by Paul Gilster | Jul 15, 2016 | Outer Solar System |
A dwarf planet designated 2015 RR245 (and now in search of a name) has been found in an orbit that takes it out to at least 120 AU. It’s a discovery made by the Outer Solar System Origins Survey (OSSOS), an international collaboration focused on the Solar System beyond Neptune. The goal is to test models of how the Solar System developed by studying the movements of icy objects, many of which may have been destroyed or ejected from the Solar System altogether through movements of the giant planets early in the formation process.
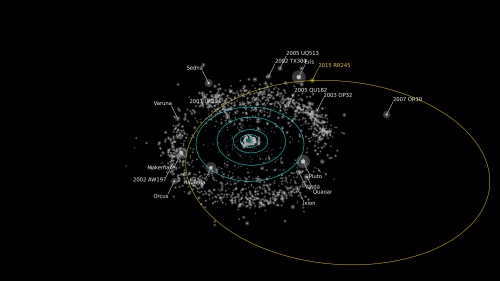
Image: Rendering of the orbit of RR245 (orange line). Objects as bright or brighter than RR245 are labeled. The blue circles show the projected orbits of the major planets. The Minor Planet Center describes the object as the 18th largest in the Kuiper Belt. Credit: Alex Parker/OSSOS team.
We’ve had a close look at one dwarf planet at the edge of the system when New Horizons flew past Pluto a year ago, and with a diameter of roughly 700 kilometers, 2015 RR245 will itself be worthy of future investigation. We now know from the New Horizons example that distant icy worlds like these produce exotic landscapes and unexpected geological processes. But their orbits may tell us just as much as their surface features. Consider:
Perihelion for 2015 RR245 will take place toward the end of this century, though refining its orbit will take time. According to the project’s website, OSSOS can work out TNO orbits in about 16 months on average, which is actually less than 1% of the time it takes them to orbit the Sun. While thinking about these orbits, bear in mind that although the number of TNOs so far discovered is growing, we’re surely seeing a small subset of what must be a vast population.
We’re tracking, in other words, only those objects that are relatively close to the Sun and thus are the easiest to spot. Michele Bannister (University of Victoria BC) touches on the matter in this news release:
“The icy worlds beyond Neptune trace how the giant planets formed and then moved out from the Sun. They let us piece together the history of our Solar System. But almost all of these icy worlds are painfully small and faint: it’s really exciting to find one that’s large and bright enough that we can study it in detail.”
But the fact that we find so few TNOs that are large and bright enough for such study is itself an issue. The second Kuiper Belt Object found (1992 QB1) was discovered over sixty years after the first (Pluto), an indication of how small and faint these objects are. Ethan Siegel speaks to this in an essay for Forbes, noting that objects like 2015 RR245 have extremely eccentric orbits, in this case one with perihelion at roughly 34 AU and aphelion at 120 AU.
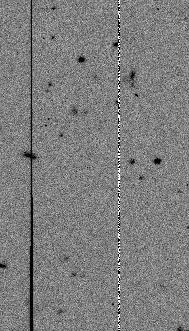
We’re reminded that we have not just Kuiper Belt Objects to contend with but scattered disk objects as well (scattered disk objects are considered to be KBOs with large orbital eccentricities), and beyond them the so-called Sednoids. This has implications for the conjectured Planet Nine, whose existence has been inferred through the clustering of a small number of Sednoid objects. Siegel has his doubts, and they’re reinforced by 2015 RR245:
But it’s also possible, as scattered disk objects and elliptical KBOs show, that there are a huge variety of objects with tremendously varied orbits out there, and we’re only seeing a tiny fraction of them. If the objects we’re seeing have even a slight bias to them, it could lead us to jump to all sorts of incorrect conclusions, just as we did decades ago claiming periodic mass extinctions due to asteroid impacts and the Nemesis theory of a second Sun. Incomplete data is what we’ve got, and the first results of OSSOS and the discovery of 2015 RR245 should remind us all of how much more there is — not just in the Universe but even in our Solar System — still left to discover.
