Yesterday's look at organic compounds on Comet 67P/Churyumov-Gerasimenko needs to be augmented today by a just released study of the comet with implications for how all comets evolve. But first, a renewed pointer to the Kickstarter campaign for KIC 8462852, the unusual star whose light curves continue to baffle astronomers. Please consider contributing to the project, which would raise enough money ($100,000) to support a year of observations. We're about halfway through the campaign but not yet at the halfway point in funds. Have a look at the information provided on the Kickstarter page, or in my essay A Kickstarter Campaign for KIC 8462852, which also has the relevant links. We know the light curves of 'Tabby's Star' are not periodic, so we need continuous monitoring to gain more data on what may be happening there. If we can raise the funds, the Las Cumbres Observatory Global Telescope Network, already supporting the project, can give us the multi-wavelength observations we need....
Charter
In Centauri Dreams, Paul Gilster looks at peer-reviewed research on deep space exploration, with an eye toward interstellar possibilities. For many years this site coordinated its efforts with the Tau Zero Foundation. It now serves as an independent forum for deep space news and ideas. In the logo above, the leftmost star is Alpha Centauri, a triple system closer than any other star, and a primary target for early interstellar probes. To its right is Beta Centauri (not a part of the Alpha Centauri system), with Beta, Gamma, Delta and Epsilon Crucis, stars in the Southern Cross, visible at the far right (image courtesy of Marco Lorenzi).
Now Reading
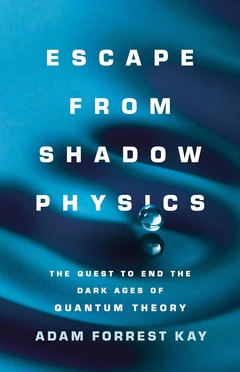
Recent Posts
- An Incoming ‘Stream’ from Alpha Centauri?
- SETI: Learning from TRAPPIST-1
- A Gravitational Wave Surprise
- Catches, Comets and Europa
- Go Clipper
- Is Dark Energy Truly a Constant?
- Planetary Defense: Good News from the Taurids
- Advancing Space Technology and Preparing for Contact with Extraterrestrial Intelligence through Multilateralism
On Comments
If you'd like to submit a comment for possible publication on Centauri Dreams, I will be glad to consider it. The primary criterion is that comments contribute meaningfully to the debate. Among other criteria for selection: Comments must be on topic, directly related to the post in question, must use appropriate language, and must not be abusive to others. Civility counts. In addition, a valid email address is required for a comment to be considered. Centauri Dreams is emphatically not a soapbox for political or religious views submitted by individuals or organizations. A long form of the policy can be viewed on the Administrative page. The short form is this: If your comment is not on topic and respectful to others, I'm probably not going to run it.
Follow by E-Mail
Advanced Propulsion Research
Exoplanet Projects (Earth)
- AFOE
- Amateur Exoplanet Archive
- Anglo-Australian Planet Search
- APACHE Project
- ASTEP: Antarctic Search for Transiting Extrasolar Planets
- ASTRA
- Astro Gregas
- Atacama Large Millimetre Array
- Automated Planet Finder
- Berlin Exoplanet Search Telescope
- California & Carnegie Planet Search
- Carl Sagan Institute (Cornell)
- CARMENES
- Carnegie Astrometric Planet Search
