by Paul Gilster | Sep 13, 2023 | Missions |
If we’re going to get to the stars, the path along the way has to go through an effort like Breakthrough Starshot. This is not to say that Breakthrough will achieve an interstellar mission, though its aspirational goal of reaching a nearby star like Proxima Centauri with a flight time of 20 years is one that takes the breath away. But aspirations are just that, and the point is, we need them no matter how far-fetched they seem to drive our ambition, sharpen our perspective and widen our analysis. Whether we achieve them in their initial formulation cannot be known until we try.
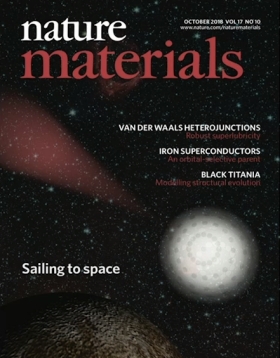
So let’s talk for a minute about what Starshot is and isn’t. It is not an attempt to use existing technologies to begin building a starship today. Yes, metal is being bent, but in laboratory experiments and simulated environments. No, rather than a construction project, Starshot is about clarifying where we are now, and projecting where we can expect to be within a reasonable time frame. In its early stages, it is about identifying the science issues that would enable us to use laser beaming to light up a sail and push it toward another star with prospects of a solid data return. Starshot’s Harry Atwater (Caltech) told the Interstellar Research Group in Montreal that it is about development and definition. Develop the physics, define and grow the design concepts, and nurture a scientific community. These are the necessary and current preliminaries.
Image: The cover image of a Starshot paper illustrating Harry Atwater’s “Materials Challenges for the Starshot Lightsail,” Nature Materials 17 (2018), 861-867.
We’re talking about what could be a decades-long effort here, one that has already achieved a singular advance in interstellar studies. I don’t have the current count on how many papers have been spawned by this effort, but we can contrast the ongoing work of Starshot’s technical teams with where interstellar studies was just 25 years ago, when few scientific conferences dealt with interstellar ideas and exoplanets were still a field in their infancy. In terms of bringing focus to the issue, Starshot is sui generis.
It is also an organic effort. Starshot will assess its development as it goes, and the more feasible its answers, the more it will grow. I think that learning more about sail possibilities will spawn renewed effort in other areas, and I see the recent growth of fusion rocketry concepts as a demonstration that our field is attaining critical mass not only in the research labs and academy but in commercial space ventures as well.
So let’s add to Atwater’s statement that Starshot is also a cultural phenomenon. Although its technical meetings are anything but media fodder, their quiet work keeps the idea of an interstellar crossing in the public mind as a kind of background musical riff. Yes, we’re thinking about this. We’ve got ideas and lab experiments that point to new directions. We’re learning things about lightsails and beaming we didn’t know before. And yes, it’s a big universe, with approximately one planet per star on average, and we’ve got one outstanding example of a habitable zone planet right next door.
So might Starshot’s proponents say to themselves, although I have no idea how many of those participating in the effort back out sometimes to see that broader picture (I suspect quite a few, based on those I know, but I can’t speak for everyone). But because Starshot has not sought the kind of publicity that our media-crazed age demands, I want to send you to Atwater’s video presentation at Montreal to get caught up on where things stand. I doubt we’re ever going to fly the mission Starshot originally conceived because of cost and sheer scale, but I’m only an outsider looking in. I do think that when the first interstellar mission flies, it will draw heavily on Starshot’s work. And this will be true no matter what final choices emerge as to propulsion.
This is a highly technical talk compressed into an all too short 40 minutes, but let’s just go deep on one aspect of it, the discussion of the lightsail that would be accelerated to 20 percent of lightspeed for the interstellar crossing. Atwater’s charts are worth seeing, especially the background on what the sail team’s meetings have produced in terms of their work on sail materials and, especially, sail shape and stability. The sail is a structure approximately 4 meters in diameter, with a communications aperture 1 meter in size, as seen in the center of the image (2 on the figure). Surrounding it on the circular surface are image sensors (6) and thin-film radioisotope power cells (5).
