by Paul Gilster | Jan 22, 2010 | Asteroid and Comet Deflection |
I’m looking at the National Research Council’s final report on the detection of near-Earth objects, the culmination of the study that produced the NRC’s interim report last year. Let’s recall the context: It was in 2005 that Congress mandated that NASA find 90 percent of NEOs with a diameter of 140 meters or greater, such discovery to be concluded by 2020. The interim report, discussed in an earlier Centauri Dreams story, concluded that NASA couldn’t meet this goal because funds for the survey had never been appropriated.
Now we have a final report with suggestions on what NASA could do to finish the survey as soon as possible after the original 2020 deadline. Two possibilities emerge: A space-based telescope working in tandem with a ground-based telescope could finish the job the fastest. But if cost-cutting is necessary, the space option will have to be abandoned in favor of ground-based equipment. Gratifyingly, the NRC stands up strongly to defend Arecibo, whose role in asteroid observation is crucial, and also voices strong support for radar observations at the Goldstone Deep Space Communications Complex. From the report:
…the complementary radar systems at Arecibo and Goldstone are powerful facilities for characterization within their reach in the solar system, a maximum of about one-tenth of the Earth-Sun distance. Arecibo, which has a maximum sensitivity about 20-fold higher than Goldstone’s, but does not have nearly so good sky coverage as Goldstone, can for example, model the three-dimensional shapes of (generally very odd-shaped) asteroids, and estimate their surface characteristics, as well as determine whether the asteroid has a (smaller) satellite or satellites around it, all important to know for planning active defense. Radar can also accurately determine orbits of NEOs, from a few relatively closely spaced (in time) observations, which has the advantage of being able to quickly calm public fears (or possibly, in some cases, show that they are warranted).
Thus this recommendation:
Immediate action is required to ensure the continued operation of the Arecibo Observatory at a level sufficient to maintain and staff the radar facility. Additionally, NASA and NSF should support a vigorous program of radar observations of NEOs at Arecibo and NASA should support such a program at Goldstone for orbit determination and characterization of physical properties.
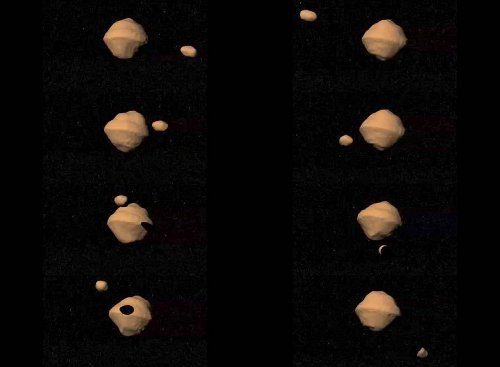
Image: Renderings of binary near-Earth asteroid 1999 KW4 showing its satellite making one orbit. The figure shows three-dimensional models in shaded relief, reconstructed from a set of radar images obtained at Arecibo and Goldstone in 2001. The models are shown in their proper orientation as viewed from Earth. Radar imaging has shown that ~15 percent of NEOs larger than 200 meters in diameter have one (or sometimes two) satellites. SOURCE: S.J. Ostro et al., Radar reconnaissance of near-Earth asteroids, pp. 143-150 in Near Earth Objects, Our Celestial Neighbors: Opportunity and Risk (A. Milani et al., eds.), Proceedings of the 236th Symposium of the International Astronomical Union, Prague, Czech Republic, August 14-18, 2006, Cambridge University Press, Copyright 2007 International Astronomical Union.
The asteroid or comet that struck the Yucatan some 65 million years ago is estimated to have been about ten kilometers in diameter, the kind of object that strikes the Earth only once every 100 million years on average. An object 140 meters in diameter, the size mandated by the survey requested of NASA, would cause regional damage, with impacts happening on average every 30,000 years, according to the report.
What to do if an NEO is discovered on a collision course with the Earth? The alternatives include using a ‘gravity tractor’ or other ‘slow push’ methods to move the object, but these are more practical for smaller objects (up to 100 meters in diameter) and would require decades of warning time. Changing a NEO’s orbit by flying a large spacecraft into it might also require warning times longer than we would necessarily have. Larger NEOs (with diameters greater than one kilometer) would demand the nuclear option:
…current technology allows us to deliver payloads for mitigation to NEOs in a wide range of orbits. However, in cases of short warning (under, say, a decade), payloads are likely to be severely limited in mass, but may often be sufficient to deliver a nuclear device. The development of the next generation of heavy-lift launch vehicles will considerably improve the situation. The development of advanced engines for in-space propulsion will considerably improve our capability for delivering rendezvous payloads (for characterization, to act as gravity tractors, or to emplace surface explosives) when the warning time is decades.