- CBA Belgium Observatory
- CHIRON
- CLEVER Planets
- CODEX
- Colossus
- Coralie
- DayNight
- DEMONEX (DEdicated MONitor of EXotransits)
- Dispersed Matter Planet Project
- East Asian Planet Search Network
- Elodie
- ESO Coude Echelle Spectrometer
- ESPRESSO (Echelle SPectrograph for Rocky Exoplanet and Stable Spectroscopic Observations)
- European Extremely Large Telescope
- Evryscope
- Exoplanet Tracker
- Externally Dispersed Interferometry
- Fabra-ROA
- GAPS (Global Architecture of Planetary Systems)
- Gemini Planet Imager
- GEMSS: Global Exoplanet M-dwarf Search-Survey
- Geneva Extrasolar Planet Search
- Habitable Zone Planet Finder
- HARPS North
- HARPS-N
- HATNet Exoplanet Survey
- High Accuracy Radial velocity Planetary Search
- Hobby-Eberly Telescope
- Italian Search for Extraterrestrial Life
- ITASEL
- Keck Interferometer
- Keck Planet Finder
- KELT North
- KELT South
- KMTNet (Korea Microlensing Telescope Network)
- KOBE: K-dwarfs Orbited By habitable Exoplanets
- Large Binocular Telescope
- Las Cumbres Global Telescope Network
- Low Frequency Array
- LYOT Project
- MACHO
- Magdalena Ridge Optical Interferometer
- Magellan Telescope
- MARVELS
- MARVELS (Multi-object APO Radial Velocity Exoplanet Large-area Survey)
- MASCARA
- Maunakea Spectroscopic Explorer
- McDonald Observatory
- MEarth
- METIS (Mid-Infrared E-ELT Imager and Spectrograph)
- MicroFUN Microlensing Follow-Up Network
- Microlensing Planet Search Project
- MINERVA (MINiature Exoplanet Radial Velocity Array)
- MOA
- MONET
- N2K
- Nancay Decametric Search
- NEAR
- NEID Spectrograph
- New Mexico Exoplanet Spectroscopic Survey Instrument
- NGTS (Next-Generation Transit Survey)
- NIRPS (Near Infrared Planet Searcher)
- Okayama Planet Search Program
- Optical Gravitational Lensing Experiment
- OWL
- PARAS (PRL Advanced Radial-velocity Allsky Search)
- Permanent All Sky Survey
- PHASES
- PIRATE (Physics Innovations Robotic Astronomical Telescope Explorer)
- PISCES (Planets in Stellar Clusters Extensive Search)
- PLANET
- PLANETS
- Precision Radial Velocity Spectrometer
- PRIMA-DDL
- Project 1640
- Pulsar Planet Detection
- QES (Qatar Exoplanet Survey)
- Radio Interferometric Planet Search
- RoboNet (Microlensing)
- SAINT-EX
- Search for Trojan Extrasolar Planets
- SEEDS (Subaru Strategic Exploration of Exoplanets and Disks)
- SHINE
- Solaris
- Sophie
- Spectrashift
- SPECULOOS
- SPHERE
- SPOTS: (Search for Planets Orbiting Two Stars
- Square Kilometer Array
- STARE
- STELLA
- SuperWASP
- Systemic
- Tennessee Automatic Photoelectric Telescope
- TEP
- Thirty Meter Telescope
- TransitSearch
- Transitsearch
- TRAPPIST (TRAnsiting Planets and PlanetesImals Small Telescope)
- TrES: The Transatlantic Exoplanet Survey
- TRESCA Project
- United Kingdom Infrared Telescope
- University of St. Andrews Planet Search
- UNSWEPS Project
- UVES
- Very Large Telescope Interferometer
- VIDA
- WASP (Wide Angle Search for Planets)
- WHAT
- XO Project
Exoplanet Projects (Space)
- ACEsat
- Aragoscope
- ARIEL: (Atmospheric Remote-Sensing Infrared Exoplanet Large-survey)
- ASTERIA
- Astro-1
- ATLAST (Advanced Technology Large-Aperture Space Telescope)
- CHEOPS – CHaracterising ExOPlanet Satellite
- CoRoT
- CubeSat
- Darwin
- Dual Use Exoplanet Telescope
- ECHO (Exoplanet Characterization Observatory)
