by Paul Gilster | Jun 22, 2017 | Missions |
Back in the 1970s, the British Interplanetary Society conceived the idea of designing a starship. The notion grew into Project Daedalus, often discussed in these pages, producing a final report that summed up what was then known about interstellar possibilities, from fusion propulsion to destination stars. Barnard’s Star, 6 light years out, became the target because at the time, it was the only star for which evidence of planets existed, though that evidence later turned out to be the result of error in the instrument being used for the observations (more on this soon, in an essay I’ve written for the Red Dots campaign. I’ll link to it as soon as it runs).
The designing of Daedalus, much of it done in London pubs, was a highly significant event. What Alan Bond, Anthony Martin, Bob Parkinson and the rest were doing was not so much putting forth something that our civilization would build as sending us a clear message. Even at this stage of our development, humans could conceive of ways to reach the stars. There is nothing in the laws of physics that prevents it. What, then, is possible in another fifty years? In a hundred? Even now, the Daedalus concept is being reimagined by Project Icarus.
I think of successive iterations in starship concepts, periodically working through the puzzles in the light of improving technologies. This is why the TU Delft Starship Team (DSTART) has caught my eye. TU Delft is the Delft University of Technology (Technische Universiteit Delft) located in a town known for its canals to the north of Rotterdam. Angelo Vermeulen, now a doctoral candidate in the systems engineering section here, is the founder of DSTART, a collection of students and researchers collaborating on an evolving starship concept.
But Vermeulen deserves further introduction. As well as being a space systems researcher, he is a biologist and artist, and serves as what TU Delft calls a ‘community architect’ in its Participatory Systems Initiative, a determinedly multidisciplinary effort. Back in 2009, Vermeulen created Space Ecologies Art and Design (SEAD), which is an international network developing projects interrelating ecology, technology and community. He has also worked with ESA and NASA, serving as crew commander of the latter’s HI-SEAS Mars simulation in Hawaii. He has held faculty positions ranging from the University of Applied Arts in Vienna to the Philippines Open University in Los Baños. He became a TED Senior Fellow in 2013.
The idea this polymathic researcher is promoting is not to develop actual hardware but to firm up a vision of deep space exploration, one which, says Vermeulen, “…unite[s] the biological, technological and social dimensions. And it is about starships that evolve during their journey.”
This is a multidisciplinary effort drawing on astrophysics, biotechnology, computer simulation, chemistry and art. It should bring to mind Rachel Armstrong’s work on worldships, analyzed in a series of essays in her book Star Ark: A Living, Self-Sustaining Spaceship (Springer, 2017). And in fact Vermeulen was one of the authors of an essay in that volume called “The World in One Small Habitat,” in a section entitled ‘Space Architectures.’
Just how a starship could evolve on journeys taking decades and perhaps longer is fodder for DSTART’s preliminary analysis, which includes near-term in-system technologies like asteroid mining and exploitation, 3D printing and studies in closed loop life support of the kind the European Space Agency is analyzing in a project called MELiSSA. The acronym here unpacks to Micro-Ecological Life Support System Alternative, defined by ESA this way:
… the recovering of food, water and oxygen from organic waste carbon dioxide and minerals, using light as source of energy to promote biological photosynthesis. It is an assembly of processes (mechanical grinding, bioreactors, filtration, wet oxidation, etc.) aiming at a total conversion of the organic wastes and CO2 to oxygen, water and food.
It is based on the principle of an “aquatic” lake ecosystem where waste products are processed using the metabolism of plants and algae which in return provide food, air revitalization and water purification.
“The World in One Small Habitat” looks at MELiSSA in the context of other projects that are exploring closed loop life support. Mastering the huge problems of sustainable ecologies is key to getting humans deep into the Solar System and beyond.
