by Paul Gilster | Nov 7, 2009 | Culture and Society |
My friend Tibor Pacher reports that the MiniSpaceWorld Design Contest is getting quite interesting, with six submissions of high quality, all going in different directions. Tibor’s goal is to build a scale model world that, like the famous Miniatur Wunderland in Hamburg, shows off space technology in tiny, exquisite detail. We’re used to seeing this kind of thing particularly in the model railroad world, so why not extend the idea into space as a tool for educators?
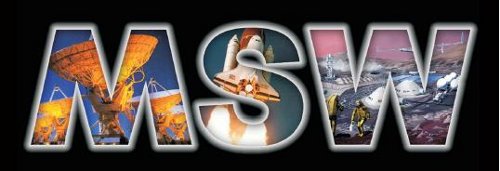
Now you can go to the site to see basic maps from the contestants. The contest award ceremony is to be held in Budapest on December 5 in the Congress Hall of the Research Centre for Social Studies, a Hungarian Academy of Sciences institute, and as Tibor notes, this is in the Budapest castle district, so there is much more to do in the area for those lucky enough to attend. Be aware that voting on the submissions is open until November 15, and have a look at the MSW site for more information.
by Paul Gilster | Nov 6, 2009 | Communications and Navigation |
If we can get the right kind of equipment to the Sun’s gravitational focus, remarkable astronomical observations should follow. We’ve looked at the possibilities of using this tremendous natural lens to get close-up images of nearby exoplanets and other targets, but in a paper delivered at the International Astronautical Congress in Daejeon, South Korea in October, Claudio Maccone took the lensing mission a step further. For in addition to imaging, we can also use the lens for communications.
The communications problem is thorny, and when I talked to JPL’s James Lesh about it in terms of a Centauri probe, he told me that a laser-based design he had worked up would require a three-meter telescope slightly larger than Hubble to serve as the transmitting aperture. Laser communications in such a setup are workable, but getting a payload-starved probe to incorporate a system this large would only add to our propulsion frustrations. The gravitational lens, on the other hand, could serve up a far more practical solution.
Keeping Bit Error Rate Low
Maccone goes to work on Bit Error Rate, a crucial measure of signal quality, in assessing the possibilities. Bit error rate charts the number of erroneous bits received divided by the total number of bits transmitted. Working out the numbers, Maccone posits a human probe in Centauri space trying to communicate with a typical NASA Deep Space Network antenna (70 meter dish), using a 12-meter antenna aboard the spacecraft (probably inflatable).
Using a link frequency in the Ka band (32 GHz), a bit rate of 32 kbps, and forty watts of transmitting power (and juggling the other parameters reasonably), the math is devastating: we get a 50 percent probability of errors. So much for data integrity as we operate within conventional systems.
But if we send Maccone’s FOCAL probe to the Sun’s gravitational lens at 550 AU, we now tap the tremendous magnification of the lens, which brings us a huge new gain. Using the same forty watts of power, we derive a completely acceptable bit rate. In fact, Maccone’s figures show that the bit error rate does not begin to become remotely problematic until we reach a distance of nine light years, when the increase in BER begins slowly increasing.
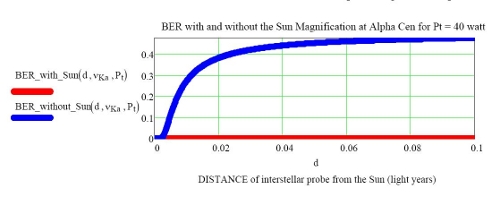
Image: Figure 4. The Bit Error Rate (BER) (upper, blue curve) tends immediately to the 50% value (BER = 0.5) even at moderate distances from the Sun (0 to 0.1 light years) for a 40 watt transmission from a DSN antenna that is a DIRECT transmission, i.e. without using the Sun’s Magnifying Lens. On the contrary (lower red curve) the BER keeps staying at zero value (perfect communications!) if the FOCAL space mission is made, so as the Sun’s magnifying action is made to work. Credit: Claudio Maccone.
