by Paul Gilster | Apr 24, 2006 | Communications and Navigation |
Centauri Dreams is saddened to learn of the death of Eberhardt Rechtin, a pioneer of deep space exploration. The list of his accomplishments is long: Rechtin served as CEO of Aerospace Corporation (El Segundo, CA), as chief engineer of Hewlett-Packard, and as director of the Defense Advanced Research Projects Agency. But his career is best remembered for his work on the Deep Space Network that today allows us to track probes at the edge of the heliosphere.
Building that network was a towering achievement, and one that seemed unlikely in the 1950s, when Rechtin developed Microlock, a system of receivers that, when deployed at different ground stations, could compensate for the shifting frequencies produced by rockets in flight. Those of us who remember the days right after Sputnik may recall that there was an intense effort to expand America’s ground tracking capabilities thereafter. Microlock was at the heart of it, expanding to the system that tracked the first US satellites. The Deep Space Instrumentation Facility would emerge as its successor.
DSIF grew as early robotic flights to the Moon became feasible and the first parabolic dish went up at Goldstone in California’s Mojave Desert. The system received an early shakeout with the launch of Pioneer 3, communicating with the spacecraft throughout its failed attempt to reach the Moon. Rechtin could see that a worldwide system of receivers would be needed for future space work, one that could provide round-the-clock coverage through stations at Woomera (Australia), north of Johannesburg in South Africa and Goldstone itself.
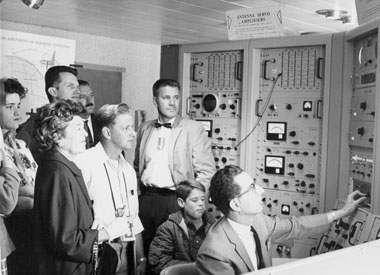
Image: Eberhardt Rechtin (far left) at the Goldstone facility in 1963. Credit: NASA/JPL.
By late 1963, DSIF had become the Deep Space Network. The big 210-foot dish that followed at Goldstone was complemented by changes of venue elsewhere, from Woomera to a site near Canberra, and out of South Africa to Robledo, near Madrid. An article on the DSN by Mark Wolverton quotes Rechtin on the challenges involved:
“I was told by Nobel Prize winners that it would not be possible really to communicate to the edge of the solar system,” Rechtin recalled in a 1995 oral-history interview with the Institute of Electrical and Electronics Engineers. “If you could, you couldn’t send back enough interesting information. You would have to have bandwidths of your receiving system wide enough to account for the Doppler shifts. If you did that you had to send megawatts of power back from enormous antennas at the edge of the solar system. Nobody knew how to do that… . Well, I sat down and figured out, ‘Wait a minute. We could track the Doppler.'”
Rechtin’s work at perfecting the phase-locked loop receiver helped the network compensate for the Doppler effect: the changes in frequency and wavelength caused by the relative motion between spacecraft and receiver on Earth. And his labors on solid-state ruby crystal masers (maser = microwave amplification by stimulated emission of radiation) allowed the creation of the world’s most sensitive radio receivers.
To say that sensitivity is critical for deep space work is a gross understatement. Ponder this: When Voyager’s 23-watt signal reaches us today, it delivers 20 billion times less power than a digital wristwatch, and its beam width is a thousand times greater than Earth’s diameter. But we’re still tracking the Voyagers, and plan to continue at least until 2020.
You can read more about the fascinating early days of the DSN in the Wolverton article, but do read Rechtin’s obituary in the Los Angeles Times as well. And let me throw in a plug for Wolverton’s outstanding book The Depths of Space: The Pioneer Planetary Probes (Joseph Henry Press, 2004), an essential resource for anyone tracking deep space research.
by Paul Gilster | Mar 21, 2006 | Communications and Navigation
Why is it so tricky to deliver large amounts of data from space? One key issue is frequency — because the amount of data that can be transmitted varies with the square of the frequency, higher frequencies give you more bang for the buck. Moving the Deep Space Network from today’s X-Band (between 8.40 and 8.45 GHz for deep space work and between 8.45 and 8.50 GHz for near-Earth operations) to the Ka-Band (31.80 to 32.30 GHz) will increase the network’s capabilities by a factor of four or five.
But the real goal is optical communications, where the far narrower signal carries a vastly increased amount of information. We need that kind of data-packing not only to get around spectrum-crowding as more and more spacecraft need to talk, but also to handle the high resolution imagery and video we’ll want to see from future deep space missions.