Image: Discovery images of RR245. The images show RR245’s slow motion across the sky over three hours. Credit OSSOS team.
Meanwhile, OSSOS plugs away, having already discovered more than five hundred new trans-Neptunian objects. 2015 RR245 is its largest discovery and the only dwarf planet found by the team, which uses the MegaPrime camera, an imager on the 3.6m Canada-France-Hawaii Telescope (CFHT). As larger telescopes like the Large Synoptic Survey Telescope (LSST) come online capable of mapping the entire visible sky in just a few nights, we will doubtless gain a better idea of the actual distribution of these varied objects. That may put to bed once and for all the question of whether a large undiscovered planet is out there at system’s edge.
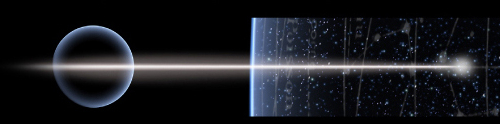
by Paul Gilster | Jul 14, 2016 | Outer Solar System |
We don’t have high-resolution pictures of Jupiter from the Juno mission yet, but we do have JunoCam in operation. It’s a color camera working in visible light that has returned data following the spacecraft’s arrival at Jupiter on July 4. This JPL news release tells us that JunoCam was folded into the mission as part of NASA’s public outreach. It is not, in other words, considered a science instrument, and we’ll need to wait until late August for the first high-resolution images. Still, it’s satisfying to see that all is apparently well in Jupiter space.
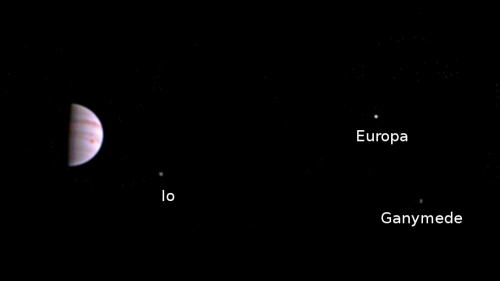
Image: This color view from NASA’s Juno spacecraft is made from some of the first images taken by JunoCam after the spacecraft entered orbit around Jupiter on July 5th (UTC). The view shows that JunoCam survived its first pass through Jupiter’s extreme radiation environment, and is ready to collect images of the giant planet as Juno begins its mission. Credit: NASA/JPL-Caltech/SwRI/MSSS.
Here we’re about 4.3 million kilometers from Jupiter on the outbound leg of the initial 53.5-day capture orbit, a view that yields atmospheric features including the Great Red Spot, along with three of the Galilean moons. Io, Europa and Ganymede appear from left to right in the image. Bear in mind that as the mission progresses, Juno will at times close to within 4100 kilometers of the cloud tops. Spectacular high-resolution views are ahead.
The View from Ceres
We might well find ice deposits on the surface of Ceres. That’s the word from researchers with the Dawn mission, who have been looking at permanently shadowed areas on the dwarf planet. Areas like these are generally on a crater floor or along a part of the crater wall facing the pole. With temperatures below -151 degrees Celsius (122 K) these areas become cold traps, where water ice can accumulate and remain stable for a billion years.
“The conditions on Ceres are right for accumulating deposits of water ice,” said Norbert Schorghofer, a Dawn guest investigator at the University of Hawaii at Manoa. “Ceres has just enough mass to hold on to water molecules, and the permanently shadowed regions we identified are extremely cold — colder than most that exist on the moon or Mercury.”
