronautics. Prentice-Hall, New Jersey.
2. SpaceX website (accessed 14 April 2016): http://www.spacex.com/about.
3. Finney, B. and E. Jones (eds). 1985. Interstellar Migration and the Human Experience. Berkeley, University of California Press.
4. Finney, B. 1992. Space Migrations: Anthropology and the Humanization of Space. NASA SP-509: Space Resources, Volume 4: Social Concerns. Washington, D.C.
5. Smith, C.M. and E.T. Davies. 2012. Emigrating Beyond Earth: Human Adaptation and: Space Colonization. Springer, Berlin.
6. Smith, C.M. and E.T. Davies. 2005. The Extraterrestrial Adaptation. Spaceflight 47(12):46.
7. Morphy, H. and G. Harrison (eds). 1998. Human Adaptation. Oxford: Oxford University Press.
© 2016 by Cameron M. Smith, PhD
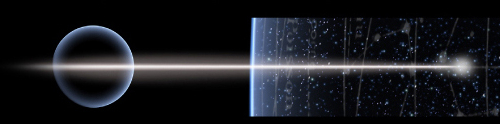
by Paul Gilster | May 5, 2016 | Outer Solar System |
David McComas (Princeton University) calls what his team of researchers have learned about the solar wind at Pluto ‘astonishing,’ adding “This is a type of interaction we’ve never seen before anywhere in our Solar System.” The reference is to data from the Solar Wind Around Pluto (SWAP) instrument that flew aboard New Horizons. McComas knows the instrument inside out, having led its design and development at the Southwest Research Institute.
Image: The first analysis of Pluto’s interaction with the ubiquitous space plasma known as the solar wind found that Pluto has some unique and unexpected characteristics that are less like a comet and more like larger planets. Credit: NASA/Johns Hopkins University Applied Physics Laboratory/Southwest Research Institute.
What startled McComas was that Pluto’s interactions with the solar wind are nowhere near what had been predicted. This stream of charged particles flowing outbound from the Sun can reach speeds of 500 kilometers per second and above, a ragged, bursting outrush that we may one day be able to capitalize on as a way to drive spacecraft. While planets act to divert the solar wind, comets slow it far more gently, an effect that researchers assumed they would find at Pluto.
Instead, Pluto’s complexities place it somewhere between planet and comet as its atmosphere copes with the solar wind. We learn in the paper from McComas and team that despite expectations, Pluto’s gravity is capable of holding electrically charged ions in its extended atmosphere. The dwarf planet has an ion tail extending ‘downwind’ to a distance of about 100 Pluto radii (120,000 kilometers). Earth has a similar ion tail, and Pluto’s is said to show ‘considerable structure.’
From the paper:
Initial studies of the solar wind interaction with Pluto’s atmosphere…, all assuming the absence of an intrinsic magnetic field, suggested that it would depend on whether the atmospheric escape flux is strong — producing a ‘comet-like’ interaction where the interaction region is dominated by ion pick-up and many times larger than the object — or weak — producing a ‘Mars-like’ interaction dominated by ionospheric currents with limited upstream pick-up and where the scale size is comparable to the object.
And Pluto, we learn, behaves more like its larger planetary cousins, as the image below suggests.
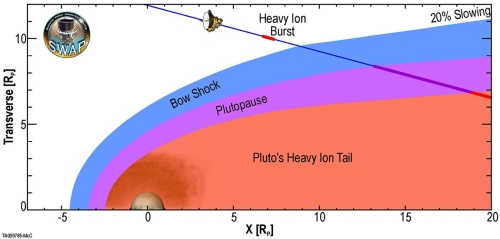
Image: This figure shows the size scale of the interaction of Pluto (at lower left) with the solar wind. Scientists thought Pluto’s gravity would not be strong enough to hold heavy ions in its extended atmosphere, but Pluto, like Earth, has a long ion tail (red) loaded with heavy ions from the atmosphere. Pluto has a very thin “Plutopause” (purple), or the boundary of Pluto’s tail of heavy ion tail and the sheath of the shocked solar wind (blue) that presents an obstacle to its flow. Credit: American Geophysical Union.
The SWAP instrument was able to separate heavy ions of methane, which is the primary gas escaping the atmosphere, from the light hydrogen ions coming from the Sun. From it we also learn that Pluto has a thin ‘Plutopause,’ a boundary region where the heavy ion tail meets the solar wind along a sheath of particles. Moreover, the solar wind is not blocked until it reaches within about about 3000 kilometers of the dwarf planet — the paper calls this the ‘upstream standoff distance’ — a bit less than three Pluto radii.
