by Paul Gilster | Nov 22, 2008 | Exoplanetary Science |
Images of distant exoplanets, once only a wish for future space missions, have begun to turn up with a certain regularity. The three planets around HR8799 and the single gas giant around Fomalhaut were announced on the same day, while a week later we once again have Beta Pictoris in focus, a young star so well studied that images of its dust disk go back to the mid-1980s. A new analysis of 2003 data from the Very Large Telescope now brings a team of French astronomers to offer a probable — but not certain — image of what may turn out to be Beta Pictoris b.
The observations seem to show a gas giant some eight times more massive than Jupiter, orbiting at roughly 8 AU, not far inside Saturn’s orbit in our own Solar System. But astronomer Gael Chauvin (Laboratoire d’Astrophysique de l’Observatoire de Grenoble) is quick to qualify the finding:
“We cannot yet rule out definitively, however, that the candidate companion could be a foreground or background object. To eliminate this very small possibility, we will need to make new observations that confirm the nature of the discovery.”
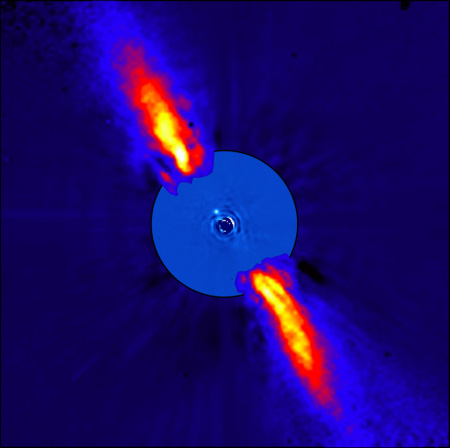
Image: This composite image represents the close environment of Beta Pictoris as seen in near infrared light. This very faint environment is revealed after a careful subtraction of the much brighter stellar halo. The outer part of the image shows the reflected light on the dust disc, as observed in 1996 with the ADONIS instrument on ESO’s 3.6 m telescope; the inner part is the innermost part of the system, as seen at 3.6 microns with NACO on the Very Large Telescope. The newly detected source is more than 1000 times fainter than Beta Pictoris, aligned with the disc, at a projected distance of 8 times the Earth-Sun distance. Because the planet is very young, it is still hot, with a temperature around 1200 degrees C. Both parts of the image were obtained on ESO telescopes equipped with adaptive optics. Credit: ESO/A.-M. Lagrange et al.
Take note of this in the above statement: The source is more than 1000 times fainter than Beta Pictoris — remember that this is at infrared wavelengths, where the contrast between the primary and candidate planet is minimized. A look at this same system in visible light would come up short, as the star’s glare would drown out any planetary signature.
That ‘small possibility’ of a spurious identification seems even smaller when you consider that an examination of Hubble archives turned up nothing by way of a foreground or background object. Moreover, the candidate object is in the plane of the disk, with the right mass and distance to meld with what we’ve already learned about this well studied environment. Beta Pictoris b would be closer than any planet we’ve found via direct imaging, an object bright in the infrared around a young star (12 million years old) that is still growing its planetary system. Refining our detection methods for cooler and older planets should widen the field of directly imaged worlds and offer new data on planetary formation.
Here’s a chart showing candidate planetary systems that have been imaged to this point (you’ll need to click to enlarge):
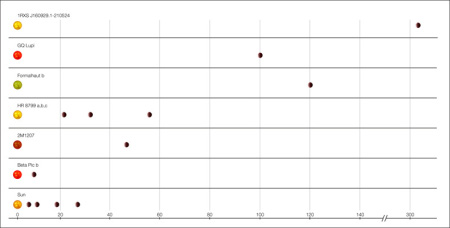
Image: This diagram compares the various candidate planetary systems that have been imaged with our own Solar System. Indicated are the star and the position of the candidate planets. The probable planet around Beta Pictoris is the closest to its host star of all extra-solar planets yet imaged, and is comparable to Saturn as far as its distance is concerned. The scale is the distance between the Earth and the Sun. A list of all candidate exoplanets directly imaged can be found at here. Credit: ESO
I don’t see the paper up on the arXiv server yet, but look here for a preprint. Submitted to Astronomy & Astrophysics, it’s Lagrange et al., “A probable giant planet imaged in the ? Pictoris disk. VLT/NACO Deep L-band imaging.”
by Paul Gilster | Nov 21, 2008 | Communications and Navigation |
Networking deep space should be a priority for future missions. If we can set up robust networking between spacecraft, we relieve the Deep Space Network of a huge burden, that of having to communicate directly with each spacecraft for tasks that are essentially routine. No more maneuvering huge dishes to catch one fleeting signal, at least not for missions to come. Instead, we could rely on spacecraft to create their own file transfers, move their own traffic to astronauts (remember the video mail in 2001: A Space Odyssey?), and manage local operations.
