by Paul Gilster | Apr 3, 2012 | Exoplanetary Science |
The idea of ‘deep time’ exerts an abiding fascination. H.G. Wells took us forward to a remote futurity when his time traveler looked out on a beach dominated by a red and swollen Sun. But of course deep time goes in the other direction as well. I can remember wanting to become a paleontologist when I discovered books about the world of the dinosaurs, my mind reeling from the idea that the world these creatures lived in was as remote as any distant star. Paleontology was a grade-school ambition I never followed up on, but the Triassic and Jurassic eras still have a hold on my imagination.
In a SETI context, deep time presents challenges galore. Charles Lineweaver’s work offers up the prospect that the average Earth-like planet in our galactic neighborhood may well be far older than our own — Lineweaver calculates something like an average of 1.8 billion years older. Would a civilization around such a star, if one could survive without destroying itself for so long, have anything it wanted to say to us? Would it have evolved to a level where it had merged so completely with its environment that we might not be able to recognize its artifacts even if we saw them?
All these musings were triggered by the news from the Max-Planck-Institut für Astronomie (Heidelberg) that European astronomers have discovered a planetary system that is truly ancient. The star in question is HIP 11952, about 375 light years from Earth in the constellation Cetus. Not surprisingly, given its estimated age of 12.8 billion years, it is extremely metal poor, but two giant planets are now known to orbit it. Says Johny Setiawan (MPIA), who led this work:
“This is an archaeological find in our own backyard. These planets probably formed when our Galaxy itself was still a baby.”
The planets around HIP 11952, which have orbital periods of 7 and 290 days, thus present us with a challenge. Elements heavier than hydrogen and helium are what astronomers consider ‘metals,’ and we’ve seen through our ongoing analysis of exoplanets that stars with higher metal content are more likely to have planets. We also have a mechanism for metal formation in which heavier elements are produced inside stars and blown into space through the explosion of supernovae. That a star as old as HIP 11952 should have planets would seem to be a rare thing indeed, but we do have another metal-poor system around HIP 13044, discovered back in 2010.
There is no doubt about the low metallicity of HIP 11952, which has an iron abundance approximately 1 percent that of the Sun. This is a population II star, one of the class that includes the oldest and most metal-poor stars that have yet been observed. Our own solar neighborhood is comparatively metal-rich and contains stars younger than 10 billion years. It’s an interesting speculation (followed up in this expanded news release from MPIA) that HIP 11952 may be a remnant from another galaxy that was absorbed by the Milky Way billions of years ago.
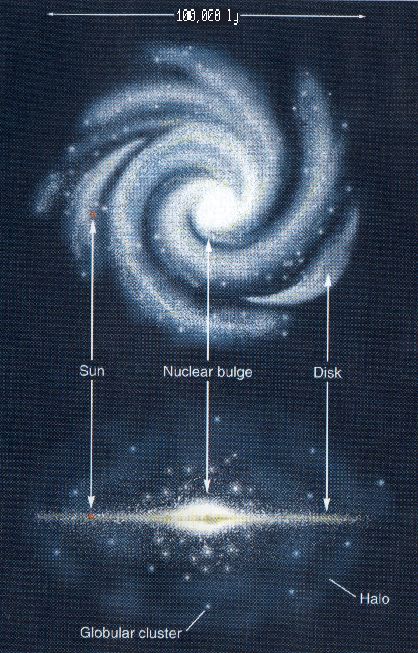
Image: Population II stars tend to have orbits that take them outside the plane of the galactic disk, and are often associated with globular clusters and the galactic halo, a roughly spherical distribution which contains the oldest stars in the Galaxy. Because of their age, most of them consist of almost pure hydrogen and helium, unenriched by earlier generations of stars. Credit: UCSD Center for Astrophysics & Space Sciences.