As the paper notes, we’ll have a good deal of data from different parts of the Solar System to examine as we try to put Pluto into context:
While the small size of the interaction region relative to Pluto is reminiscent of Mars and Venus, we note that recent observations of the solar wind interaction with the relatively weakly out-gassing Comet 67P Churyumov-Gerasimenko by instruments on ESA’s Rosetta spacecraft show deflection of the solar wind with relatively modest decrease in speed. We anticipate interesting scientific discussions of the relative roles of atmospheric escape rate, solar wind flux, and IMF strength at Mars, Comet 67P and Pluto as the data from the MAVEN, Rosetta and New Horizons spacecraft are further analyzed.
And as McComas notes in this Princeton news release, “The range of interaction with the solar wind is quite diverse, and this gives some comparison to help us better understand the connections in and beyond our solar system. The SWAP data will … be reanalyzed … for many years to come as the community collectively grapples with Pluto’s unique solar wind interaction — one that is unlike that at any other body in the solar system.”
The paper is McComas et al., “Pluto’s Interaction with the Solar Wind,” accepted at the Journal of Geophysical Research: Space Physics (abstract / preprint).
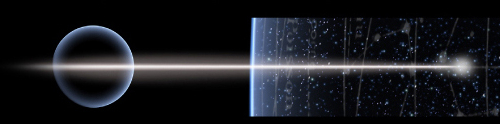
by Paul Gilster | May 4, 2016 | Uncategorized |
When you don’t have the technology to get to an interesting place like the Oort Cloud, it’s more than a little helpful when nature brings an Oort Cloud object to you. At least we think that the object known as C/2014 S3 (Pan-STARRS) has moved into the warmer regions of the Solar System from the Oort. A gravitational nudge in that distant region would be all it took to send the object, with an orbital period now estimated to be 860 years, closer to the Sun.
And here things get interesting, because C/2014 S3 is the first object discovered on a long-period cometary orbit that shows all the spectral characteristics of an inner system asteroid. The level of activity on the object, apparently the result of sublimation of water ice, is five to six orders of magnitude lower than what we would expect from an active long-period comet at a similar distance from the Sun. Karen Meech (University of Hawaii) and colleagues believe that the object formed in the inner system at about the same time as the Earth, after which it was ejected before it could be bathed by long-term solar radiation.
Meech calls C/2014 S3 “…the first uncooked asteroid we have found,” which means we’re not only dealing with a building block of the early Solar System, but one that has been preserved for billions of years in a pristine environment. Further underlining its unusual status is the fact that C/2014 S3 is not developing the tail we would expect from a long-period comet as it approaches the Sun. Hence the moniker ‘Manx object’ given by Meech and team, a reference to the tail-less breed of cat from the Isle of Man.
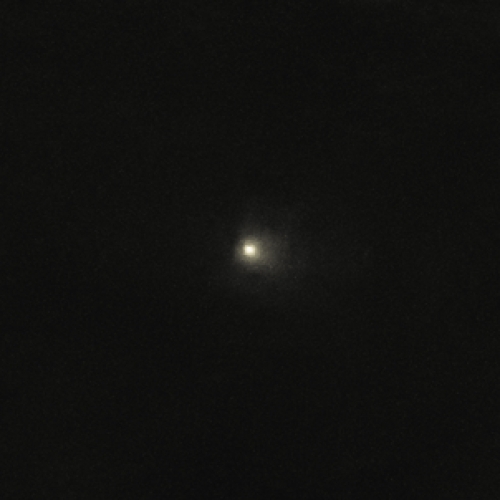
Image: Observations with ESO’s Very Large Telescope, and the Canada France Hawaii Telescope, show that C/2014 S3 (Pan-STARRS) is the first object to be discovered that is on a long-period cometary orbit, but that has the characteristics of a pristine inner Solar System asteroid. It may provide important clues about how the Solar System formed. This image of the comet was acquired using the Canada France Hawaii Telescope. Credit: K. Meech (IfA/UH) / CFHT/ESO.
A population of objects of the C/2014 S3 class would be useful indeed, and as this University of Hawaii Institute for Astronomy news release suggests, would help us differentiate between differing models of Solar System development. The problem is that while several of these models can duplicate the Solar System’s current configuration, some require the migration of the gas giants while others do not. Moreover, the models yield different predictions on the amount of rocky material expelled early on into the Oort Cloud.