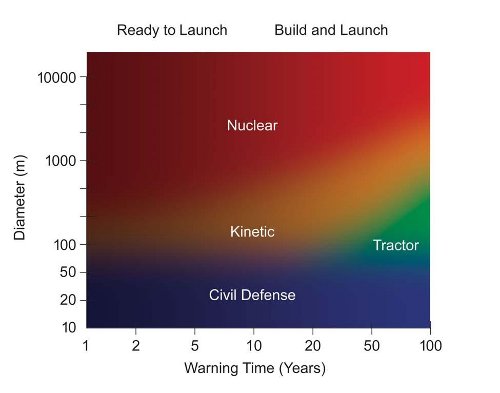
Image: Approximate outline of the regimes of primary applicability of the four types of mitigation (see report for the many caveats associated with this figure). Image Courtesy of Tim Warchocki.
The problem we face is that while these methods may work on paper, we’re not ready to put any of them to work on short notice. We also lack basic information about the kind of target we would be trying to deflect or destroy. Because of this, the report recommends a new program dedicated to more fully dealing with the hazards of NEOs:
The United States should initiate a peer-reviewed, targeted research program in the area of impact hazard and mitigation of NEOs. Because this is a policy driven, applied program, it should not be in competition with basic scientific research programs or funded from them. This research program should encompass three principal task areas: surveys, characterization, and mitigation. The scope should include analysis, simulation, and laboratory experiments. This research program does not include mitigation space experiments or tests which are treated elsewhere in this report.
We’re left, too, with the question of how small an NEO can be before we safely disregard it. The 1908 impact at Tunguska devastated more than 2000 square kilometers of forest. And while earlier estimates for the size of this object have been around 70 meters in diameter, there is recent research indicating the object could have been as small as 30 meters in diameter. That conclusion is, the report notes, preliminary, but because smaller objects are more numerous than larger ones, the likelihood of such an impact event is approximately once every three centuries. We also need to learn more about airbursts from impactors in this size range and whether they can cause tsunamis that damage coastlines.
The recommendation:
Because recent studies of meteor airbursts have suggested that near-Earth objects as small as 30 to 50 meters in diameter could be highly destructive, surveys should attempt to detect as many 30- to 50-meter objects as possible. This search for smaller-diameter objects should not be allowed to interfere with the survey for objects 140-meters in diameter or greater.
Space missions like NEAR Shoemaker and Hayabusa have given us information on two NEOs, and what we’ve learned is that Eros and Itokawa are dissimilar in many ways. From these missions and ground-based observations, we’ve learned that NEOs have a wide range of internal structures and more complex surfaces than had been realized. It’s clear that we need to learn more about these properties through further dedicated space missions if we’re to develop the strategies that will ensure we can move or destroy a NEO.
You can get a copy of the NRC report “Defending Planet Earth: Near-Earth Object Surveys and Hazard Mitigation Strategies” through the National Academies Press. It’s absorbing reading as we consider the problem of how to deal with a threat that may be exceedingly rare but potentially catastrophic. Our technology is only now getting to the point where we have the capability of affecting the trajectory of an incoming asteroid, depending upon its mass and the amount of advance warning we have. My belief is that a space-based infrastructure developing over the next century will come to play an essential role in safeguarding our planet.
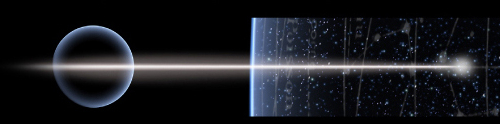
by Paul Gilster | Jan 21, 2010 | Missions |
If you haven’t read George Dyson’s fascinating history of Project Orion, let me recommend it to you highly. Project Orion: The True Story of the Atomic Spaceship (Henry Holt, 2002) fires the imagination with the audacity of the project, a nuclear pulse rocket that would have exploded atomic bombs behind the vehicle, using the world’s ultimate shock absorbers to ride the wave to the outer planets. There was talk of going to Saturn (to Enceladus, no less) in the late 1960’s, but those dreams were quickly quashed by treaties forbidding nuclear testing.