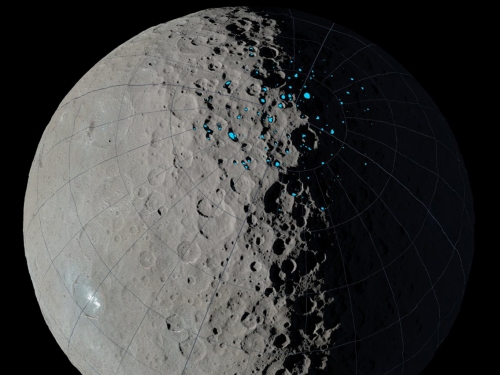
Image: At the poles of Ceres, scientists have found craters that are permanently in shadow (indicated by blue markings). Such craters are called “cold traps” if they remain below about minus 151 degrees Celsius. These shadowed craters may have been collecting ice for billions of years because they are so cold. Credit: NASA/JPL-Caltech/UCLA/MPS/DLR/IDA.
Using computer modeling in conjunction with Dawn’s camera imagery, Schorghofer and colleagues could analyze surface features in great detail, learning which areas receive direct sunlight and how changes during the course of a year on Ceres (1680 days) affect the solar radiation reaching the surface. Dozens of permanently shaded regions turned up in the northern hemisphere, the largest (inside a 16-kilometer crater) less than 65 kilometers from the north pole. Altogether, these regions make up about 1800 square kilometers.
As opposed to the Moon and Mercury (which, like Ceres, have a very small spin axis tilt, or obliquity), the cold trap regions on Ceres extend much further toward the equator. The permanently shadowed regions have to be close to the poles on the Moon and Mercury to get cold enough for ice to remain stable. But like Mercury, these areas account for about the same fraction — less than one percent — of the surface area of the northern hemisphere, and most of these areas on Ceres are cold enough to serve as efficient cold traps for water ice.
All of this has useful implications if we’re thinking ahead to one day exploiting Ceres’ resources:
“While cold traps may provide surface deposits of water ice as have been seen at the moon and Mercury, Ceres may have been formed with a relatively greater reservoir of water,” said Chris Russell (UCLA), principal investigator of the Dawn mission. “Some observations indicate Ceres may be a volatile-rich world that is not dependent on current-day external sources.”
It’s also interesting to note that we don’t know the origin of the ice in the cold traps of either Mercury or the Moon. A possible source is incoming comets, meteorites or interplanetary dust particles, but as the paper on this work notes, we might also find ice generated from solar wind interactions (a mechanism not fully understood) or even outgassing. We would expect, however, that solar wind-generated water resources would be less common with greater distance from the Sun, while infalling H2O would be higher on Ceres than on Mercury because of Ceres’ location in the asteroid belt. Which leads us, the paper notes, to a way to investigate further:
…the trapping efficiency on Mercury and Ceres are similar; that is, for the same number of generated water molecules per surface area, the thickness of the ice accumulating in the cold traps should be almost as high on Ceres than on Mercury. Hence, significant differences in the thickness of young ice deposits may reveal the main source of water. A lack of ice deposits in Cerean cold traps would suggest that infall is not a major source of this ice, consistent with a solar wind generation mechanism on Mercury.
We can, the paper argues, expect fresh and perhaps optically bright ice deposits in the cold traps of Ceres, even without any further water delivery. The authors calculate that about 1 out of every 1000 water molecules generated on the surface of Ceres will wind up in a cold trap during a Cerean year. The upshot: Thin but detectable ice deposits over a 100,000 year period.
The paper is Schorghofer et al., “The permanently shadowed regions of dwarf planet Ceres,” Geophysical Research Letters 6 July 2016 (full text). A JPL news release is also available.
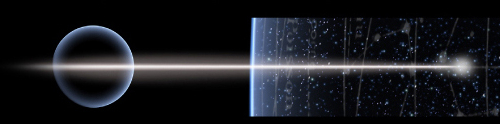
by Paul Gilster | Jul 13, 2016 | Exoplanetary Science |
A team led by Lucas Cieza (Universidad Diego Portales, Santiago, Chile) has produced the first image directly showing the water snowline in a protoplanetary disk, using the Atacama Large Millimeter/submillimeter Array (ALMA). It’s fascinating to actually see a mechanism we’ve long discussed in these pages when analyzing exoplanetary systems (or for that matter, our own). We have a young star called V883 Orionis to thank for the possibility. It’s an FU Orionis star of the kind we recently looked at in FU Orionis: Implications of Sudden Brightening for Planet Formation. And here, too, the implications are rich.