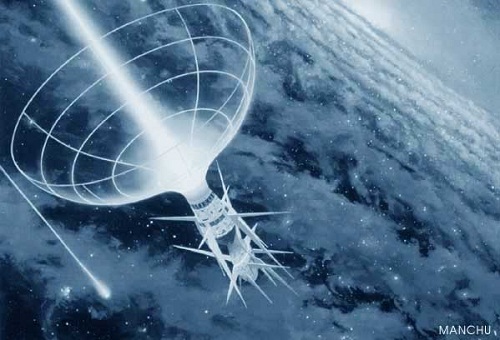
Image: A Bussard ramjet in flight, as imagined for ESA’s Innovative Technologies from Science Fiction project. Credit: ESA/Manchu.
Centauri Dreams has many readers in The Netherlands and nearby. If you’re anywhere in range on Monday June 26, DSTART will be holding a mini-symposium to share its recent work.
PROGRAM
16:00: Evolving asteroid starships, Angelo Vermeulen
16:20: Why interstellar exploration, Jimmy Verkooijen
16:35: Modular starship architecture, Francisco Muñoz Correa
16:55: Modeling a regenerative ecosystem, Alvaro Papic
17:15: Modeling asteroid mining and architectural evolution, Andreas Theys
17:35: The use of VR for interactive starship design, Anton Dobrevski
17:50: Concluding remarks, Angelo Vermeulen
18:00: Drinks
WHEN
Monday June 26, 4-6pm
WHERE
Room H (Wing A), Faculty TPM, TU Delft
Jaffalaan 5, 2628 BX Delft
FOR WHOM
Everyone is welcome. Feel free to bring guests.
Places are limited.
Efforts like these encourage me because they point to increasing interest in the great themes of interstellar exploration. And it’s always heartening to see active university groups tackling these matters — Drexel University’s energetic Icarus Interstellar chapter also comes to mind.
Interstellar crossings are well beyond our capabilities at present, at least at time frames we find acceptable. But we can still come together to analyze the issues and take early steps toward their solution, helping to define problems and clarify research needs. Ad astra incrementis means exactly this: No single breakthrough but steady and progressive steps. Not that we wouldn’t all welcome breakthroughs, but the point is that persistence and hard work are what it takes to move the ball forward even as we remain open to the unexpected.
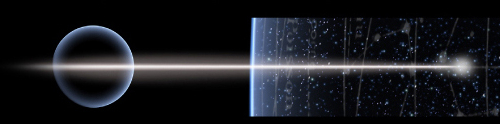
by Paul Gilster | Jun 21, 2017 | Exoplanetary Science |
The European Space Agency has just announced the official adoption of the PLATO mission. The untangled acronym — PLAnetary Transits and Oscillations of stars — tells us that, like Kepler and CoRoT, this is a planet hunting mission with asteroseismological implications. Photometric monitoring of nearby bright stars for planetary transits and determination of planetary radii should help build our target list for spectroscopic follow-up as we delve into planetary atmospheres looking for biosignatures. Launch is scheduled for 2026.
Asteroseismology studies how stars oscillate, giving us information about the internal structure of the star that would not be available through properties like brightness and surface temperature. PLATO will be carrying out high precision photometric monitoring at visible wavelengths, targeting bright stars (mV ? 11), though with capabilities for fainter stars down to magnitude 16. Several hundred thousand stars will ultimately be characterized in the search for planets around G-class stars like the Sun, subgiants and red dwarf stars.
Keep in mind one important difference between PLATO and Kepler. The latter worked with a starfield that was in most cases quite faint, down to magnitude 17. No one can argue with the success of Kepler and its recently released final catalog, but the faintness of many of its stars meant that many planetary candidates have proven difficult to follow up and confirm. PLATO’s wide field will allow monitoring of the brightest stars, which should allow smaller planets to be followed up by ground-based instruments and ultimately confirmed.