The Problem with Orion
Kelvin Long, who heads up the ambitious Project Icarus attempt to revisit and extend Project Daedalus, notes in a recent post on the Icarus blog that Freeman Dyson (George’s father) ultimately gave up on Orion (a fact that surprised me when I did a telephone interview with him on the prospects for interstellar propulsion back in 2003). Here’s what Dyson says about the subject in his book Disturbing the Universe (1979):
Sometimes I am asked by friends who shared the joys and sorrows of Orion whether I would revise the project if by some miracle the necessary funds were suddenly to become available. My answer is an emphatic no…. By its very nature, the Orion ship is a filthy creature and leaves its radioactive mess behind it wherever it goes…. Many things that were acceptable in 1958 are no longer acceptable today. My own standards have changed too. History has passed Orion by. There will be no going back.
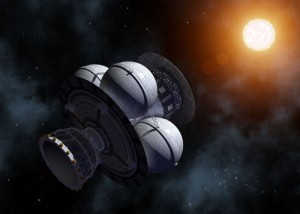
People still advocate concepts like Orion, but the Icarus team draws on the extensive work that has been done on pulsed fusion using a high intensity laser beam or an electron driver to produce detonation of fusion fuel pellets. This was the Daedalus approach in the 1970s, capitalizing not only on the research performed for Orion but also the intense scrutiny on pulsed micro-explosions that began in the early 1970s. The crucial fact is that this body of knowledge can be tapped to produce realistic performance assessments.
Image: Daedalus arrives at Barnard’s Star. Credit: Adrian Mann.
Fusion Alternatives and Their Uses
Long goes through various space propulsion systems in his post, noting two obvious categories. The first involves electric propulsion, which is power-limited; i.e., these methods can produce high specific impulse and high exhaust velocity, but at very low levels of thrust. The second covers both chemical and nuclear methods, which are energy-limited and can produce high thrust with high exhaust velocity, although at the cost of a short specific impulse. If we can develop fusion technologies sufficiently, we should be able to combine the advantages of both these approaches, getting more out of our fuel and increasing efficiency.
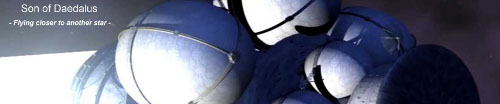
The fuel itself? Long talks about the same Deuterium/Helium-3 reaction that interested the Daedalus designers, although he notes the potential for Deuterium/Tritium. The latter involves the production of large amounts of neutrons and could therefore be problematic. On the other hand, we’re talking about huge demands on the civilization that develops D/He3 methods, too, as he goes on to note when discussing recent advances in fusion research:
The physics of fusion research has moved forward dramatically in recent years with the US National Ignition Facility now operational and others such as Laser MegaJoule in France under construction. Fast ignition proposals such as HiPER are also under consideration. The chances for scientists finally solving the ‘fusion problem’ are greatly increased. With this in mind, thinking about the implications to deep space missions is timely. It is quite possible that the demands of a fusion based drive will necessitate a sophisticated space based infrastructure for resource acquisition, processing, manufacture and construction. Especially if He3 mining of the gas giant Jupiter or even the Moon is considered. However, as a theoretical exercise in the application of science and engineering Project Icarus has a large amount of intrinsic worth.
Icarus and Competing Studies
We’ll see what the Project Icarus team comes up with (disclaimer: I am a consultant on the project, and obviously an advocate of Icarus). Given that Daedalus was a design study that changed the game, producing the first detailed investigation of what it would take to build an interstellar probe, it makes sense to build upon that work to re-evaluate its key assumptions and improve the design. It would be excellent to see such efforts paralleled by a study of an interstellar probe based on solar sail technology coupled with laser or microwave beam propulsion. Icarus is one attempt to expand our knowledge of interstellar engineering. It is not meant to rule out studies of competing concepts by others.
Ultimately [writes Long] the aim would be to improve the Technological Readiness Level for this sort of engine design type. If other teams used the same approach, and say built upon historical projects like Vista, Longshot, TAU or Starwisp it is a personal belief that the credibility of engineering designs for interstellar missions would be vastly improved. The historical link with both Orion and Daedalus also captured the hearts of the Icarus team and made for a strong support base upon which to galvanize both academic and public interest; a necessary condition to inspire people that this design study is worth doing. Although it is also true that after having questioned the original assumptions of Daedalus, the final Icarus design may look very different with technology not envisioned in the 1970s.