Are there, then, more planets around metal-poor stars than we have previously thought? We need to find more planetary systems in this age bracket to learn more, but Anna Pasquali (Heidelberg University), a co-author of the paper on this work, says “The discovery of the planets of HIP 11952 shows that planets have been forming throughout the life of our Universe,” a thought that reminds us to be careful about drawing hasty conclusions about planet formation. Don’t be surprised, either, if the idea doesn’t once again bring Dr. Fermi knocking at the door.
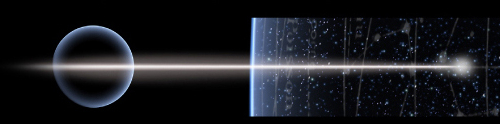
by Paul Gilster | Apr 2, 2012 | Antimatter |
Once when reading Boswell’s monumental life of the 18th Century writer and conversationalist Samuel Johnson, I commented to a friend how surprised I had been to discover that Johnson didn’t spend much time reading in his later years. “He didn’t need a lot of time,” replied my friend, a classics professor. “He tore the heart out of books.” That phrase stuck with me over the years and re-surfaced when I started working with Adam Crowl. More than anyone I know, Adam can get to the heart of a scientific paper and explain its pros and cons while someone like myself is still working through the introduction. And because of his fine work with Project Icarus, I thought Adam would be just the person to explain the latest thinking about a classic concept that Friedwardt Winterberg would like to take to the next level.
by Adam Crowl
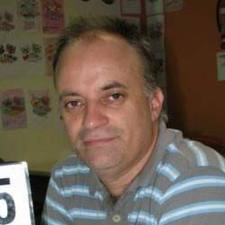
In Jules Verne’s From the Earth to the Moon, the bold Frenchman Michel Ardan, in his first speech to the Baltimore Gun Club, when discussing travelling to the Moon via a cannon-shell, makes the following statement…
Well, the projectile is the vehicle of the future, and the planets themselves are nothing else! Now some of you, gentlemen, may imagine that the velocity we propose to impart to it is extravagant. It is nothing of the kind. All the stars exceed it in rapidity, and the earth herself is at this moment carrying us round the sun at three times as rapid a rate… Is it not evident, then, I ask you, that there will some day appear velocities far greater than these, of which light or electricity will probably be the mechanical agent?
Rockets replaced cannon-shells as the preferred means of interplanetary travel in the early 20th Century, thanks to the work of Tsiolkovksy, Goddard, Oberth and Noordung. They took up Verne’s insight and developed Ardan’s hand-waving further. Applying electricity to rocket motion resulted in the Ion Rocket, and applying light, the Photon Rocket. However the first rocket scientist to propose an engineering solution to how light might be directly harnessed to rocket propulsion, rather than just pushing solar-sails, was Eugen Sänger [1].
Antimatter and the Photon Rocket
Sänger’s discussion of photon rockets showed clearly how difficult it would be – every newton of thrust would require 300 megawatts of photon energy released. Any vehicle generating photons by conventional means would be confined to painfully low accelerations, thus Sänger proposed using matter-antimatter reactions, specifically the mutual annihilation of electrons and positrons, with the resulting gamma-rays (each 0.511 MeV) being reflected by an electron-gas. Unfortunately the electron-gas mirror would need a ridiculously high density, seen only in white-dwarf stars.
The next stage for the matter-antimatter photon rocket saw the work of Robert Forward [2], and more recently Robert Frisbee [3], who applied more modern knowledge of particle physics to the task. Instead of instant and total annihilation of proton-antiproton mixtures, resulting in an explosion of pure high-energy gamma-rays in all directions, the reactions instead produce for a brief time charged fragments of protons, dubbed pions, which can be directed via a magnetic field. According to theoretical analyses by Giovanni Vulpetti [4], in the 1980s, and more recently by Shawn Westmoreland [5], the theoretical top performance of a pion rocket is a specific impulse equivalent to 0.58c. However the pion rocket isn’t strictly a pure photon rocket and suffers from the inefficiency of magnetic nozzles. Simulations by John Callas [6] at JPL, in the late 1980s suggested an effective exhaust velocity of ~1/3 the speed of light could be achieved.