FU Orionis stars are young, pre-main sequence objects that can produce extreme changes in magnitude and spectral type. The eponymous FU Orionis itself, 1500 light years away in the constellation Orion, underwent an event in 1936 that took it from a visual magnitude of 16.5 to 9.6. In the case of V883 Orionis, a similar outburst in temperature and luminosity has heated the protoplanetary disk while making the star 400 times more luminous than the Sun, although it is only 30% more massive. The snowline has been pushed out to a distance great enough for us to observe.
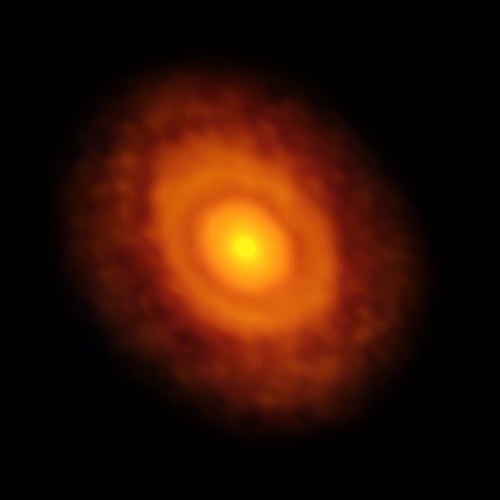
Image: This image of the planet-forming disc around the young star V883 Orionis was obtained by ALMA in long-baseline mode. This star is currently in outburst, which has pushed the water snowline further from the star and allowed it to be detected for the first time. The dark ring midway through the disc is the water snowline, the point from the star where the temperature and pressure dip low enough for water ice to form. Credit: ALMA (ESO/NAOJ/NRAO)/L. Cieza.
The protoplanetary disk surrounding a young star is usually gaseous within several AU of the star, but beyond this distance, lowering temperatures and pressure allow water to sublimate; i.e., water molecules can go directly from the gaseous state to form ice on dust grains and other particles, with no intervening liquid state. Sublimation contributes to the ability of dust grains to clump together, a key process in the beginning of planet formation.
Because water freezes at a relatively high temperature, the water snowline is usually too close to a young star to allow observatories to distinguish it. But because of the star’s recent outburst, V883 Orionis’ snowline has been pushed out ten times as far as usual, to a distance of about 40 AU, roughly the size of Pluto’s orbit. This allows ALMA, which has a resolution at this distance of about 12 AU, to produce the above image.
This kind of outburst is likely a stage that most planetary systems go through. In other words, what we are seeing here may well be common in infant systems, giving us a look at how planet formation occurs throughout the galaxy. Bear in mind that the snowlines for other molecules have already been observed. As distance from a star increases and temperatures drop, molecules like carbon dioxide (CO2), carbon monoxide (CO) and methane (CH4) exhibit snowlines of their own, likewise aiding the planet formation process. The snowline of carbon monoxide around TW Hydrae was imaged by ALMA in 2013.
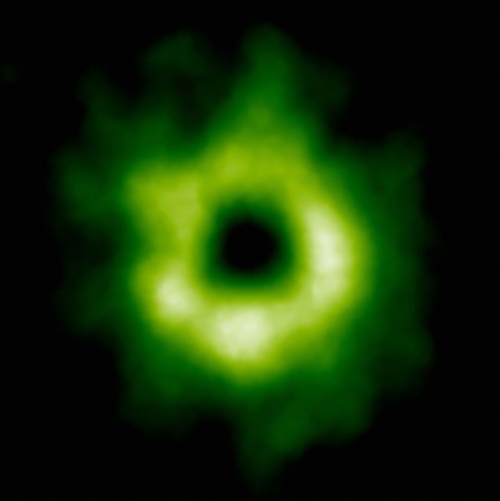
Image: This ALMA image shows the region where carbon monoxide snow has formed around TW Hydrae. The carbon monoxide is shown here in green, and begins at a distance of more than 30 astronomical units from TW Hydrae. Aside from being necessary for planetary and comet formation, carbon monoxide is needed for the creation of methanol, which is a fundamental building block required for life. Credit: ALMA (ESO/NAOJ/NRAO).