“Using observations of stellar vibrations, PLATO will for the first time fully characterize these stars and their planets with regard to mass, radius, and age”, says Prof. Dr. Laurent Gizon, director of the Max Planck Institute for Solar System Research and head of the PLATO Data Center. “This will revolutionize the study of the evolution of exoplanets and their host stars… With today’s adoption, the implementation – the actual building and construction of the spacecraft and its instruments – can begin. In parallel, the design of the software to analyse the observations will be developed at the PLATO Data Center.”
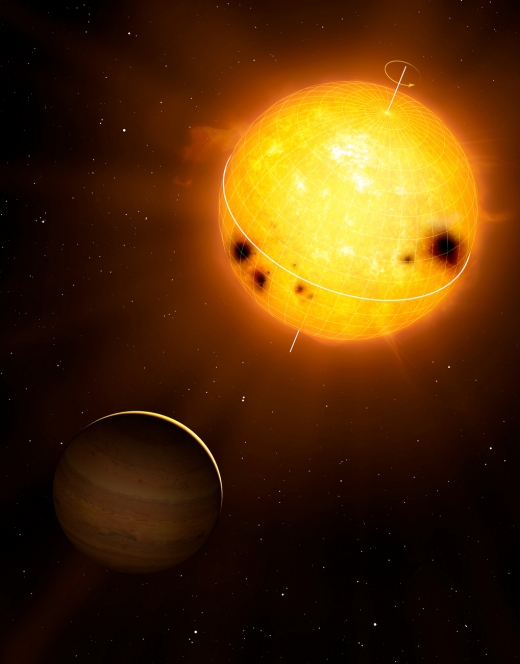
Image: Artist’s impression of one of the new worlds that PLATO will discover. Among those there will be Earth-like planets around Sun-like stars with the potential to host life. Credit & copyright: MPS/ Mark A. Garlick (markgarlick.com).
Bear in mind, too, that unlike the upcoming TESS (Transiting Exoplanet Survey Satellite) mission, PLATO will have the ability not only to do asteroseismology (quite useful in determining the size of exoplanets it discovers), but also to look for Earth-like planets around stars like our G-class Sun. TESS should give us plentiful information about planets around M-dwarfs, but PLATO backs us out to Earth-like worlds around stars like our own.
Using 34 separate small telescopes and cameras, PLATO’s current plan calls for a four-year observation period consisting of long-duration observations of two sky fields lasting two years each. But this could change: ESA also charts an alternative course including a single, three-year long-duration phase and a one year phase with several different pointings. The final strategy is not to be decided until two years before launch, but depending on the choice, the mission will cover between 10 percent and 50 percent of the sky during the nominal mission. An extended mission of up to four years will be a built-in option.
Orbiting the L2 Lagrangian point, PLATO should be able to characterize numerous rocky, icy or giant planets at unprecedented levels of precision, with radius measurements down to 3 percent and mass determinations better than 10 percent precision, assembling a catalog of confirmed and characterized planets with known mean densities, compositions and evolutionary stages, some of them being, it is assumed, planets in the habitable zone.
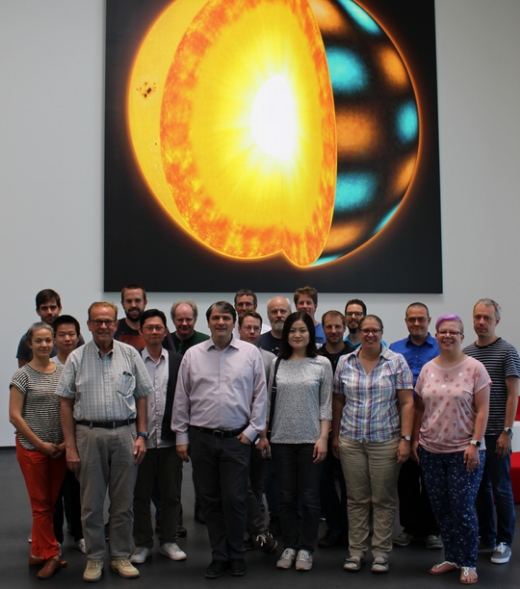
Image: The PLATO-team at the Max Planck Institute for Solar System Research. Credit & copyright: MPS.