“It can take hours with the existing wireless radio frequency technology to get useful scientific information back from Mars to Earth. But an optical link can do that thousands of times faster,” said Karl Berggren, assistant professor in the Department of Electrical Engineering and Computer Science at MIT.
So news from Berggren’s team at MIT is heartening. Researchers there have developed a new light detector that can use optical links to surmount far slower radio technologies. The detector works at the same wavelength used by the optical fibers carrying broadband signals to homes and offices, and may eventually lead to startling results including color video from the far corners of the Solar System.
And just as critical, we’ll be able to move more and more of the high resolution imagery from missions like Mars Global Surveyor back to Earth. These are incredibly bulky datasets, including the results from observations made with synthetic aperture radar, terrain-mapping radar, and hyper-spectral imaging, and they gobble up plenty of precious bandwidth.
We need detectors like this one because spacecraft are starved for power. Using superconductor technology and nanowires, the MIT design is incredibly sensitive — working down to the level of a single photon — meaning it can receive signals from smaller lasers. The design is also speedy and efficient at light-gathering.
Such detectors are only one step, but they are markers of our progress on the road to an interplanetary infrastructure of laser installations that far surpasses conventional radio links. And that leads, ultimately, to the kind of optical network that will receive laser signals from our first generation of true interstellar probes.
For more, see Rosfjord, Yang, Berggren et al., “Nanowire single-photon detector with an integrated optical cavity and anti-reflection coating,” in Optics Express Vol. 14, Issue 2 (23 January 2006), pp. 527-534. A PDF is available here.
by Paul Gilster | Jan 20, 2006 | Communications and Navigation
All but lost in the recent news of the Stardust sample return and New Horizons launch, the Messenger spacecraft continues on its journey to Mercury. And significant science has already occurred, particularly the laser link set up across a record 24 million kilometers (15 million miles) between the spacecraft and Earth. Laser communications with spacecraft are still in their infancy, but this test showed the potential of moving past microwaves into far more effective laser channels.
The beauty of a laser signal is that it spreads much more slowly than conventional radio signals, a huge factor given the need to return significant data at maximum speed. Consider this: the Mars Pathfinder mission returned a radio signal that spread to hundreds of times the diameter of the Earth by the time it reached us. The 23-watt signal of the distant Voyagers broadcasts a beam now a thousand times the Earth’s diameter. These numbers play havoc with signal strength. Voyager’s signal to Earth during the Neptune encounter was twenty billion times less than the power needed to operate a digital wristwatch.
Now ponder that a probe circling Centauri A or B would return a radio signal with 1/81.000.000 the energy that Voyager returned from Neptune. No wonder we have to look to laser methods as we contemplate missions into nearby interstellar space. At that, the Messenger signals were primarily designed to test the spacecraft’s laser altimeter, but they offered a fine proof of concept. From 24 million kilometers, the laser pulses had expanded to roughly 1900 kilometers. That tighter signal will one day allow higher data rates, though it also demands refinements in the precision of spacecraft tracking.
A key paper for those interested in the application of lasers to interstellar communications is James Lesh, C. J. Ruggier, and R. J. Cesarone, “Space Communications Technologies for Interstellar Missions,” Journal of the British Interplanetary Society 49 (1996): 7-14. Also be aware of Alex Harwit, Martin Harwit, and Joss Bland-Hawthorn, “Laser Telemetry from Space,” Science 297, no. 5581 (July 26, 2002): 523, where the theoretical case for lasers is developed.
The Messenger tests are analyzed in David E. Smith, Maria T. Zuber et al., “Two-Way Laser Link over Interplanetary Distance,” Science Vol. 311 No. 5757 (January 2006), p. 53. The abstract is here.
by Paul Gilster | Oct 25, 2005 | Communications and Navigation |
One reason we need to re-think our communications strategies is that our resources are so limited. The Interplanetary Internet Project, for example, points out as a major justification for its work that if we can network spacecraft in distant planetary environments, we can sharply cut back on the amount of antenna time needed. After all, having a trio of spacecraft (including an orbiter, perhaps, and two rovers) linking their data to a single relay would mean a unified data download to Earth.
