A growing interest in JPL's Solar Gravitational Lens mission here takes Wes Kelly on an odyssey into the past. A long-time Centauri Dreams contributor, Wes looks at the discovery of gravitational lensing, which takes us back not only to Einstein but to a putative...
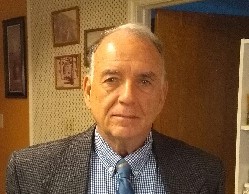