Indeed. Are hybrid designs possible? Long mentions antimatter-catalyzed fusion techniques (Penn State did early work on these possibilities), and that notion resonates given the relatively small amounts of antimatter needed and the potential for mining naturally occurring antimatter in the Solar System (James Bickford has studied the matter extensively — see this earlier Centauri Dreams story for more on his work). Whatever the case, just as the solar sail concept was refined through key papers in the 1980s, leading to our current readiness to deploy small sails for testing in space, fusion methods continue to be investigated in hopes of pushing our research into designs that will eventually be practical.
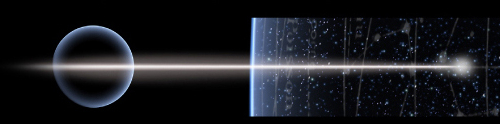
by Paul Gilster | Jan 20, 2010 | Research Tools |
The Exoplanetology site is developing a tool for those in need of quick exoplanet information. The Exoplanet Seeker is an interface that will make it easy to query various exoplanet databases, including the Extrasolar Planet Encyclopedia, NASA’s PlanetQuest New Worlds Atlas, the Exoplanet.org site and other sources like the Wikipedia and SIMBAD. Each of these sites has its own strengths, from light curves to graphical charts, so bringing them together will be helpful once early bugs in the interface (producing frequent failed queries) are resolved.
From tools on the Net to tools in space, it’s always interesting to speculate on what’s in the pipeline. Maybe ‘pipeline’ is too strong a word, though, because tools like the Transiting Exoplanet Survey Satellite (TESS) have to be approved by NASA, which is willing to consider an earlier version of the instrument it rejected but can offer no promise of success. Nonetheless, the results from CoRoT and the early detections of Kepler (not to mention the possibilities of WISE in finding nearby brown dwarfs) keep space instrumentation in sharp focus.
We’ve looked at TESS before, noting that its six wide-angle lenses would be put to use flagging stars with planets, building a list that the James Webb Space Telescope (to be launched in 2014) would then study in greater detail. What’s interesting about TESS, as this short article in Popular Science points out, is that it would scan a chunk of sky some 400 times larger than Kepler. That involves 2.5 million of the closest stars, a search that should return an abundance of interesting worlds to study.
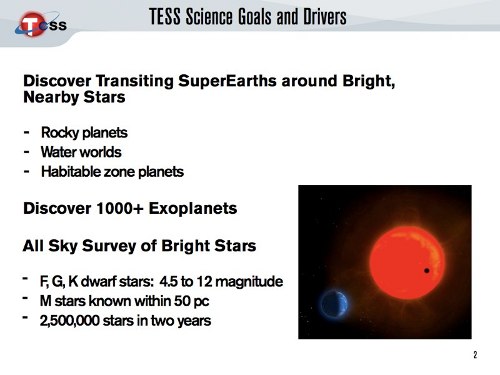
Image credit: MIT.
The thinking of the TESS team, according to this MIT news release, is that between 1600 and 2700 planets might be found in the first two years of observations, including between 100 and 300 small, rocky worlds. The light curves produced by the planetary transits TESS observes would then be passed along to observatories on the ground and slated for spectroscopic analysis by the Webb instrument. The latter could potentially reveal details about planetary atmospheres and their constituents. Expect a new TESS proposal to be submitted this year to follow up an earlier initial feasibility study.
Meanwhile, we continue to watch the development of the Webb telescope with great interest. Mark Clampin (NASA GSFC), discusses the possibilities for planetary spectroscopy in an instrument originally designed to search for the earliest galaxies. JWST will be able to extract a lot of information from a planetary transit:
“By comparing the two spectra for the star (in and out of transit), we can extract the planet’s spectrum and learn about the planet’s atmosphere. We have to collect a lot of infrared light — a billion or more photons — for each spectral element to isolate features. Webb is perfect for this kind of study.”
TESS could be quite useful in feeding targets to JWST, but the latter will also be doing planet-hunting of its own using coronagraphic techniques that block the light of stars so planets around them are more readily visible. Clampin continues:
“Webb will show us what the ‘exoplanet zoo’ looks like. This telescope will be very good at observing and taking spectra of gas giant planets, and we can take some spectral data on smaller planets, too, about Neptune-sized. Our telescope will also zoom in to study newly discovered super Earths — planets bigger than Earth but smaller than Neptune.”