Just how widely these models vary is explained in the paper. Here it describes the gas giant migration model:
The “Grand Tack” model starts the simulation of solar system formation at an early phase, when the giant planets grew and migrated in a gas-rich protoplanetary disk. During their inward migration, the giant planets scattered inner solar system material outward; during their outward migration, they implanted a significant amount of icy planetesimals [from 3.5 to 13 astronomical units (AU)] into the inner solar system. The Grand Tack model predicts the presence of rocky objects in the Oort cloud at an icy comets/rocky asteroids ratio of 500:1 to 1000:1…
But how we view the Oort Cloud varies depending upon which model we accept:
Other dynamical models, which assume nonmigrating giant planets, make different predictions about the fraction of the Oort cloud population comprising planetesimals initially within the asteroid belt or the terrestrial planet region. These predictions range from 200:1 to 2000:1. Other models do not explicitly estimate the mass of rocky planetesimals eventually implanted in the Oort cloud, but from the amount initially available, it is reasonable to expect that the ratio of icy planetesimals to rocky planetesimals in the final Oort cloud is between 200:1 to 400:1. Instead, a recent radically different model of terrestrial planet formation predicts that the planetesimals in the inner solar system always had a negligible total mass; in this case, there would be virtually no rocky Oort cloud population.
Be aware that C/2014 S3 is not the first inactive object discovered on a long-period comet orbit, with 1996 PW, found in 1996, being characterized as an extinct comet or asteroid ejected into the Oort Cloud. Five other ‘Manx candidates’ have been observed by Meech’s team, all of them showing colors similar to 1996 PW. C/2014 S3 is the only candidate to date that shows the spectrum of an S-type asteroid, a type usually found in the main asteroid belt.
So it’s an intriguing find, and according to the paper, learning how many S-type objects like it exist in the Oort Cloud will be a useful test of the various models. The paper argues that making a selection between the models will require characterization of up to 100 such ‘Manx objects,’ with the number of S-types helping us determine the ratio of icy to rocky objects in the Oort. As we are now discovering Manx objects on the order of about 15 per year as the Pan-STARRS project continues, scientists will soon have a sufficient number to work with.
The paper is Meech et al., “Inner solar system material discovered in the Oort cloud,” Science Advances Vol. 2, No. 4 (29 April 2016). Full text.
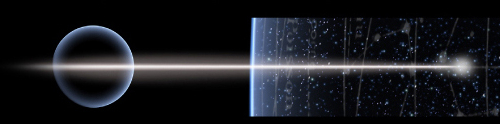
by Paul Gilster | May 3, 2016 | Astrobiology and SETI |
I’ve always found the final factor in the Drake Equation to be the most telling. Trying to get a rough idea of how many other civilizations there might be in the galaxy, Drake looked at factors ranging from the rate of star formation to the fraction of planets suitable for life on which life actually appears. Some of these items, like the fraction of stars with planets, are being clarified almost by the day with continuing work. But the big one at the end — the lifetime of a technological civilization — remains a mystery.
By ‘technological,’ Drake was referring to those civilizations that were capable of producing detectable signals; i.e., releasing electromagnetic radiation into space. And when we have but one civilization to work with as example, we’re hard pressed to know what this factor is. This is where Adam Frank (University of Rochester) and Woodruff Sullivan (University of Washington, Seattle) come into the picture. In a new paper in Astrobiology, the researchers argue that there are other ways of addressing the ‘lifetime’ question.
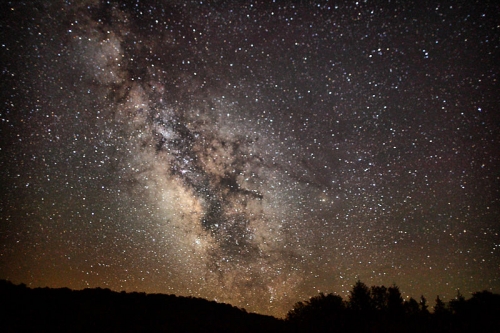
Image credit: http://www.ForestWander.com [CC BY-SA 3.0 us], via Wikimedia Commons.
What Came Before Us
The idea is to calculate how unlikely our advanced civilization would be if none has ever arisen before us. In other words, Frank and Sullivan want to put a lower limit on the probability that technological species have, at any time in the past, evolved elsewhere than on Earth. Here’s how their paper describes this quest:
Standard astrobiological discussions of intelligent life focus on how many technological species currently exist with which we might communicate (Vakoch and Dowd, 2015). But rather than asking whether we are now alone, we ask whether we are the only technological species that has ever arisen. Such an approach allows us to set limits on what might be called the ”cosmic archaeological question”: How often in the history of the Universe has evolution ever led to a technological species, whether short- or long-lived? As we shall show, providing constraints on an answer to this question has profound philosophical and practical implications.