Why not use the Internet we’ve already got? Unfortunately, the TCP/IP (Transmission Control Protocol/Internet Protocol) tools we use today are ‘chatty,’ a term that means the computers that run them exchange data over and over again through the course of a transaction. Suppose you want to send a file through FTP (File Transfer Protocol). Doing so takes eight round trips of data between the computers involved before the file can be sent. Not only that, but FTP will time out after a few minutes of inactivity. Try that on a target that’s ten light hours away and the delay times will stretch a routine file swap into a multi-day event.
Vinton Cerf, the visionary behind the original TCP/IP work (and now a Google vice president), has been developing new protocols to handle this problem for a decade. The term to master is Disruption-Tolerant Networking (DTN), and it’s just been through a successful shakedown. Because DTN assumes no continuous connection, it uses store-and-forward methods to hold data until they can be sent. Says Leigh Torgerson (JPL):
“In space today, an operations team has to manually schedule each link and generate all the commands to specify which data to send, when to send it, and where to send it. With standardized DTN, this can all be done automatically.”
It’s satisfying to learn that the EPOXI spacecraft, part of the extended mission of the original Deep Impact vehicle, is one of the nodes on the nascent network, the other nine being simulated spacecraft run through the Jet Propulsion Laboratory. The goal is to get the technology ready for regular use, with a new round of testing scheduled for the International Space Station next summer. And EPOXI, which runs the EPOCH (Extrasolar Planet Observation and Characterization) observing program, adds a nice touch of interstellar drama to the first round, even though the spacecraft itself is, at 32 million kilometers, relatively close to home.
The first intensive use of interplanetary networking is surely going to occur around Mars, where landers can communicate with rovers and the satellites orbiting above. But it’s interesting from the point of view of pure communications that the DTN techniques could have uses here on Earth, particularly in areas where Internet access is needed but sender and receiver may have entirely different schedules. Until Net access is ubiquitous, there will be many places on this planet that can benefit from a system that knows enough to hold data until a signal is available.
For useful background documents (although the pages have not been updated in some time), check the Interplanetary Internet Project site. This NASA news release is also available.
by Paul Gilster | Nov 20, 2008 | Exotic Physics |
What is the unusual source of high-energy cosmic rays that has been discovered within 3000 light years of the Sun? Everyone loves a mystery, and this one has all the earmarks of a classic. The source was found by the Advanced Thin Ionization Calorimeter (ATIC) experiment, which was lofted to high altitude above Antarctica via helium-filled balloon. Behind the experiment was the goal of studying cosmic rays that are otherwise shielded from the surface by the Earth’s atmosphere, but among the results was an unexpected finding.
Cosmic ray electrons at 300 to 800 billion electron volts are simply too powerful to be regarded as standard fare, for these particles lose energy as they move through the galaxy. That means that a study like this should see fewer electrons at higher energies. Nearby sources, on the other hand, stand out, making it clear there is what principal investigator John Wefel (Louisiana State) calls “…a very interesting object near our solar system waiting to be studied by other instruments.” We’re talking about candidates ranging from a pulsar or a black hole to a supernova remnant or even a mini-quasar. See this news release for more.
Then again, could this be an indication of dark matter in the neighborhood? The annihilation of exotic particles caused by two of them colliding — some believe such particles are candidates for dark matter — could produce just what we are seeing, says Eun-Suk Seo (University of Maryland), who adds that the process would produce normal electrons and protons and their antimatter equivalents, positrons and antiprotons.