ESA’s point about planetary evolution is telling, especially in light of the work we looked at yesterday on mini-Neptunes and the ‘gap’ between them in Kepler data and smaller, rocky worlds. The focus on highly accurate radius and mass measurements and better determination of stellar ages is designed to give us a better understanding of planetary changes over time.
Terrestrial planets can lose their primordial hydrogen atmospheres, later developing secondary atmospheres and perhaps life. A key goal for PLATO, then, is to study the physical and dynamical processes on Earth-like planets at different epochs in the life of a stellar system, with asteroseismology being a key determinant of host star ages. ESA believes asteroseismology can now determine a star’s age to a precision of 10 percent.
And, of course, we continue the quest for planets in the habitable zone of their star. PLATO’s long-duration observations of a star field should allow the mission to capture two transits of any Earth twin it finds around a G-class star. “With this concept and the high precision of the instrument we will find rocky planets orbiting sunlike stars and will be able to characterise them accurately.” says Heike Rauer (DLR-Berlin), principal Investigator of the mission.
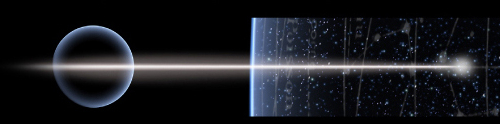
by Paul Gilster | Jun 20, 2017 | Exoplanetary Science |
As announced yesterday at NASA Ames, the Kepler team has released the final Kepler catalog from the spacecraft’s first four years of data and its deep stare into Cygnus. The numbers still impress me despite our having watched them grow with each new report: We have 4034 planet candidates, of which 2335 have been verified as exoplanets. More than 30 of the approximately 50 near-Earth sized habitable zone candidates have been verified.
The new release brought us 219 new candidates, 10 of them habitable zone possibilities, giving us a final catalog that is our first take on the prevalence and characteristics of planets in the Milky Way, and paving the way for future space-based instruments as we look for targets for atmospheric characterization and direct imaging. By introducing simulated planet transit signals and adding known false signals, the researchers were able to tighten up the catalog, ensuring against errors in the analysis growing out of the team’s processing methods.
The Kepler data are also the subject of a new take on planetary demographics, as seen in the image below, which takes us into the realm of the kind of planets we do not see in our Solar System, so-called ‘mini-Neptunes’ ranging from 2 to 3.5 times the size of Earth.
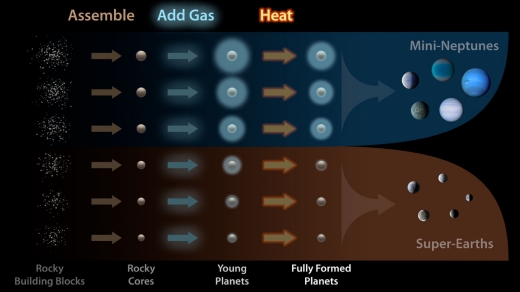
Image: This diagram illustrates how planets are assembled and sorted into two distinct size classes. First, the rocky cores of planets are formed from smaller pieces. Then, the gravity of the planets attracts hydrogen and helium gas. Finally, the planets are “baked” by the starlight and lose some gas. At a certain mass threshold, planets retain the gas and become gaseous mini-Neptunes; below this threshold, the planets lose all their gas, becoming rocky super-Earths. Credit: NASA/Kepler/Caltech (R. Hurt).
A research group working under Andrew Howard (Caltech) has drilled deep into the dataset, measuring the size of 1300 stars in the Kepler field of view to determine the radii of 2025 Kepler planets with four times more precision than had previously been achieved. Out of this work on planetary sizes comes the finding that these planets can be classified into two distinct groups: Rocky Earth-like planets and mini-Neptunes. From the paper:
We find evidence for a bimodal distribution of small planet sizes. Sub-Neptunes and super-Earths appear to be two distinct planet classes. Planets tend to prefer radii of either ?1.3 R? or ?2.4 R?, with relatively few planets having radii of 1.5–2.0 R?. Planets in the gap have the maximum size for a rocky core, as seen in previous studies of bulk planet density and of ultra-short period planets. We posit that the bimodal planet radius distribution stems from differences in the envelope masses of small planets. While our current dataset is insufficient to distinguish between theoretical models that produce the gap, it charts a path forward to unraveling further details of the properties of the galaxy’s most abundant planets.