To do this, the authors produce their own equation drawing on Drake’s. Consider A the number of technological civilizations that have formed over the history of the observable universe. Rather than dealing with Drake’s factor L — the lifetime of a technological civilization — Frank and Sullivan propose what they call an ‘archaeological form’ of Drake’s equation. The need for the L factor disappears. The new equation appears in this form:
A = Nast * fbt
Where A = The number of technological species that have evolved at any time in the universe’s past
Nast = The number of habitable planets in a given volume of the universe
fbt = The likelihood of a technological species arising on one of these planets.
You can see that what Frank and Sullivan rely on are recent advances in the detection and characterization of exoplanets. We’re learning a great deal more about how common planets are and how many are likely to orbit in the habitable zone around their star, where liquid water could exist. Their term Nast relies on this work and draws together various terms from the original Drake equation including the total number of stars, the fraction of those stars that form planets, and the average number of planets in the habitable zone of their stars.
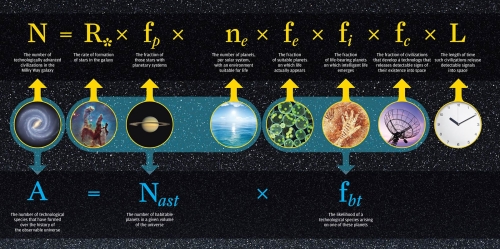
From the paper:
With our approach we have, for the first time, provided a quantitative and empirically constrained limit on what it means to be pessimistic about the likelihood of another technological species ever having arisen in the history of the Universe. We have done so by segregating newly measured astrophysical factors from the fully unconstrained biotechnical ones, and by shifting the focus toward a question of ”cosmic archaeology” and away from technological species lifetimes. Our constraint addresses an issue that is of particular scientific and philosophical consequence: the question ”Have they ever existed?” rather than the usual narrower concern of the Drake equation, ”Do they exist now?”
The paper is short and interesting; I commend it to you. The result it produces is that human civilization can be considered unique in the cosmos only if the odds of a civilization developing are less than one part in 10 to the 22nd power. Frank and Sullivan call this the ‘pessimism’ line. If the probability of a technological civilization developing is greater than this standard, then we can assume civilizations have formed before us at some time in the universe’s history.
And yes, this is a tiny number — one in ten billion trillion. Frank says in this University of Rochester news release that he believes it implies technology-producing species have evolved before us. Even if the chances of civilization arising were one in a trillion, there would be about ten billion civilizations in the observable universe since the first one arose. As for our own galaxy, another civilization is likely to have appeared at some point in its history if the odds against it evolving on any one habitable planet are better than one in 60 billion.
We fall back on cosmic archaeology in suggesting that given the size and age of the universe, Drake’s factor L may still play havoc with our chances of ever contacting another civilization. Sullivan puts it this way:
“The universe is more than 13 billion years old. That means that even if there have been a thousand civilizations in our own galaxy, if they live only as long as we have been around—roughly ten thousand years—then all of them are likely already extinct. And others won’t evolve until we are long gone. For us to have much chance of success in finding another “contemporary” active technological civilization, on average they must last much longer than our present lifetime.”
We can play with this a bit. Taking the Milky Way and choosing a probability of 3 X 10-9, we are likely to be one of hundreds of civilizations that have arisen. But drop that probability to 10-18 (one in a billion billion) and we are likely the first advanced civilization in the galaxy. Yet even with the latter constraint, that would still mean we are one of thousands of civilizations that have developed at some time in the visible universe.
I always appreciate work that frames an issue in a new perspective, which is what Frank and Sullivan’s paper does. We can’t know whether there are other civilizations currently active in our galaxy, but it appears that the odds favor their having arisen at some time in the past. In fact, these numbers show us that we are almost certainly not the first technological civilization to have emerged. Is the galaxy filled with the ruins of civilizations that were unable to survive, or is it a place where some cultures have mastered the art of keeping themselves alive?
The paper is Frank and Sullivan, “A New Empirical Constraint on the Prevalence
of Technological Species in the Universe,” Astrobiology Vol. 16, No. 5 (2016). Preprint available.
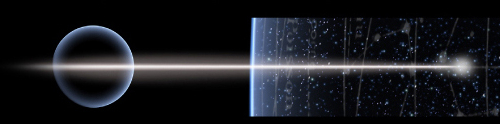
by Paul Gilster | May 2, 2016 | Exoplanetary Science |
About forty light years from Earth in the constellation Aquarius is the star designated 2MASS J23062928-0502285, which as of today qualifies as perhaps the most interesting ultracool dwarf we’ve yet found. What we learn in a new paper in Nature is that the star, also known as TRAPPIST-1 after the European Southern Observatory’s TRAPPIST telescope at La Silla, is orbited by three planets that are roughly the size of the Earth. We may have a world of astrobiological interest — and conceivably several — orbiting this tiny, faint star.