That latter possibility is highly theoretical, depending upon dark matter theories that invoke extra dimensions, but we still have to find out whether there is an unseen object that is accelerating electrons to these energy levels, surely the first step in characterizing this mysterious source. And here another mission may help us. For what ATIC has uncovered is roughly similar to data from the PAMELA (Payload for Antimatter Matter Exploration and Light-nuclei Astrophysics) satellite, which likewise found a source of energetic particles in the same range. From the paper on the PAMELA results:
Our results clearly show an increase in the positron abundance at high energy that cannot be understood by standard models describing the secondary production of cosmic-rays. Either a significant modification in the acceleration and propagation models for cosmic-rays is needed, or a primary component is present. There are several interesting candidates for a primary component, including the annihilation of dark matter particles in the vicinity of our galaxy. There may also be a contribution from near-by astrophysical sources, such as pulsars.
So what are these exotic particles? Here I want to quote from an article by Geoff Brumfiel on this work in Nature, running in the same issue as the ATIC results:
The exact nature of the dark-matter particles that produce electrons is uncertain, but one idea is that they may be ordinary particles that spend part of their lives in a compact extra dimension of space. Whereas the particles would appear relatively stationary to observers trapped in three spatial dimensions, they could be moving at ultra-high speeds in a fourth spatial dimension. At high speeds, they would create a gravitational force that could be felt by matter trapped in three dimensions of space-time.
I’ll opt for a nearby pulsar, as discussed in the above article, one whose magnetic fields would create the needed acceleration of electrons, but we may just have to wait for the Fermi Gamma-ray Telescope (originally called GLAST) to verify both ATIC and PAMELA. The paper on ATIC is Chang et al., “An excess of cosmic ray electrons at energies of 300-800 GeV,” Nature 456 (20 November 2008), pp. 362-365 (abstract). The PAMELA work is Adriani et al., “Observation of an anomalous positron abundance in the cosmic radiation,” submitted to Nature and available online.
by Paul Gilster | Nov 19, 2008 | Astrobiology and SETI |
A Dyson Sphere makes an extraordinary setting for science fiction. In fact, my first knowledge of the concept came from reading Larry Niven’s 1970 novel Ringworld, a book that left such an impression that I still recall reading half of it at a sitting in the drafty little parlor of a house I was renting in Grinnell, Iowa. Ringworld had just come out as a Ballantine paperback with the lovely cover you see below. I was hooked after about three pages and read deep into a night filled with wind and snow.
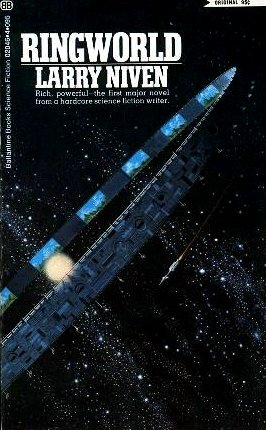
It could be argued, of course, that a ring made out of planetary material, a habitat so vast that it completely encircles its star, is actually one of the smaller Dyson concepts. It was in 1960 that Freeman Dyson suggested how a civilization advanced to the point of such astro-engineering might use everything it found in its solar system to create a cloud of objects, a swarm that would make the most efficient use of its primary’s light. And as you keep adding objects, you point to the ultimate outcome, a Dyson Sphere that completely envelopes the star from which it draws its energy.
A Dyson Sphere Search with IRAS
Last April I looked at Dyson spheres in the context of an article by Bruce Dorminey that considered new SETI strategies. Now I see that Richard Carrigan, a retired physicist from Fermilab, has added a new paper to the arXiv site, one that discusses the work reviewed in that earlier story. Carrigan has been examining sources identified by the Infrared Astronomical Satellite (IRAS), the idea being to look for objects that seem to be radiating waste heat in such a way that they might be Dyson Spheres of one kind or another. A fully enveloped star won’t be visible to the eye, but Carrigan’s infrared search covers the blackbody temperature region from 100 to 600 degrees Kelvin for full or partial Spheres.
The data come from an IRAS database that covers 96 percent of the sky and includes some 250,000 sources. Exciting stuff on the face of it, because unlike a conventional SETI search, a hunt for Dyson Spheres involves no necessary intent to communicate on the part of the civilization in question. And when you’re dealing with SETI, the fewer preconceptions you bring to the dance, the better. Here’s the thinking behind Carrigan’s attempt:
For a Dyson Sphere the stellar energy from the star would be reradiated at a lower temperature. If the visible light was totally absorbed by a thin “shell” a pure Dyson Sphere signature would be an infrared object with luminosity equivalent to the invisible star and a Planck or blackbody distribution with a temperature corresponding to the radius of the spherical shell formed by the cloud of objects. For a sun-like star with the shell at the radius of the Earth the temperature would be approximately 300º K.