Building a Radio Bridge
Now this is interesting stuff because it demonstrates that when we do achieve the ability to create a human presence around a nearby star, we will have ways to establish regular, reliable communications. A second FOCAL mission, one established at the gravitational lens of the target star, benefits us even more. We could, for instance, create a Sun-Alpha Centauri bridge. The bit error rate becomes less and less of a factor:
…the surprise is that… for the Sun-Alpha Cen direct radio bridge exploiting both the two gravitational lenses, this minimum transmitted power is incredibly… small! Actually it just equals less than 10-4 watts, i.e. one tenth of a milliwatt is enough to have perfect communication between the Sun and Alpha Cen through two 12-meter FOCAL spacecraft antennas.
This seems remarkable, but gravitational lenses make remarkable things possible. Recall that it was only months ago that the first tentative discovery of an extrasolar planet in the Andromeda galaxy (M31) was made, using gravitational lensing to make the observation.
Into the Galactic Bulge
Maccone goes on to work out the numbers for other interstellar scenarios, such as a similar bridge between the Sun and Barnard’s Star, the Sun and Sirius A, and the Sun and a Sun-like star in the galactic bulge. That third possibility takes us into into blue sky territory, but it’s a fascinating exercise. If somehow we could use the gravitational lens of the star in the galactic bulge as well as our own gravitational lens, we would have a workable bridge at power levels higher than 1000 watts.
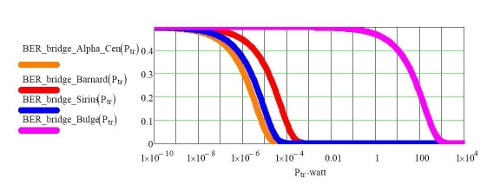
Image: Bit Error Rate (BER) for the double-gravitational-lens of the radio bridge between the Sun and Alpha Cen A (orangish curve) plus the same curve for the radio bridge between the Sun and Barnard’s star (reddish curve, just as Barnard’s star is a reddish star) plus the same curve of the radio bridge between the Sun and Sirius A (blue curve, just as Sirius A is a big blue star). In addition, to the far right we now have the pink curve showing the BER for a radio bridge between the Sun and another Sun (identical in mass and size) located inside the Galactic Bulge at a distance of 26,000 light years. The radio bridge between these two Suns works and their two gravitational lenses works perfectly (i.e. BER = 0) if the transmitted power higher than about 1000 watt. Credit: Claudio Maccone.
I’m chuckling as I write this because Maccone concludes the paper by imagining a similar bridge between the Sun and a Sun-like star inside M31, using the gravitational lenses of both. We’re working here with a distance of 2.5 million light years, but a transmitted power of about 107 watts would do the trick. This paper is a dazzling dip into the possibilities the gravitational lens allows us if we can find ways to reach and exploit it.
The paper is Maccone, “Interstellar Radio Links Enhanced by Exploiting the Sun as a Gravitational Lens,” presented at the recent IAC. I’ll pass along publication information as soon as the paper appears.
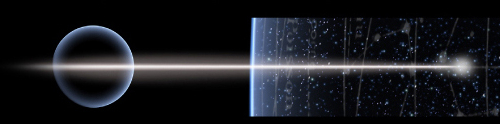
by Paul Gilster | Nov 5, 2009 | Deep Sky Astronomy & Telescopes |
The Earth receives thousands of tons of interplanetary dust every year as it makes its way around the Sun. Can we trace any of this material to a particular source? Scientists from the Carnegie Institution think the answer is yes, at least in the case of comet Grigg-Skjellerup. The Carnegie team worked with interplanetary dust particles (IDPs) collected by a NASA aircraft in 2003, just after the Earth had passed through the comet’s tail, focusing on the chemical, isotopic and micro-structural composition of the grains.
Collecting Comet Dust
A bit of background: NASA’s Scott Messenger (JSC-Houston) predicted that Grigg-Skjellerup dust grains could be captured in the stratosphere at a specific time of the year (the comet reappears every five years). The dust collection flights that followed his prediction involved an ER-2 high-altitude aircraft flown out of Dryden Flight Research Center in 2003. Out of this work came the new mineral now called Brownleeite, a manganese silicide found in one of the particles. NASA routinely collects IDPs in such flights.