- Eddington
- EPOXI (Extrasolar Planet Observation and Deep Impact Extended Investigation)
- Euclid
- EXCEDE
- ExoplanetSat CubeSat
- FINESSE
- Gaia
- GEST
- HabEx
- HEK (Hunt for Exomoons with Kepler)
- High Étendue Multiple Object Spectrographic Telescope (THE MOST)
- High-Definition Space Telescope
- HST Astrometry
- James Webb Space Telescope
- Kepler
- Kilometer Space Telescope
- Laser Interferometer Space Antenna
- LISE Hypertelescope
- LUVOIR
- MOST (Microvariability and Oscillations of STars)
- Nancy Grace Roman Space Telescope
- NEAT
- New Worlds Imager
- Origins Billion Star Survey
- Origins Space Telescope
- Pegase
- Planet Imaging Concept Testbed
- Plato
- PlaVi (PlanetVision)
- Project Blue
- SISTINE
- Space Interferometry Mission
- SPICES (Spectro-Polarimetric Imaging and Characterization of Exo-planetary Systems)
- Spitzer Space Telescope
- SUPER-SHARP
- SWEEPS
- Terrestrial Planet Finder
- TESS (Transiting Exoplanet Survey Satellite)
- TOLIMAN
- Twinkle
- UMBRAS
Further Astronomical and Astronautical Resources
- 100 Year Starship
- Acta Astronautica
- ADS Abstract Service
- Alternative Earths Astrobiology Center
- American Astronomical Society
- American Geophysical Union
- American Institute of Aeronautics and Astronautics
- astro-ph preprint server
- AstroArt of David A. Hardy
- AstroBetter
- Astrobiology Magazine
- Astrobites
- Astrometry.net
- Astronautics Now
- Astronomical Journal
- Astronomy & Astrophysics
- Astronomy Picture of the Day
- Astrophysical Journal
- Beyond NERVA
- British Interplanetary Society
- Bulletin of the American Astronomical Society
- Cosmic Ancestry
- Division for Planetary Sciences
- European Federation of Biophysics
- Event Horizon Telescope
- Exoplanet Transit Database
- Exploring the Universe with Andrew Fraknoi
- Extrasolar Planets and Astrobiology
- Extrasolar Planets Encyclopedia
- Galaxy Forum
- Galileo Project
- Google Scholar
- Icarus Interstellar
- Institute for Interstellar Studies
- Interstellar Journey
- Interstellar Research Centre
- Interstellar Studies Bibliography
- James Benford
- L’Institut de l’Information Scientifique et Technique
- Lunar and Planetary Institute
- Meteoritics and Planetary Science
- NASA Technical Reports Server
- Nature
- Orbital Index
- Orbital Index
- Overview Institute
- Physics
- Planetary and Life Science Community Meetings Calendar
- Planetary and Space Science
- Principium (Journal of I4IS)
- ResearchGATE
- RocketSTEM
- Science
- Scitizen
- SDSS SkyServer
- SETI News
- SFSU Exoplanet Group
- SIMBAD Astronomical Database
- Space Agenda
- Space Sailing
- Space Telescope Science Institute
- Space Transport and Engineering Methods
- spaceweather.com
- The neighborhood
- Trans-Neptunian Automated Occultation Survey
- Troy Project
Weblogs, Discussions, Commentaries
- Adam Crowl (Crowlspace)
- Airminded
- Alien Life
- Ancient Solar System
- Antimatter
- Apparent Brightness
- AstroBlog
- AstroEngine.com
- Astrogator's Logs (Athena Andreadis)
- Astronautical Evolution
- Astronomist
- Astronomy Blog
- Astronomy.com Blog
- astroPT
- Astroquizzical
- Asymptotia
- Atlas of the Universe
- B612 Foundation
- Bad Astronomy
- Beyond Earthly Skies
- Beyond Impossible
- Billion Year Plan
- Buran Space Shuttle
- Captain Interstellar (Paul Titze)
- Celestial Matters