Although most of the Kepler planets have proven to be between the size of the Earth and Neptune, the planets were previously thought to span the range between the two rather than to fall into distinct groupings. Erik Petigura (Caltech) is a co-author of the new study, which will appear in The Astronomical Journal, along with two other papers that make up an observational program known as the California-Kepler Survey (see citations below):
“In the solar system, there are no planets with sizes between Earth and Neptune. One of the great surprises from Kepler is that nearly every star has at least one planet larger than Earth but smaller than Neptune. We’d really like to know what these mysterious planets are like and why we don’t have them in our own solar system.”
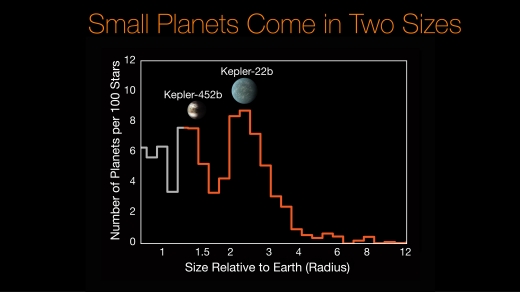
Image: Researchers using data from the W. M. Keck Observatory and NASA’s Kepler mission have discovered a gap in the distribution of planet sizes, indicating that most planets discovered by Kepler so far fall into two distinct size classes: the rocky Earths and super-Earths (similar to Kepler-452b), and the mini-Neptunes (similar to Kepler-22b). This histogram shows the number of planets per 100 stars as a function of planet size relative to Earth. Credit: NASA/Ames/Caltech/University of Hawaii (B. J. Fulton).
The Keck work involved researchers at UC Berkeley, Harvard, the University of Hawaii, Princeton and the University of Montreal as well as Caltech, a multi-year project analyzing the Kepler spectral data to obtain precise measurements of the host stars, which then allowed the scientists to refine the sizes of the planets orbiting those stars. Why the planets exist in two distinct groups — with a clear gap between rocky Earths and mini-Neptunes — remains problematic. Are Earth-sized planets the norm, with some of them simply obtaining enough of a gas envelope to become mini-Neptunes? Andrew Howard comments:
“A little bit of hydrogen and helium gas goes a very long way. So, if a planet acquires only 1 percent of hydrogen and helium in mass, that’s enough to jump the gap. These planets are like rocks with big balloons of gas around them. The hydrogen and helium that’s in the balloon doesn’t really contribute to the mass of the system as a whole, but it contributes to the volume in a tremendous way, making the planets a lot bigger in size.”
On the other hand, the researchers speculate, it may be that planets without enough of a gas envelope to fall into the gap can lose gas because of radiation from the host star. In this scenario, planets that wind up in the gap aren’t likely to stay there for long, producing thin atmospheres that are quickly blown off. Either scenario produces the populations we see in the new data, but we’ll need to learn more about the composition of mini-Neptunes to get a picture of why they seem to form so easily around other stars but not around our Sun.
The paper adds this:
…making a planet with a thin atmosphere requires a finely tuned amount of H/He. Second, photoevaporating a planet’s envelope significantly changes its size. Our observation of two peaks in the planet size distribution is consistent with super-Earths being rocky planets with atmospheres that contribute negligibly to their size, while sub-Neptunes are planets that retain envelopes with mass fractions of a few percent.