Sorting the Evidence
A distinct signature? You would hope so, and if that is the case, we can dig through our data practicing what Carrigan delightfully calls ‘cosmic archaeology,’ using data that cover the 8 to 100 micron infrared range needed to study a Dyson Sphere’s emissions under these assumptions. Yet an identification runs into immediate problems, not the least of which is the need to differentiate any candidate from natural sources that show much the same signature. A cocoon of gas and dust around a young star, for example, might mimic an artificial source.
Carrigan goes through the possibilities — protostars, planetary nebulae, dying stars — and weighs their telltale infrared identity against a true Dyson Sphere, with notes on how to tell the natural from the potentially artificial. Here he considers the methods (italics mine):
A Dyson Sphere candidate with a blackbody distribution can have several characteristics such as a blackbody temperature, the distance from our Sun, magnitude in the infrared, and variability. It may also have a stellar signature in the visible or infrared. Slysh (1985) notes, “The confusion between red giants with thick circumstellar envelopes and possible Dyson Spheres in the IRAS survey is a serious problem, and to differentiate the two we need additional data.” …[S]ome of the source types discussed above populate the same region of an infrared color-color plot as a Dyson sphere candidate would. Non-Dyson Sphere objects can be eliminated using discriminants like spectral lines in the infrared or radio regime, implausible blackbody temperatures, established classifications, and statistical departures from a blackbody distribution.
A Dwindling List
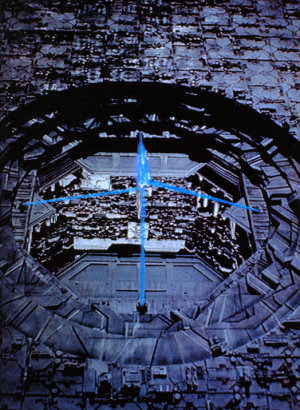
So we still have a chance to find a true Dyson Sphere, assuming one or more are out there. If I had more money to burn, I would ring up Tibor Pacher with an offer to make another bet, this one saying that no Dyson Sphere will be found in this century. Tibor is bound to take that one, but I’ve lost several other bets recently and had better put down my cards (our other bet, on the date of the first true interstellar mission, is viewable at the Long Bets site; feel free to comment on either side of that one).
Image: A Dyson Sphere as envisioned by the producers of Star Trek: The Next Generation, from the episode “Relics.” Credit: Paramount Pictures.
Bet or no, the process of working through the database is fascinating, but the list of candidates quickly dwindles in Carrigan’s discussion. We wind up with a scant seventeen possibilities, none of them particularly promising, though worthy of further study. Carrigan comments:
This search has shown that at best there are only a few quasi-plausible Dyson Sphere signatures out of the IRAS LRS sample in the 100 < T < 600 ºK temperature region. This limit includes both pure and partial Dyson Spheres. With several possible exceptions all the "good" sources identified in this search have some more conventional explanation other than as a Dyson Sphere candidate. In spite of the fact that there are many mimics such as stars in a late dusty phase of their evolution good Dyson Sphere candidates are quite rare!
Next Steps
Where do we go from here? Compiling more on the list of seventeen Dyson candidates would be the logical next step (Carrigan discusses how). And we can search further using the more powerful Spitzer Space Telescope, an instrument with greater angular resolution than IRAS and three orders of magnitude better sensitivity in the infrared ranges needed for this work. This would extend the survey out past the center of the galaxy, but we’ll lose some of the IRAS sources, which are too bright for Spitzer’s camera to avoid saturation. And only one of the seventeen candidates Carrigan finds would be covered by such a Spitzer study.
This intriguing work reminds us how early we are in the study of Dyson Spheres, and the broader attempt to identify astro-engineering on this vast scale. The Low Resolution Spectrometer aboard the IRAS satellite was only sensitive enough to track solar-sized Dyson Spheres out to a range of some 300 parsecs, which includes a million solar-type stars. Extending that reach, and finding ways to either rule out or strengthen the case for some of Carrigan’s seventeen candidates, is work that extends our existing radio and optical SETI methods. Beefing up our infrared tools will help us determine whether a concept once considered outrageous might conceivably flag an extraterrestrial presence.