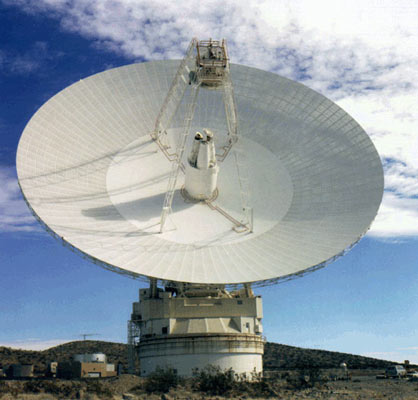
The IPN idea would create new networking protocols that could make these things happen. But research continues on other fronts, including the technology we use to transmit signals. An upcoming paper discusses phased arrays, wherein a large number of mini-transmitters could send a combined beam into the sky. Systems like these are familiar to those working with military radar but have been hitherto unavailable for cost reasons for civilian uses. The paper, by Louis Scheffer at Cadence Design Systems (San Jose, CA) discusses the advantages of such phased arrays with comparisons to digital cell phone technology and a consideration of the steep savings offered by saving transmission time over the Deep Space Network’s huge dishes.
Image: The Goldstone antenna, a 70-meter-diameter (230-foot) dish capable of tracking a spacecraft travelling more than 16 billion kilometers (10 billion miles) from Earth. Can we find ways to make the Deep Space Network’s antennae more efficient and cost-effective? Credit: NASA/JPL.
From an American Geophysical Union press release:
Currently, planetary radars and distant spacecraft communications need transmitters with extremely high power, which has been accomplished by combining a strong microwave source with a large reflective antenna. This is now done with giant satellite dishes mechanically steered to a point in the sky. NASA’s Goldstone radar, for example, the agency’s sensitive, deep-space analysis radar, uses a 500 kilowatt transmitter and a 70-meter [230-foot] reflector for tracking asteroids that may collide with Earth. The large antenna is focused on only a small point in space at a time, and must be adjusted–and occasionally shut down–due to changing weather conditions. In addition, Scheffer points out that while almost all of the world’s largest antennas are used to both send and receive, the powerful transmissions severely hinder their ability to detect faint signals from space.
What Scheffer has in mind as an alternative is a large, flat array of transmitters controlled by computers and capable of sending a powerful beam in one or several directions simultaneously. Scheffer’s system is transmit-only, and thereby useful for spacecraft control while freeing up existing antennae to operate in receive-only mode. It leverages the mass-production technologies now common to all kinds of electronics to arrive at the conclusion that an antenna similar to Goldstone’s could be built for a quarter of the cost. Existing work in phased radar arrays makes this conclusion plausible.
Centauri Dreams‘ note: Scheffer talks about a phased communications array being used for asteroid research as we scan the skies for near-Earth objects. But he’s also willing to speculate on the potential for such arrays when it comes to SETI signals sent by us to nearby stars. The technologies for producing such signals are rarely discussed in SETI literature, but have an obvious bearing on the likelihood of other civilizations deciding to create them in the first place (are we, as some have speculated, a galaxy full of listeners with no one in the transmission business?)
The paper is Scheffer, L. “A Scheme for a High-Power, Low-Cost Transmitter for Deep-Space Applications,” slated to appear in Radio Science in its October issue (Volume 40).
by Paul Gilster | Sep 28, 2005 | Communications and Navigation |
Celestial mechanics seems a long way from atomic physics, but new work by scientists and engineers suggests some remarkable parallels. In fact, the mathematics describing both have provided new designs for space missions, as witness the Genesis spacecraft, which returned particles from the solar wind to Earth. Genesis’ highly unstable orbit was controlled by the L1 Lagrange equilibrium point, a point between Earth and the Sun where the gravity of both bodies is balanced. The orbit is an example of a chaotic trajectory identical to those traversed on the atomic level by highly excited electrons.
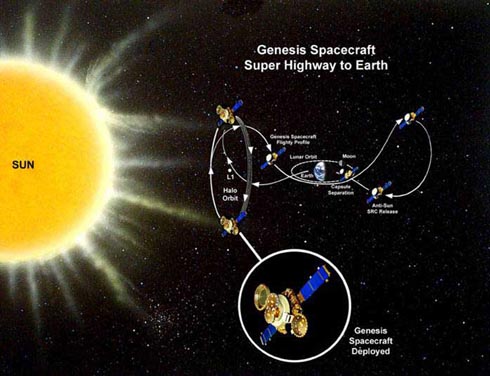
Image: The extraordinary orbit of the Genesis spacecraft, a lesson in controlling chaos. Credit: Jet Propulsion Laboratory.