Three papers from the California-Kepler Survey are now available on the arXiv site in preprint form, all of them accepted for publication at The Astronomical Journal: Petigura et al., “The California-Kepler Survey. I. High Resolution Spectroscopy of 1305 Stars Hosting Kepler Transiting Planets” (preprint); Johnson et al., “The California-Kepler Survey. II. Precise Physical Properties of 2025 Kepler Planets and Their Host Stars” (preprint); and Fulton et al., “The California-Kepler Survey. III. A Gap in the Radius Distribution of Small Planets” (preprint).
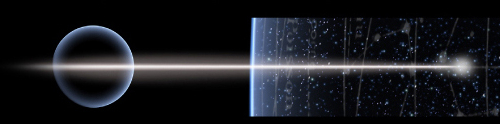
by Paul Gilster | Jun 19, 2017 | Exoplanetary Science |
The Pale Red Dot campaign that discovered Proxima Centauri b produced one of the great results of exoplanet detection. For many of us, the idea that a world of roughly Earth mass might be orbiting in Proxima Centauri’s habitable zone — where liquid water can exist on the surface — was almost too good to be true, and it highlighted the real prospect that if we find such a planet around the closest star to our own, there must be many more around similar stars. Hence the importance of learning more about our closest neighbors.
Which is why it’s so heartening to see that Pale Red Dot is by no means done. This morning, the team led by Guillem Anglada-Escudé (Queen Mary University, London) announced plans to acquire data from the European Southern Observatory’s HARPS instrument (High Accuracy Radial velocity Planet Searcher) in a new campaign to study not just Proxima Centauri in search of further planets, but also the red dwarfs Barnard’s Star and Ross 154.
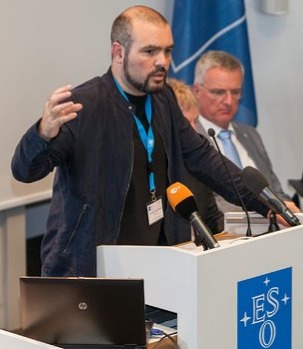
Also involved will be a network of small telescopes performing photometric monitoring, including the Las Cumbres Global Observatory Telescope network, SpaceObs ASH2 in Chile, the Observatorio de Sierra Nevada and the Observatorio Astronómico del Montsec, both in Spain. But the star of the show continues to be HARPS, a high-precision spectrograph attached to the ESO’s 3.6-meter telescope at La Silla. HARPS is capable of detecting radial velocity motions down to 3.5 kilometers per hour, the pace of a leisurely evening walk.
Image: Lead author Guillem Anglada-Escudé speaking at a press conference in Garching, Germany about the 2016 discovery of Proxima Centauri b. Credit: ESO/M. Zamani.
Are there other planets around Proxima Centauri? The findings around TRAPPIST-1, all seven of them, give reason to hope that we’ll make further discoveries. As to Barnard’s Star, we still have no information about planets there, although for a time in the mid-20th Century, it was thought due to instrument error that there might be one or more gas giants orbiting the star. We know now that that isn’t the case, but the possibility of terrestrial-class worlds remains.
I’ll have more to say about that situation later in the week, but do want to note that the reason the Project Daedalus planners chose Barnard’s Star as their mission target was the supposition that those planets existed. Proxima Centauri would obviously have been a closer target.
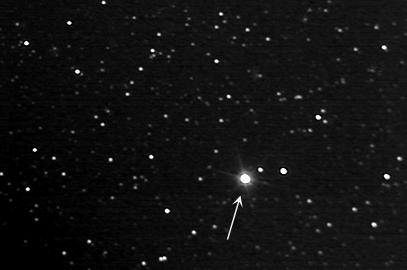
Image: Barnard’s Star ca. 2006. Credit: Steve Quirk.
The M-dwarf Ross 154 is just under 10 light years from Earth, the nearest star in Sagittarius. That distance is closing at a good clip (in astronomical terms), so that the star will come to within about 6.4 light years in another 157,000 years. Like Proxima Centauri, Ross 154 is a UV Ceti-type flare star, producing major flares on the order of every two days. Given that flare activity in M-dwarfs is a major factor in the question of whether life can develop, finding planets close enough to be characterized by later space and ground telescopes would be a significant development, and the more systems the better to allow comparative analysis.