The paper is Carrigan, “IRAS-based Whole-Sky Upper Limit on Dyson Spheres,” available online.
by Paul Gilster | Nov 18, 2008 | Antimatter |
Irradiate a millimeter-thick gold target with the right kind of laser and you might get a surprise in the form of 100 billion positrons, the antimatter equivalent of electrons. Researchers had been studying the process at Lawrence Livermore National Laboratory, where they used thin targets that produced far fewer positrons. The new laser method came about through simulations that showed a thicker target was more effective.
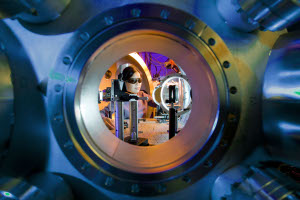
And suddenly lasers and antimatter are again making news. Hui Chen is the Livermore scientist behind this work:
“We’ve detected far more anti-matter than anyone else has ever measured in a laser experiment. We’ve demonstrated the creation of a significant number of positrons using a short-pulse laser.”
Image: Physicist Hui Chen sets up targets for the antimatter experiment at the Jupiter laser facility. Credit: Lawrence Livermore National Laboratory.
What’s happening here is that ionized electrons are interacting with gold nuclei, giving off energy that decays into matter and antimatter. It’s a method that has been studied before at Livermore, using the laboratory’s petawatt laser. An article by physicist Michael Perry explains the interactions that laser achieved, first produced about a decade ago:
The intense beam of Livermore’s Petawatt laser was powerful enough to break up atoms by causing reactions in their nuclei. Accelerated by the laser, electrons traveling at nearly the speed of light collided with nuclei in a gold foil target, producing gamma rays that knocked out some of the neutrons from other gold nuclei and caused the gold to decay into elements such as platinum. Gamma rays also zoomed in on a layer of uranium sitting behind the gold and split uranium nuclei into lighter elements. Before the Petawatt, all of these effects had been solely in the domain of particle accelerators or nuclear reactors.
Accelerated to energies exceeding 100 megaelectronvolts, the electrons in the gold targets produced high-energy x rays. These in turn decayed into pairs of electrons and their antimatter counterparts, positrons, in such large numbers as to possibly generate an electron-positron plasma, never before created in the laboratory. An intense beam of protons also turned up. Not only was the Petawatt the most powerful laser in the world, but, unexpectedly, it also was a powerful ion accelerator.
The petawatt laser was decommissioned in mid-1999. Its original development centered on the study of inertial confinement fusion, in which a pellet of fuel could be ignited through intense laser bombardment. Variations on inertial confinement fusion play interestingly through interstellar propulsion studies, including the massive Daedalus probe designed by the British Interplanetary Society, which would have used deuterium and helium-3 as its fodder for a trip to Barnard’s Star.
But the petawatt laser also opened up the possibility of using a laser to do things particle accelerators had been called upon to do in the past. And now we’ve moved significantly beyond the earlier results — the petawatt experimenters detected roughly 100 antimatter particles compared to Chen’s one million. And note this: Chen’s number refers to particles that were directly detected, a result that produces an overall estimate of 100 billion positrons produced in the entire experiment.
We’ve looked in these pages at the possibility of harvesting naturally forming antimatter found in our own Solar System, even near the Earth, where cosmic ray interactions with the upper atmosphere produce small quantities. And the presence of antimatter near the center of our galaxy has been established, detectable because matter/antimatter annihilation produces gamma rays. The trick has always been that harvesting antimatter — or producing it in accelerators — yields small amounts at great expense. The latest work at least offers hope for more robust laboratory study of a material whose propulsive properties have long attracted interstellar theorists.
Just how significant a step this is remains to be seen, but I note what Peter Beiersdorfer, who works with Chen at Livermore, has to say:
“We’ve entered a new era. Now, that we’ve looked for it, it’s almost like it hit us right on the head. We envision a center for antimatter research, using lasers as cheaper antimatter factories.”
We’ll know more shortly, for Chen is presenting her work at the American Physical Society’s Division of Plasma Physics meeting that runs through Friday this week. A Livermore news release is available. Thanks to Centauri Dreams reader Leith for the heads-up on this work.