We still have much to learn about V883 Orionis-style stellar outbursts, but the assumption is that large amounts of disk material are being consumed by the star, flash-heating the disk. These sudden accretion events add mass to the star while temporarily adjusting the snowlines for various molecules. The presence of the water snowline between Mars and Jupiter during the formation of the Solar System helps us understand how rocky planets form, in a region where water is vaporized well inside the snowline, with gaseous planets forming beyond this limit.
The paper is Cieza et al., “Imaging the water snow-line during a protostellar outburst,” Nature 14 July 2016.
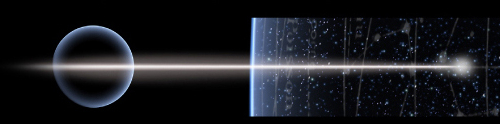
by Paul Gilster | Jul 12, 2016 | Deep Sky Astronomy & Telescopes |
Because we want to learn more about how stars form, we study the so-called Initial Mass Function, which tells us, for a given population of stars, the distribution of their initial masses. As one recent reference (the Encyclopedia of Astrobiology, Springer, 2011) puts it: “The initial mass function is the relative number of stars, as a function of their individual initial mass, that forms during a single star forming episode.” Figuring out the IMF for places like the Orion Nebula, visible from Earth with the naked eye as a patch in Orion’s sword, is a start in learning how this grouping of stars formed.
About 1350 light years from Earth, the Orion Nebula is known as an H II region, a reference to the fact that it contains ionized hydrogen in its star-forming, gaseous depths. A surprise emerged when the European Southern Observatory put its HAWK-I infrared instrument on the Very Large Telescope to work on the nebula. What turned up were faint brown dwarfs and isolated objects of planetary mass in much larger numbers than expected. Says Amelia Bayo (Universidad de Valparaíso, Chile), a co-author of the paper describing the results:
“Understanding how many low-mass objects are found in the Orion Nebula is very important to constrain current theories of star formation. We now realise that the way these very low-mass objects form depends on their environment.”
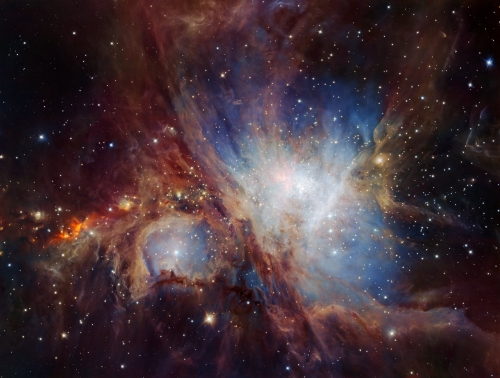
Image: A deep infrared view of the Orion Nebula from HAWK-I. Credit: ESO.
Indeed. The sheer numbers involved here show us that the Orion Nebula may be forming far more low-mass objects than less active star formation areas. The study finds that apart from 929 low-mass stars (less than 1.4 times the mass of the Sun), the Orion Nebula’s Initial Mass Function contains some 757 brown dwarf candidates and 158 ‘isolated planetary mass objects’ (IPMO). The latter have masses greater than 0.005 Solar masses. Overall, the study finds a high fraction of substellar objects (about 50%), ten times more than previously estimated.