It will be fascinating to watch the new Pale Red Dot campaign develop, for these observations will be highly visible to the general public. While Pale Red Dot presented its results on Proxima b to the public only after extensive peer review, the observational data from the new campaign, beginning with Proxima Centauri, will be revealed and discussed in real time.
The scientists involved intend to maintain an active social media presence supported by various online tools. Keep an eye on the Red Dots Facebook page, the Red Dots Twitter account and the #reddots hashtag, as well as the main project page, where updates and featured contributions from the community will be posted on a regular basis.
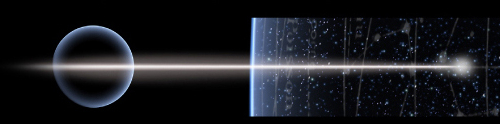
by Paul Gilster | Jun 16, 2017 | Missions |
Beamed propulsion concepts are usually conceived in terms of laser or microwave beams pushing a lightsail. But as we’ve seen over the years, there are other ways of thinking about these things. Clifford Singer went to work back in the 1970s on the concept of pellet streams fired by an accelerator, each pellet a few grams in size. The idea here is to vaporize the pellets when they reach the spacecraft, their energy being redirected as a plasma exhaust.
There are enough interesting variations on the idea that I’ll probably return to it soon. But over the weekend, an email from Jeff Greason reminded me of Jordin Kare’s unusual ‘fusion runway’ idea, to which he attached the moniker the ‘Bussard Buzz Bomb.’ Kare is an astrophysicist and space systems consultant with a background in laser technologies. He’s been involved in studies of laser launch methods, in which beamed energy is focused on an onboard heat exchanger that converts liquid propellant into a gas to produce thrust. Currently he serves as chief scientist for LaserMotive, a laser power transmission firm in Kent, WA.
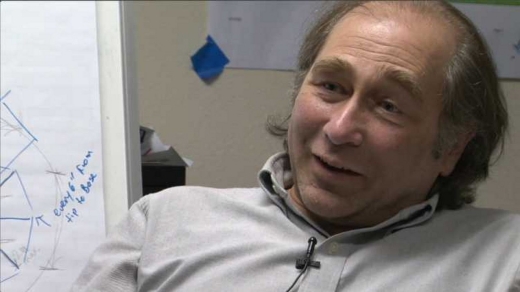
Image: Astrophysicist and space systems consultant Jordin Kare.
In the interstellar community, Kare is best known for SailBeam, where pellet propulsion is supplanted by tiny micro-sails that are pushed at huge velocities to the spacecraft, to be vaporized there much in the manner of Singer’s pellets. His sails turn out to require a smaller optical system than would be needed to push a large sail, and they can be driven to high accelerations while still close to the beam, reducing pointing and collimation challenges.
But I haven’t said much in these pages about Kare’s fusion runway, which he presented at a Workshop on Advanced Space Propulsion at the Jet Propulsion Laboratory back in the late 1990s. In 2003, when I interviewed him for my Centauri Dreams book, Kare gave me a breakdown of the concept. The idea harkens back to pellets, in this case fusion fuel pellets made up of deuterium and tritium that are slammed together to achieve ignition.
The pellets are laid down in an outbound track for the spacecraft that will eventually use them, deployed in advance by small spacecraft seeding the runway along the route of flight. Kare thinks in terms of a runway about half a light-day in length. The accelerating spacecraft would gobble up the fusion pellets one at a time, taking about ten days to exit the Solar System, moving along a runway track that stretched from near Earth to beyond the orbit of Pluto.
What kind of a craft would this be? Think in terms of a vehicle in the shape of a doughnut, or perhaps in more elongated form as a cylinder. The spacecraft would have its own supply of fusion fuel pellets. As the craft accelerates, it drops a pellet into the central ‘hole’ when one of the pellets of the fusion runway is about to be encountered. Nearing the end of the fusion runway, the spacecraft is being driven by fusion explosions at the rate of thirty per second.