The linkage between orbits and atoms is discussed in an article running in an article called “Ground Control to Niels Bohr: Exploring Outer Space with Atomic Physics,” running in the October 2005 issue of Notices of the American Mathematical Society. The article is also available online through the AMS Web site (free registration required). Authors Mason A. Porter and Predrag Cvitanovic write that the “…almost perfect parallel between the governing equations of atomic physics and celestial mechanics implies that the transport mechanism for these two situations is virtually identical…”
This is provocative stuff, but with such insights, engineers, mathematicians and physicists were able to set Genesis on a trajectory that met its misson goals with a minimal use of fuel. From the article:
On the celestial scale, transport takes a spacecraft from one Lagrange point to another until it reaches its desired destination. On the atomic scale, the same type of trajectory transports an electron initially trapped near the atom across an escape threshold (in chemical parlance, across a “transition state”), never to return. The orbits used to design space missions thus also determine the ionization rates of atoms and chemical-reaction rates of molecules!
Centauri Dreams’ take: In addition to providing new tools for mission designers, this work is valuable because, like spacecraft, comets and asteroids can also behave in a chaotic way, making an accurate determination of some orbits difficult. Creating a statistically valid description of near-Earth asteroids demands tools that can make sense of chaotic systems. Clearly, the crossover work occurring between astronomers, mathematicians and chemists can provide unexpected insights toward this goal.
by Paul Gilster | Jun 30, 2005 | Communications and Navigation |
Getting an interstellar probe to its target involves navigation of a high order. ‘Marker’ stars — stars that are both bright and distant enough to have relatively fixed positions for the duration of the journey — often show up in the scientific literature. Thus Rigel and Antares, both of which are far larger than the Sun, are attractive markers. Rigel (Beta Orionis) is some 800 light years distant, while Antares (Alpha Scorpii) is over 500 light years away. Are there other, better kinds of markers?
Perhaps so, according to the European Space agency. ESA, through its Ariadna initiative, is homing in on using pulsars for navigation. Ariadna operates under ESA’s Advanced Concepts Team to study new space technologies through linkages with the European academic community; it’s a way to strengthen the agency’s ties with independent researchers. As in the US, creating such connections is tricky business, but Ariadna is already doing interesting work, as its new study on pulsars suggests.
To be precise, the study, called “Feasibility study for a spacecraft navigation system relying on pulsar timing information,” relies on so-called ‘millisecond pulsars,’ those that spin at high rates of more than 40 revolutions per second. The beauty of millisecond pulsars is that they are old and quite regular in their rotation, so much so that their pulses can be used as extraordinarily accurate timing mechanisms. The fastest known millisecond pulsar is PSR 1937+211, which completes 642 revolutions every second.
Image: An artist’s impression of the millisecond pulsar PSR J1740-5340 (spinning at 274 times per second) and its companion star. The absorption of matter from an elderly red giant seems to have revitalized the spin rate of the pulsar, while its companion is being emptied of matter and turned into a white dwarf. Credit: European Space Agency.
We need to use marker objects because Earth-based navigation gets problematic for deep space probes. For one thing, when the Sun moves between Earth and the probe, no communication is possible. For another, navigation from Earth is feasible only along the line of sight to the craft. The kind of autonomous navigation systems that could avoid these issues would require a precisely understood natural reference frame, one that can be accomplished by millisecond pulsars. Autonomous systems developed to use such markers will be what we use on our first robotic probes to other star systems.
The trick, as the Ariadna report makes clear, will be to balance spacecraft mass and power constraints against the navigation hardware that will be developed. Pulsar signals may have an exquisite sense of timing, but they’re also very faint compared to conventional radio tracking signals. The study discusses suitable pulsar sources and derives the minimal hardware configurations that can be used to create such a positioning system. A possible catch: using several pulsar sources with different antennae seems necessary for the best accuracy, but this requires heavy hardware.
J. Sala, A. Urruela, X. Villares, et al., “Feasibility study for a spacecraft navigation system relying on pulsar timing information” has been available on the Net but seems to have been taken down. Until it’s back online, a mission analysis study of pulsar navigation (with link to the online file) can be found here. A backgrounder on Ariadna from ESA’s Advanced Concepts Team is also available. We’ll have much more to say about ACT and other advanced work there in coming days.