The other difficulty of matter-antimatter propulsion, as graphically illustrated by Frisbee’s work, is the extreme difficulty of storing antimatter. The old concept of storing it as plasma is presently seen as too power intensive and too low in density. Newer understanding of the stability of frozen hydrogen and its paramagnetic properties has led to the concept of magnetically levitating snowballs of anti-hydrogen at the phenomenally low 0.01 K. This should mean a near-zero vapour pressure and minimal loses to annihilation of the frozen antimatter. What it also means is immensely long and thin spacecraft designs. Frisbee’s conceptual designs are literally the size of planets, thousands of kilometres long, but merely metres wide. This minimises the gamma-radiation exposure of heat-sensitive components and maximises the exposure of radiators to the cosmic heat-sink. To achieve 0.5c, using known materials, results in vehicles massing millions of tonnes [3].
Harvesting the Fire
Friedwardt Winterberg’s recent preprint [7] suggests a different concept, with the promise of near total annihilation and near perfect collimation of a pure gamma-ray exhaust. Poul Anderson described such a vehicle’s operation in fiction in his Harvest the Fire (1995), describing an advanced matter-antimatter rocket – the exhaust was so efficiently directed that it was invisible for thousands of kilometres before finally appearing as a trail of scattered energy. So what is Winterberg proposing?
We’ve encountered Winterberg’s work before [8] in Centauri Dreams in his designs for deuterium fusion rockets, and his new work is an outgrowth of his work on the magnetic collapse of ions into incredibly dense states. Using the technique he describes, high compression of fusion plasma can be achieved, but in the case of a matter-antimatter ambiplasma (a plasma that is an even mix of the two) the result is even more spectacular.
Essentially what Winterberg describes is generating a very high electron-positron current in the ambiplasma, while leaving the protons-antiprotons with a low energy. This high current generates a magnetic field that constricts rapidly, a so-called pinch discharge, but because it is a matter-antimatter mix it can collapse to a much denser state. Near nuclear densities can be achieved, assuming near-term technical advancements to currents of 170 kA and electron-positron energies of 1 GeV. This causes intensely rapid annihilation that crowds the annihilating particles into one particular reaction pathway, directly into gamma-rays, pushing them to form a gamma-ray laser. By constricting the annihilating particles into this state a very coherent and directional beam of gamma-rays is produced, the back-reaction of which pushes against the annihilation chamber’s magnetic fields, providing thrust.
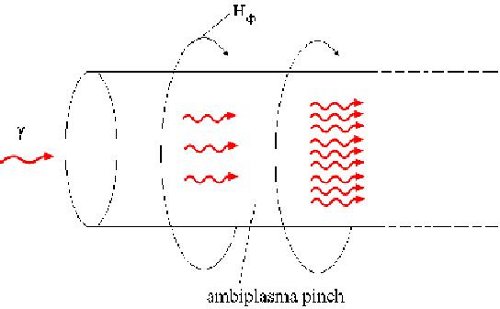
Figure 1. [from Ref.7] Gamma-ray Laser
The figure above depicts the processes involved – the magnetic field of the ambiplasma (from the electron-positron current) squeezes a linear atom of protons-antiprotons which begin annihilating, stimulating more annihilation, all in one direction from the annihilation being triggered at one end of the discharge. Thanks to the very confined channel created by the magnetic pinch, the laser beam produced has very limited spread. Intense magnetic-field recoil is created by the firing of the gamma-beam, with a pulsed field-strength of 34 tesla. The recoil force can thus be transferred back to the vehicle by the right choice of conductor surrounding the reaction chamber.