- Cheap Astronomy
- Cocktail Party Physics
- collectSPACE
- Colony Worlds
- Comets & Asteroids: Small Bodies of the Solar System
- Cosmic Diary
- Cosmic Mirror
- Cosmic Tusk
- Cosmic Variance
- Cosmic Visions
- CosmoCoffee
- Cumbrian Sky
- Dad2059
- Deep Sky Blog
- Dialogos of Eide
- Dick’s Rocket Dungeon
- Dragon's Gaze
- Dream of the Open Channel
- Dreams of Space – Books and Ephemera
- Dreams of Space: Books and Ephemera
- Drew Ex Machina (Andrew LePage)
- DSFP's Spaceflight History Blog
- Dynamics of Cats
- Eternos Aprendizes
- Eureka
- Eureka (Daniel Marín)
- Ex Space
- ExoClimes.com
- Exoplanetology
- Exoplanets Channel
- Extrasolar Visions II
- Final Frontier
- Finding Pluto
- Flank Speed
- Fly Me to the Moon
- Fraknoi's Universe
- Future & Cosmos
- Future Incredible
- Future Planetary Exploration
- Futurismic
- Galactic Journey
- Gregory Benford
- Habitable Worlds
- Habitable Zone
- Hop's Blog
- Il Tredicesimo Cavaliere
- In the Dark (Peter Coles)
- Innovation Watch
- Innumerable Worlds
- Invitation to ETI
- Isaac Arthur (videos)
- James Essig
- James Randi Educational Foundation Forum
- Jatan's Space
- John Cleary Creations
- Jon Lomberg
- Kentucky Space
- Know the Cosmos
- Last Word on Nothing
- Laurel's Pluto Blog
- Leonard David's Inside Outer Space
- Letters to Nature
- Lifeboat Foundation
- Lone Mind
- Long Bets Foundation
- Long Now Foundation
- Lost in Transits
- Magellan AO
- Many Worlds (Marc Kaufman)
- Martian Chronicles
- Meridiani Journal
- Music of the Spheres
- Nano Age
- NASA Watch
- NASA-UC Eta-Earth Survey
- New Papyrus
- Next Big Future
- NGTS (Next-Generation Transit Survey)
- On the Path to Space
- One-Minute Astronomer
- OrbitalHub
- Orion's Arm
- Our Universe in 202 Notations
- Out of the Cradle
- Overcoming Bias (Robin Hanson)
- Patrick McCray
- peregrinus interstellar
- PHASES (Palomar High-precision Astrometric Search for Exoplanet Systems)
- Physics arXiv Blog
- PI Club
- Planet/Planet
- PLANETPLANET (Sean Raymond)
- Polymath (Robert Clark)
- Posthuman Blues
- Potentia Tenebras Repellendi
- Profiles of Our Future in Space
- Project Icarus Weblog
- Project Rho (Winchell Chung)
- Quasar9
- Real Science
- Remote Central
- Rick Costello Space Art
- Riding with Robots
- Robot Explorers
- Robot Guy
- Rymden i Dag
- Science Meets Fiction
- Science News
- SciTech Journal
- Scitizen
- Simostronomy
- Singularity Institute
- Slacker Astronomy
- SolStation
- Sorting Out Science
- Space Archaeology
- Space Elevator Blog
- Space FTW
- Space Law Probe
- Space Pragmatism
- Space Review
- Space Transport News
- Space Travel Blog UT Tartu Observatory
- Spaceflight History
- Spacewriter’s Ramblings
- Stan Erickson's Alien Civilization Blog
- Star Bright?
- Star Stryder
- Starts with a Bang
- Strange Paths
- Sufficiently Advanced
- Supernova Condensate
- This Is Rocket Science
- This Week’s Finds in Mathematical Physics
- Tiny Mantras
- Titan Exploration
- Tom Barclay/Planet Hunter
- Tomorrow Is Here
- Trevor Paglen
- Ultratech Memes
- Universe Today
- Unmanned Spaceflight
- Velcro City Tourist Board
- Visions 2200
- Visual Astronomy
- Visualizing Science
- Wanderingspace
- Watered Down Physics
- Where's The Flux (Tabby's Star)
- Will Gater
- Woodward Effect
- Worlds of David Darling
- Wow! Signal Podcast
- Written Worlds