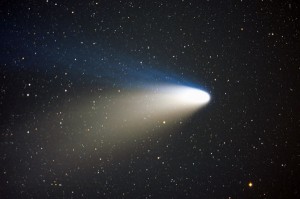
In the Carnegie IDP study, a particular signature seems to stand out. “What we found is that they are very different from typical IDPs,” says Carnegie’s Larry Nittler. “They are more primitive, with higher abundances of material whose origin predates the formation of the solar system.” That again points the finger at comet Grigg-Skjellerup, and it also offers the opportunity to study comet particles in the lab from more than a single comet. Recall that the Stardust mission brought back particles from comet 81P/Wild 2 in 2006.
Image: Comet Grigg-Skjellerup offers up material that is more than 4.5 billion years old. Credit: iStockphoto.
This is helpful stuff because comets are believed to incorporate matter from the Solar System’s formative era. We would expect such material to have escaped the heating and chemical processing that would have affected the planets, but interestingly enough, the Wild 2 dust included a great deal of altered material, as this news release points out. Grigg-Skjellerup’s dust tells us that all comets are not the same in terms of composition, giving us the chance, as Nittler says, to “…use them as tracers for different processes that occurred in the solar system four-and-a-half billion years ago.”
Cometary Differences and Similarities
We can also weigh the Grigg-Skjellerup material against the findings of the Deep Impact experiment on comet 9P/Tempel 1. From the abstract of the paper on this work:
Our study together with observations of comet 9P/Tempel 1 during the Deep Impact experiment and 81P/Wild 2 dust analyses reveal some compositional variations and many similarities among three Jupiter-family comets. Specifically carbonates and primitive organic matter or amorphous carbon were widespread in the comet-forming regions of the outer protoplanetary disk and not all comets contain as much inner solar system material as has been inferred for comet 81P/Wild 2.
Presolar grains — dust particles formed in previous star generations and in supernovae before the birth of the Solar System — provided the biggest surprise. They can be identified by their unusual isotopic compositions, but they’re normally quite rare. Yet in the dust particles associated with Grigg-Skjellerup they are tens of times more plentiful than in other primitive materials.
The paper is Busemann et al., “Ultra-primitive interplanetary dust particles from the comet 26P/Grigg-Skjellerup dust stream collection,” Earth and Planetary Science Letters, published online 8 October 2009 (abstract).
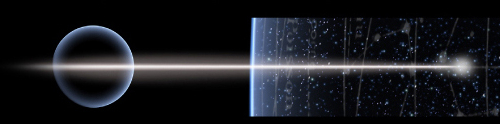
by Paul Gilster | Nov 4, 2009 | Exotic Physics |
If you’re going to snare dark matter, you’d better have incredibly accurate detectors. So the thinking goes at Case Western Reserve, where researchers are planning the most sensitive experiment yet to go after WIMPs (weakly interacting massive particles). WIMPs are almost impossible to detect because they don’t give off radiation and pass through normal matter unimpeded. The CWRU group has received a three year $3.2 million National Science Foundation grant to design a new WIMP detector.
The existence of dark matter is a theory that received support in 2006 when the collision of two distant galaxies was analyzed in ways that seemed to show the effects of dark matter on a cloud of galactic gas. Dark matter could provide the needed mass that keeps galaxies like the Milky Way from flying apart, but we still need a direct detection. The new experiment is a 20-ton liquid xenon detector called LZD. The Case Western group proposes LZD as an experiment for the Deep Underground Science and Engineering Laboratory planned for the abandoned Homestake Gold Mine, to be established almost a mile beneath Lead, South Dakota.
We’ve tracked earlier dark matter efforts in these pages, systems like the XENON 10 prototype in San Grasso, Italy and the Large Underground Experiment (LUX), which also operates in the former Homestake mine. But LZD would be seventy times larger than LUX and a whopping 2,000 times the size of XENON 10. The press materials on the detector claim it would increase the chance of spotting a WIMP by more than 30,000 times over XENON 10 and 150 times over LUX.