Winterberg ends his paper with an anecdote about Edward Teller, one of the many fathers of the H-bomb, who was of the opinion that photon rockets would eventually be possible – in “500 years” which equates to “impossible” in the minds of the short-sighted. Certainly making antimatter efficiently will be a Herculean task, as the energy requirements are immense. Storing it is equally “impossible”. However, as Winterberg notes, there might be a quicker pathway to confinement.
Over the last decade researchers at the University of Gothenburg, led by Leif Holmlid, have been studying exotic states of deuterium. In the past two years they have reported [9] an ultra-dense state, which has also been independently computed [10] to form inside low-mass brown-dwarf stars. This exotic quantum liquid is one million times denser than liquid deuterium and apparently a superconducting superfluid at room-temperature. Only minute amounts have been made and studied so far, but such a material could be able to sustain intense magnetic fields, up to 100,000 tesla. If it can be manufactured in large amounts, and is stable in intense magnetic fields, then the problem of magnetic confinement of anti-hydrogen at friendlier temperatures becomes more tractable.
To quote Winterberg [7], paraphrasing Teller…
Therefore, if nature is kind to us, the goal for a relativistic photon rocket might be closer than the 500 years prophesized by Teller.
References
[1] E. Sänger, 4th International Astronautical Congress, Zürich, Switzerland 3-8 August 1953.
[2] R.L. Forward, “Antiproton Annihilation Propulsion”, AFRPL TR-85-034, (1985)
[3] G. Vulpetti, “Maximum terminal velocity of relativistic rocket,” Acta Astronautica, Vol. 12, No. 2, 1985, pp. 81-90.
[4] S. Westmoreland, “A note on relativistic rocketry,” Acta Astronautica, Volume 67, Issues 9-10, November-December 2010, pp. 1248 – 1251.
[5] J.L. Callas,”The Application of Monte Carlo Modeling to Matter-Antimatter Annihilation Propulsion Concepts,” JPL Internal Document D-6830, October 1, 1989.
[6] R. H. Frisbee, 39th AIAA/ASME/SAE/ASEE Joint Propulsion Conference, Huntsville, AL, July 2003, AIAA-2003-4676
[7] F. Winterberg, “Matter-Antimatter GeV Gamma Ray Laser Rocket Propulsion”, 2011 (preprint).
[8] F. Winterberg, “Advanced Deuterium Fusion Rocket Propulsion For Manned Deep Space Missions”, JBIS Vol.62 No 11/12 (2009).
[9] P.U. Andersson and L. Holmlid, “Superfluid ultra-dense deuterium D(-1) at room temperature”. Phys. Lett. A 375 (2011) 1344-1347. doi:10.1016/j.physleta.2011.01.035.
[10] L.Berezhiani, G.Gabadadze and D.Pirtskhalava, “Field Theory for a Deuteron Quantum Liquid”, JHEP 1004, 122 (2010). Preprint available.
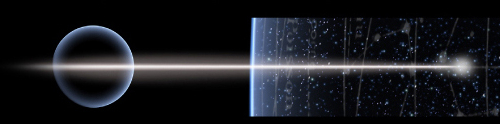
by Paul Gilster | Apr 2, 2012 | Administrative |
Last week I reported on information from a source on the WISE mission that no new red dwarfs had yet been discovered out to a distance of 10 light years. This past weekend I received an email from my source apologizing for mis-typing. He had meant to say no brown dwarfs — not red dwarfs — out to a distance of 10 light years. And as I mentioned with the earlier post, the data analysis continues and there may be surprises yet to come.
A nearby brown dwarf is something I’ve been writing about here for some time, pondering its implications and wondering whether one might actually turn up that was closer than the Alpha Centauri stars. So the news is that no brown dwarfs matching the description have yet turned up, but the hunt continues.
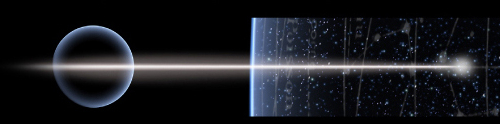