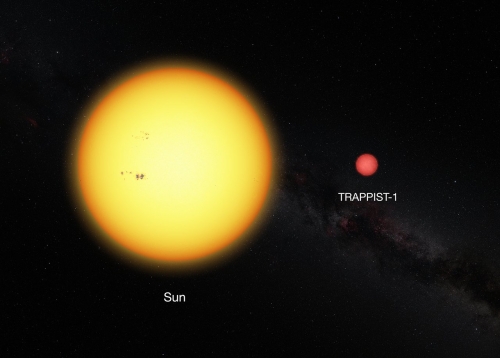
Image: Comparison between the Sun and the ultracool dwarf star TRAPPIST-1. Credit: ESO.
If we untangle the TRAPPIST acronym, we find that it refers not to an order of monks (famous for their beers) but to the TRAnsiting Planets and PlanetesImals Small Telescope, a 60 cm robotic instrument that is operated from a control room in Liège, Belgium. TRAPPIST homes in on sixty nearby dwarf stars at infrared wavelengths to search for planets. Michaël Gillon, who led the team from the University of Liège that made the recent discovery, nails its significance:
“Why are we trying to detect Earth-like planets around the smallest and coolest stars in the solar neighbourhood? The reason is simple: systems around these tiny stars are the only places where we can detect life on an Earth-sized exoplanet with our current technology. So if we want to find life elsewhere in the Universe, this is where we should start to look.”
In other words, life may exist on many worlds, and many of us believe that it does. But at our current level of equipment and expertise, a star like TRAPPIST-1 is significant because the star is small and dim enough for the atmospheres of Earth-sized planets to be studied. We learn from the Nature paper that two of the planets here have orbital periods of 1.5 days and 2.4 days respectively. The third is not as well characterized, with its orbit in a range from 4.5 to 73 days despite follow-up work with ESO’s 8-metre Very Large Telescope in Chile.
Noting how close the planets are to the host star, Gillon likens the scale of the system to that of Jupiter and its larger moons. These are worlds twenty to one hundred times closer to their star than the Earth is to the Sun, but the two inner planets receive only twice and four times respectively the stellar radiation that the Earth receives from the Sun. That puts them too close to the star to be in the habitable zone, while the outer planet may possibly lie within it.
But bear in mind that at least the inner two planets are probably tidally locked, with one side perpetually facing the star, the other turned away from it. Hence there may be regions near the terminator that receive daylight but maintain relatively cool temperatures. Given that the third planet may turn out to be entirely within the habitable zone, we have a fascinating test case for upcoming attempts to characterize the atmospheres of each of these Earth-sized worlds.
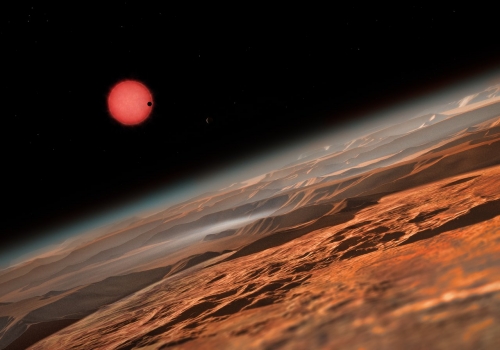
Image: Artist’s impression of the ultracool dwarf star TRAPPIST-1 from close to one of its planets. Credit: ESO.
This ESO news release quotes Julien de Wit, a co-author of the paper on this work, with regard to where we go next:
“Thanks to several giant telescopes currently under construction, including ESO’s E-ELT and the NASA/ESA/CSA James Webb Space Telescope due to launch for 2018, we will soon be able to study the atmospheric composition of these planets and to explore them first for water, then for traces of biological activity. That’s a giant step in the search for life in the Universe.”
The paper is Gillon et al., “Temperate Earth-sized Planets Transiting a Nearby Ultracool Dwarf Star,” published online in Nature 2 May 2016 (abstract). I’ll note too the continuing interest Centauri Dreams reader Harry R. Ray has shown in TRAPPIST 1, and thank him for bringing it to my attention before these first news reports surfaced.
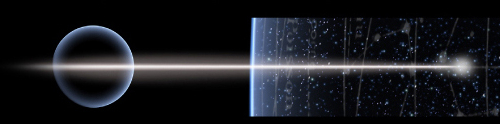