A WIMP colliding with a xenon atom should produce a tiny flash of light that LZD could trace and analyze. The experiment is to be lowered underground next year, where the WIMP investigation can proceed without impediment from the charged particles that continually strike the Earth’s surface in their billions. But LUX and a smaller interim detector called LZS will have to duke it out for funding with technologies that use germanium crystals frozen to nearly absolute zero, or liquid argon detectors. Masahiro Morii (Harvard) likes the xenon approach: “Liquid xenon has a distinct advantage: it’s straight forward to scale up,” Morii said. And, “It’s ahead of the other technologies by 3 to 5 years.”
Related: Measurements of the cosmic microwave background (CMB) using data from the QUaD telescope project near the South Pole provide further support for the standard cosmological model of the universe. That model predicts that dark matter and dark energy make up 95 percent of everything, with the ordinary matter we see and interact with accounting for just five percent. The measurements zeroed in on variations in the CMB’s temperature and polarization, which offer clues as to how matter in the early universe was distributed.
Creating a map of CMB polarization allows the researchers to investigate how light became polarized when it struck moving matter, pinpointing not only where matter existed but how it was moving. The results strongly match the predictions of temperature and polarization we derive from the standard cosmological model, which includes the existence of dark matter and dark energy.
Says Walter Gear (Cardiff University):
“Studying the CMB radiation has given us extremely precise pictures of the Universe at just 400,000 years old. When we first started working on this project the polarization of the CMB hadn’t even been detected and we thought we might be able to find something wrong with the theory. The fact that these superb data fit the theory so beautifully is in many ways even more amazing. This reinforces the view that researchers are on the right track and need to learn more about the strange nature of dark energy and dark matter if we are to fully understand the workings of the universe.”
The paper on the CMB work is Brown et al., “Improved Measurements of the Temperature and Polarization of the Cosmic Microwave Background from QUaD,” Astrophysical Journal 705 (November, 2009), pp. 978-999 (abstract).
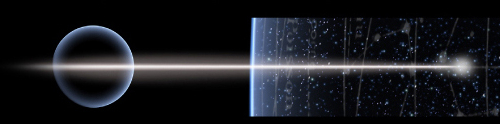
by Paul Gilster | Nov 3, 2009 | Exotic Physics |
By Larry Klaes
We have much to do as we scramble to explain the universe’s continuing acceleration. Dark energy seems to be demanded by the data, but there are holdouts who argue for a reinterpretation of General Relativity. Tau Zero journalist Larry Klaes looks at one proponent of a revised GR who sees exceptions to the rule in a far earlier era.
Albert Einstein’s work created one of the biggest revolutions in the history of science and radically changed our perceptions of the Cosmos. One of his later breakthrough ideas is the Theory of General Relativity, or GR for short. Really massive objects such as the Sun literally warp space and time around them as they move through the heavens. Since Einstein first published his ideas on GR in 1915, scientists have been able to use the theory to understand the behaviors of even more massive celestial bodies and the very beginning of the Universe itself.
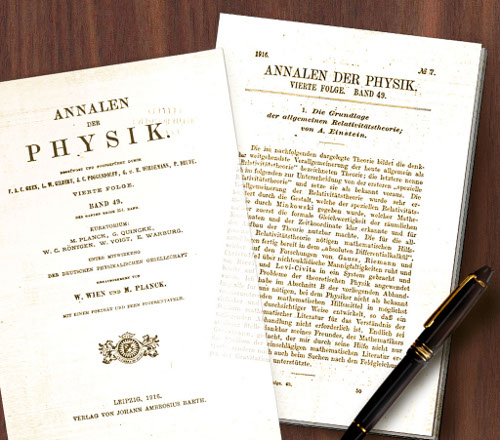
Image: This key Einstein paper included the effect of gravitation on the shape of space and the flow of time, proposing that matter causes space to curve. Credit: NCSA/UIUC.
The Need for a New Look
Now an assistant professor of cosmology at Cornell University thinks she may have found a deviation from the predictions of GR at the very largest cosmological scales. If her finding turns out to be true, it would be a major shift in our understanding of how our Universe works. Rachel Bean, whose paper on the subject has just appeared on the arXiv site, explains that while gravity is very well tested on Solar System scales, when it comes to the distances of the farthest galaxies, which are billions of light years from our own Milky Way galaxy, astronomers still have much to understand.