Before HAWK-I went to work, the largest grouping of objects known in the Orion Nebula were about one quarter as massive as the Sun. We now have a second maximum to work with at a much lower level of mass. How to explain this ‘bimodal Initial Mass Function’? The authors argue that the excess of brown dwarfs and planetary mass objects is probably produced by ejected material from circumstellar disks, citing recent simulations on pre-stellar disk fragmentation. “We suggest that the final explanation for Orion’s bimodal IMF has to be searched for along that direction.”
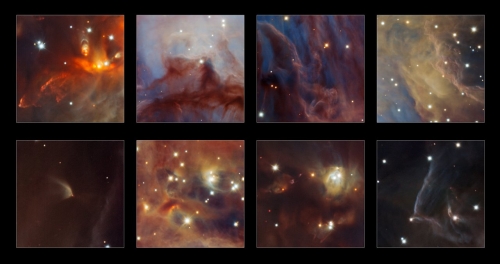
Image: Highlights from a new infrared image of the Orion Nebula. Credit: ESO.
Exactly how we define a brown dwarf is not yet a settled matter, as we saw in the discussion of WISE 0855 in yesterday’s post. One common definition places brown dwarfs between roughly 13 and 75 Jupiter masses, with smaller objects sometimes referred to as rogue planets or sub-brown dwarfs. The paper we looked at yesterday by Andrew Skemer et al. calls WISE 0855 a brown dwarf although it is about five Jupiter masses, evidently because it is thought to have evolved from a collapsing protostellar cloud rather than within a protoplanetary disk.
As we work out such definitions, we have a great deal to learn about objects throughout this mass range. The Orion Nebula is considered a benchmark for studying the Initial Mass Function of young star-forming regions and the substellar mass objects that also populate them. The HAWK-I instrument (High Acuity Wide-field K-band Imager) observes in the 0.9-2.5 μm near-infrared spectral range to see through dust to detect cooler stars and planets. The fact that we have numerous planet-sized objects showing up at the current observational limit raises hopes that the European Extremely Large Telescope and other ELTs slated for the 2020s will be able to mount an observing campaign targeting these and perhaps much smaller worlds.
The paper is Drass et al., “The bimodal initial mass function in the Orion Nebula Cloud,” accepted at Monthly Notices of the Royal Astronomical Society (abstract / preprint).
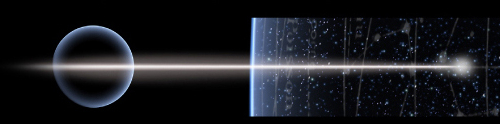
by Paul Gilster | Jul 11, 2016 | Exoplanetary Science |
A brown dwarf as a ‘quieter’ version of Jupiter? That’s more or less the picture offered in a new paper on WISE 0855 from Andrew Skemer (UC-Santa Cruz) and colleagues. Here we’re working in the Solar System’s close neighborhood — WISE 0855 is a scant 7.2 light years from Earth — and we’re observing an object that is the coldest known outside of the Solar System. That makes the observational task difficult, but it has yielded rich results in the discovery of clouds of water or water ice.
We learn that WISE 0855 is about five times the mass of Jupiter, with a temperature in the range of 250 K (-23 Celsius). This is the nearest known object of planetary mass, but it is too faint to characterize with conventional spectroscopy — separating light into its component wavelengths — in the optical or near infrared. But it turns out the object can be studied through thermal emissions from deep in its atmosphere in the range of 5 µm (a range frequently used to study Jupiter’s own deep thermal emissions). Skemer calls spectroscopy of such a faint object in this 5 µm ‘window’ “challenging but not impossible.”
And while we have no current space observatories that can perform 5 µm spectroscopy, we can use ground-based instruments working through the 4.5-5.2 µm atmospheric window of our own planet, although the target is a dim object indeed and observations are limited by the brightness of the Earth’s sky. WISE 0855 becomes the faintest object yet detected with ground-based 5 µm spectroscopy, fully five times fainter than the previous such detection.