Exciting times ahead as we contemplate our next steps in space-based observation, especially the deployment of JWST’s 25 m2 collecting area. Will JWST be able to tell us whether life exists on distant terrestrial worlds? Probably not, though Clampin isn’t above hedging his bets:
“A true Earth twin would be too small to emit enough infrared light from its atmosphere for Webb to pick up. Still, every time scientists make statements like that, someone proves them wrong. Transit science is changing so fast, it’s hard to say exactly what wonders Webb’s hunt will turn up.”
A recent NASA article on JWST is here.
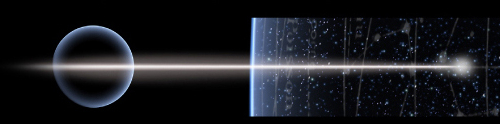
by Paul Gilster | Jan 19, 2010 | Exoplanetary Science |
Over twenty percent of the planets we’ve found around other stars inhabit binary systems. It’s intriguing to take a close look at these. Most of the planet-bearing binaries are what is known as ‘wide S-types,’ meaning that the companion star orbits the inner star/planet system at a distance of over 100 AU. But take a good look at GJ86b, γ Cephei b and HD41004b. Here we’re looking at three planets in close binary systems with a separation between the component stars of 20 AU or less. That separation raises the eyebrows, for Alpha Centauri A and B form a close binary with a semimajor axis of 23.4 AU.
We have three ongoing planet hunts around the Centauri stars, Debra Fischer’s work being matched by Michel Mayor’s team at La Silla and both complemented by a new search based at Mt. John Observatory in New Zealand. So it may not be long — months, possibly — before we have some word about planets around these stars. Informing all these searches, though, is the issue of gravitational perturbations caused by the proximity of the two stars. Various simulations have shown that planets of Earth mass can exist around stars like this, but recent work has questioned whether planetary embryos can form and remain stable in the first place, a question tackled in a new paper.
Astronomers Ji-Wei Xie, Jian Ge (University of Florida) and Ji-Lin Zhou (Nanjing University, China) have created a series of simulations to estimate how long it takes planetesimals embedded in a protoplanetary disk to accrete into planetary embryos. This is rapidly becoming the key issue. What we know so far is that the mass upper limit of a planet in this system is 2.5 Jupiter masses around Centauri A and 3.5 Jupiter masses around Centauri B. That leaves us with the possibility of lower-mass planets if this system will allow planetary embryos to form. The problem is that most simulations have assumed a disk of such embryos as the starting point and have followed planet development from that point.
From the paper:
…the remaining question is whether these embryos can form. Thébault at al. (2009) recently addressed this problem by analyzing the conditions for planetesimal accretion. They conclude planetary embryos formation through planetesimal accretion seems impossible around α CenB, unless the binary separation was wider in its initial stages. However, their conclusions are limited in the absolutely coplanar case, where the inclination between the gas disk and binary stellar orbit is exactly equal to zero…
Ji-Wei Xie and team reopen the case by extending Thébault’s work to include the effect of binary inclinations. They develop a new model tested through simulation to study whether the zone from 0.5 to 2.5 AU around Centauri B may allow planets to form. The simulations vary the gas-disk density as well as the binary inclination and work with variables like planetesimal mass distribution, impact rate and the fraction of accreting collisions to come up with a conclusion: Planetesimal accretion into planetary embryos takes significantly longer in this binary environment than around single stars, which does not favor the formation of gas giant planets, but the formation of smaller, terrestrial worlds is possible.
Let me quote the concluding paragraph on this:
Our results support recent work by Guedes et al. (2008), which has shown Earth-mass planets can be formed near the habitable zone (0.5-0.9 AU) of α CenB if the disk is initially composed of lunar-mass planetary embryos. The possible accretion zone shown in this paper is roughly between 1-2 AU, which matches well with their planet formation zone (~0.5-2.0 AU)… In addition, at the time of writing this paper, we note a promising result from Payne et al. (2009) that Earth-like planets can also form in the habitable zone of α CenB-like binary systems through outward migration from the inner accretion-unperturbed zone (within ~ 0.7 AU). Therefore, by combining these studies…and our simulations, it is quite possible that a habitable Earth-like planet may be hidden around α CenB.