The fusion runway relies on impact fusion, with the departing spacecraft first needing to reach speeds high enough (about 200 kilometers per second, by Kare’s reckoning) to ignite the reaction. Once ignition is achieved, the craft continues to accelerate along the runway track. The runway length would have to be adjusted depending on the mission, with robotic probes obviously capable of coping with far higher accelerations than humans. Add a human crew at 1 g of acceleration and a fusion runway might need to stretch out to a tenth of a light year.
String enough fuel pellets along the runway and the spacecraft gets up to ten percent of c. Although Kare built his workshop presentation around a one-ton interstellar probe, he sees the concept as scalable, telling me in that interview: “The fusion runway doesn’t care if you’re working with a ten or a hundred ton probe. You just need more pellets. You don’t need to build larger lasers. So it probably scales up better than most other schemes.”
Several other advantages emerge in the fusion runway concept. So-called ‘impact fusion’ doesn’t require the exquisitely symmetrical fuel pellets demanded by inertial confinement methods, nor does it demand that each pellet be fed energy simultaneously from every direction. Remember that Kare is assuming a spacecraft that is already moving — through some other energy source — at 200 kilometers per second to achieve ignition. From that point on, velocity depends upon the number of fuel pellets available in the runway ahead.
When I think about possible show-stoppers here, I wonder about accuracy. After all, each runway pellet has to hit the ship-borne pellet precisely, though Kare believes that this could be managed by laser pulses guiding the pellets internally. Perhaps the ship can be designed so as to channel runway pellets to the exact point of collision. Also challenging is the magnetic nozzle that will be necessary to contain the fusion explosions and direct their energy.
As far as getting up to speed, Geoff Landis told me some years back that a close pass by the surface of the Sun could be used to reach somewhere in the range of 500-600 kilometers per second. That could give you the velocity needed for ignition. Line the fuel pellets up so as to begin hitting them outbound and the method could work. In our recent email exchange, Landis does question Kare’s 200 kilometers per second as the sufficient velocity to ignite impact fusion — some figures in the literature point to 3500 km/s for a deuterium/tritium mixture.
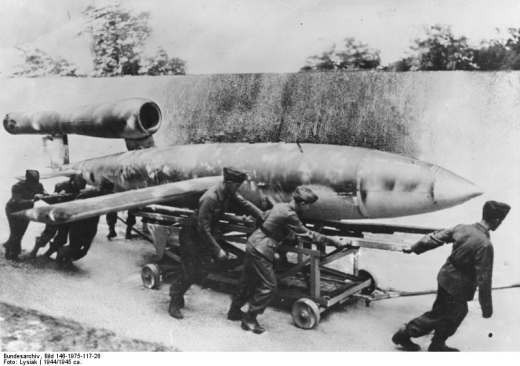
Image: Not exactly an interstellar prototype, but the German V1 gives its name to an advanced propulsion concept because of how it would sound (if you could hear it). Credit: Bundesarchiv, Bild 146-1975-117-26 / Lysiak / CC-BY-SA 3.0.
The ‘buzz bomb’ reference? That one is easy. The German V-1 was a pulse-jet rocket that gave off a characteristic staccato buzzing sound much like what a fusion runway spacecraft would sound like if you could hear it at all. The nod to Robert Bussard stems from the latter’s work on interstellar ramjet concepts, craft that pull in interstellar hydrogen to serve as fuel. Thus we have, as so often in interstellar studies, a hybrid design putting two distinct propulsion concepts together in ways that attempt to enhance the performance of each.
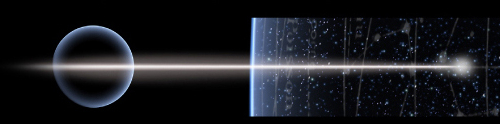