The observations were performed with the Gemini-North telescope and the Gemini Near Infrared Spectrograph at Mauna Kea, where the infrared background is the lowest for any telescope of its class. Skemer and team observed WISE 0855 over a thirteen night period for a total of about 14 hours. The resulting spectrum reveals the presence of atmospheric water vapor and clouds. The results depict an object with an appearance similar to Jupiter, a place that fits the team’s calculations involving an atmosphere dominated by water vapor.
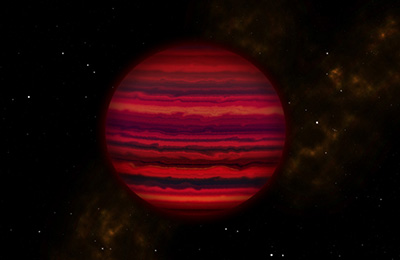
Image: Artist’s rendering of WISE 0855 as it might appear if viewed up close in infrared light. Credit: Joy Pollard, Gemini Observatory/AURA.
The cloud models used here make no assumptions about the composition of cloud being observed, but the paper notes that the temperature of WISE 0855 is such that a composition of water or water ice is likely. Even so, the authors add that the fit of their cloudy model to the observations is not exact, suggesting that there is further complexity here, perhaps involving the vertical and horizontal structure of the clouds, which would affect the brown dwarf’s appearance.
How to move forward from these results? The paper suggests the following:
To understand the nature of clouds and vertical mixing in cold gas giants, we need 5 µm spectra of objects across a continuum of temperatures. After Jupiter (130 K) and WISE 0855 (250 K), the next coldest object with a 5 µm spectrum is Gl 570D (700 K) (Sorahana & Yamamura 2012). In the near term, ground-based telescopes have the sensitivity to obtain spectra of a handful of objects in this temperature range. Future facilities, like the James Webb Space Telescope (JWST), will have the sensitivity to characterize cold gas giants with higher precision, and at wavelengths not possible from Earth (Morley et al. 2014).
As mentioned above, it will be interesting to see what we can learn about Gl 570D, which is a cool brown dwarf about 1500 AU from the triple star system Gl 570, itself composed of a K-class dwarf separated by 190 AU from two red dwarfs that orbit the primary. The brown dwarf here has an estimated mass about 50 times greater than Jupiter and a temperature of about 700 K. The system is approximately 19 light years from Earth.
And we should bear in mind that next-generation Earth-based telescopes (the so-called Extremely Large Telescopes, or ELTs) will have the ability to study large samples of exoplanets in the 5 µm atmospheric window, allowing us to compare not just them but brown dwarfs of various sizes and temperatures with the gas giants here in the Solar System.
Given WISE 0855’s similarities to Jupiter especially regarding the water absorption features in its spectrum, it’s interesting to note the differences. The researchers found that the spectrum of WISE 0855 does not show a strong phosphine (PH3) signal:
If WISE 0855 had Jupiter’s abundance of PH3, it would easily be visible in our spectrum. The fact that WISE 0855 has less PH3 than Jupiter most likely implies that WISE 0855 has less turbulent mixing than Jupiter, although atmospheric metallicity and gravity may also play a role.
So we have a less turbulent atmosphere than Jupiter’s on a cloudy world that probably looks much like the giant planet. We’re probing dynamical and chemical features of an ultra-cool brown dwarf, characteristics we have long studied in our own gas giants. Lacking the mass to spark fusion following the gravitational collapse of the original proto-stellar cloud, WISE 0855 is an ‘almost star’ that may eventually teach us much about the formation of such objects as we expand our range of observations to perform similar studies of gas giant exoplanets.
The paper is Skemer et al., “The First Spectrum of the Coldest Brown Dwarf,” accepted at Astrophysical Journal Letters (preprint). A UC-Santa Cruz news release is available.
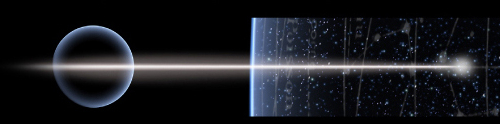