That’s good news for planet hunters, of course, and the happy fact is that we should be getting solid data to measure these simulations against in the not distant future. The paper is Ji-Wei Xie et al., “Planetesimal Accretion in Binary Systems: Could Planets Form Around α Centauri B?” Astrophysical Journal 708, pp. 1566-1578 (abstract / preprint). For a discussion of Thébault’s work, see this earlier Centauri Dreams story.
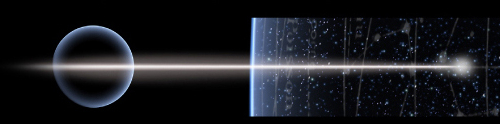
by Paul Gilster | Jan 18, 2010 | Outer Solar System |
What goes on under the clouds of Neptune and Uranus? A new paper reinforces the possibility that there are oceans of liquid diamond in such places, diamond seas with solid diamond icebergs. It’s a notion with a pedigree. I’m looking, for example, at a paper by David Stevenson (Caltech) from the Journal de Physique from November of 1984, where I find this:
There is clear evidence that many hydrocarbons decompose (or collapse) upon shock compression, probably into graphite and hydrogen. It is very important to establish the range of temperature and C:H ratios for which this decomposition can occur. It is equally important to establish whether an actual phase separation occurs (implying possible formation of a diamond or liquid metallic (?) carbon layer in Uranus and Neptune) or whether a collapsed but intimately mixed C-H structure results.
Stevenson’s work built on that of Marvin Ross, who suggested the possibility of diamonds in this environment in 1981. Researchers at the University of California at Berkeley demonstrated about ten years ago that the high temperatures and pressures found inside planets like these can turn methane into diamond, and in fact that the diamonds settling into Neptune’s core could account for the excess heat radiated by the planet. Now a team led by Jon Eggert (Lawrence Livermore National Laboratory) has explored the conditions under which diamonds melt, and has shown that, like water, liquid diamond when freezing and melting produces solid forms atop liquid ones.
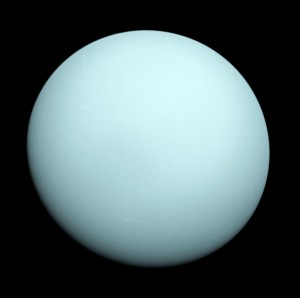
To melt diamond requires high pressures, which has made measuring its melting point extremely difficult. But the pressures found inside gas giants, and the high temperatures that go with them, should be enough to do the job. This Discovery News story notes that Eggert’s team subjected a small diamond (about a tenth of a carat) to a laser beam, liquifying the diamond at pressures some 40 million times greater than at sea level on Earth. As they then reduced the temperature and pressure, solid chunks of diamond began to appear at about 11 million times sea level pressure with a temperature of some 50,000 Kelvin.
Image: Voyager’s view of Uranus. Does an ocean of diamond lurk beneath these clouds? Credit: NASA/JPL.
The diamond chunks, like tiny icebergs, did not sink but floated. Eggert believes an ocean of liquid diamond could explain the fact that, unlike the relatively close match we find on Earth, the magnetic and geographical poles on Uranus and Neptune do not align, and can be offset by as much as 60 degrees from the north-south axis. Put an ocean of liquid diamond in just the right place and the magnetic field displacement makes more sense. We won’t know for sure without further study, but Eggert’s work makes the prospects of diamond oceans more plausible, reinvigorating what may be the most exotic scenario in the Solar System, one that could extend to other gas giants and even to the interiors of brown dwarfs.
The paper is Eggert et al., “Melting temperature of diamond at ultrahigh pressure,” Nature Physics 6 (1 January 2010), pp. 40-43 (abstract). The Ross paper is “The ice layer in Uranus and Neptune — diamonds in the sky?” Nature 292 (30 July, 1981), 435 – 436 (abstract). Stevenson’s paper is “High pressure physics and chemistry in giant planets and their satellites,” Journal de Physique Vol. 45 (November, 1984), pp. C8-97 to C8-103 (abstract).
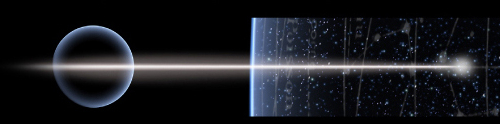