“Einstein’s theory of General Relativity tells us how gravity determines the relationship between the matter in the Universe and the Universe’s size,” says Bean. “If matter in the Universe is attracted by gravity, then the Universe’s size should be expanding at an ever slowing rate (decelerating). What observations show, though, is that in the last 6 billion years (out of the Universe’s 13.7 billion year existence), the expansion has been speeding up. So we need new physics, labeled ‘dark energy’, to explain this apparent disparity.”
Dark energy is a hypothetical force which makes up almost three-quarters of the mass-energy of the known Cosmos. Cosmologists currently use dark energy to explain why the Universe is expanding when it should otherwise stop moving or even collapse back upon itself.
Scientists do not yet know what dark energy is composed of. Adds Bean:
“There are two broad possibilities for what dark energy is. Either it is a strange, new type of matter that is not attracted by gravity, or Einstein’s Theory of General Relativity needs to be modified on cosmic scales. Part of my research is to try and understand the origins of dark energy and how we can use current and future astrophysical surveys to measure its properties.”
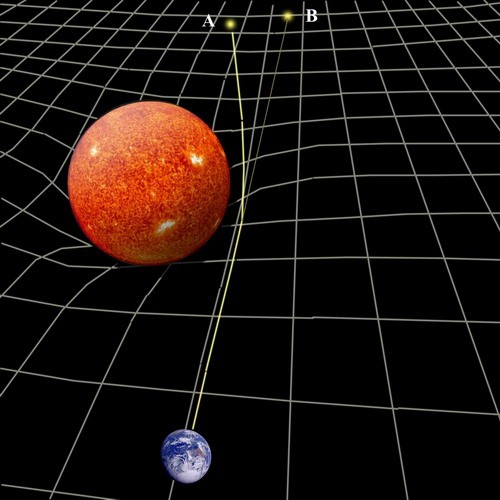
Image: The bending of starlight by matter and energy is well attested. Is there any way General Relativity may have differed in earlier eras? Credit: Ethan Siegel/Lewis & Clark College, OR.
Dark Energy and Cosmic Structure
Bean examined and compared the data collected from the recent Cosmic Evolution Survey, or COSMOS, which involved a number of telescopes both on the ground and in Earth orbit, including the Hubble Space Telescope (HST). The survey examined over two million galaxies, along with distant exploding suns called supernovae and the relic radiation created just 400,000 years after the formation of our Universe in the Big Bang, otherwise known as the Cosmic Microwave Background.
The Cornell scientist says that in order to distinguish between the two possible types of dark energy, astronomers need to include a second type of observations in addition to the distance measurements, namely how galaxies and clusters of galaxies, or large scale structures, evolve over time under the influence of gravity. Bean did this by examining the data on distant “lensed” galaxies whose light from their billions of suns is distorted by other galaxies between themselves and our Milky Way.
“My paper uses a combination of large scale structure and distance measurements to test gravity on cosmic scales to see whether it is consistent with GR or not,” declares Bean. “What I find is that there is a better fit to one time slice in the weak lensing data from the COSMOS survey using the HST, between 3 and 7 billion years after the Big Bang, if gravity is allowed to deviate from GR.”
A Modification to Gravity
If her idea proves to be correct, the results could “indicate that dark energy is related to a modification to gravity rather than a new type of matter.”
While Bean recognizes and admits there may be systematic errors in the data she studied, she also sees her work as a method for using these large scale structure datasets to test theories on dark energy:
“Future astronomical surveys coming in the next six years, namely the Dark Energy Survey (DES) and the Large Synoptic Survey Telescope (LSST), of which Cornell has just become an institutional member, will have much improved control over errors in the analysis and will be able to constrain the properties of gravity with far better precision.”
When it comes to the Universe, Bean says she was always interested in astronomy, mathematics, and physics as a child.
“My mum said that I used to go into bookshops even as a small child and buy math books rather than reading books. However, I didn’t get into astrophysics until my mid-20s after a few years working as an analyst at a Management Consultancy. Since then I’ve never looked back….”
The paper is Bean, “A weak lensing detection of a deviation from General Relativity on cosmic scales,” available online.