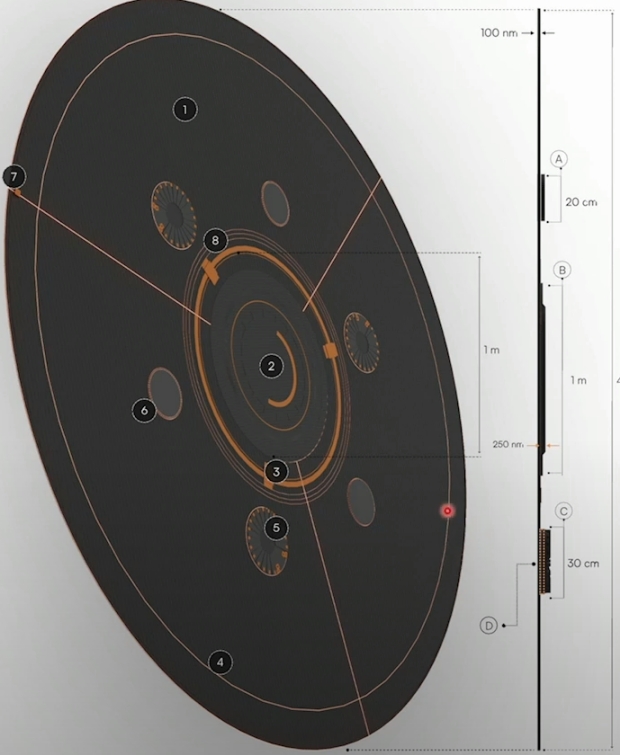
Maneuvering LEDs (4) provide attitude control, and thin-film magnetometers (7) are in the central disk, with power and data buses (8) also illustrated. A key component: A laser reflector layer positioned between the instruments that are located on the lightsail and the lightsail itself, which is formed as a silicon nitride metagrating. As Atwater covers early in his presentation, the metagrating is crucial for attitude control and beam-riding, keeping the sail from slipping off the beam even though it is flat. The layering is crucial in protecting the sailcraft instrumentation during the acceleration stage, when it is fully illuminated by the laser from the ground.
How to design lensless transmitters and imaging apertures? Atwater said that lensless color camera and steerable phased array communication apertures are being prototyped in the laboratory now using phased arrays with electrooptic materials. Working one-dimensional devices have emerged in this early work for beam steering and electronic focusing of beams. The laser reflector layer offers the requisite high reflectivity at the laser wavelength being considered, using a hybrid design with silicon nitride and molybdenum disulfide to minimize absorption that would heat the sail.
I won’t walk us through all of the Starshot design concepts at this kind of detail, but rather send you to Atwater’s presentation, which shows the beam-riding lightsail structure and its current laboratory iterations. The discussion of power sources is particularly interesting given the thin-film lightweight structures involved, and as shown in the image below, it involves radioisotope thermoelectric generators actually integrated into the sail surface. Thin film batteries and fuel cells were considered by Breakthrough’s power working group but rejected in favor of this RTG design.
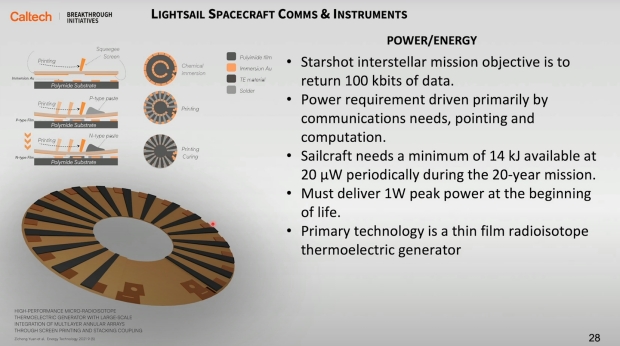
So much is going on here in terms of the selection of sail materials and the analysis of its shape, but I’ll also send you to Atwater’s presentation with a recommendation to linger over his discussion of the photon engine, that vast installation needed to produce the beam that would make the interstellar mission happen. The concept in its entirety is breathtaking. The photon engine is currently envisioned as an array of 1,767,146 panels consisting of 706,858,400 individual tiles (Atwater dryly described this as “a large number of tiles”), producing the 200 gW output and covering 3 kilometers on the ground. The communications problem for data return is managed by scalable large-area ground receiver arrays, another area where Breakthrough is examining cost trends that within the decades contemplated for the project will drive component expenses sharply down. The project depends upon these economic outcomes.
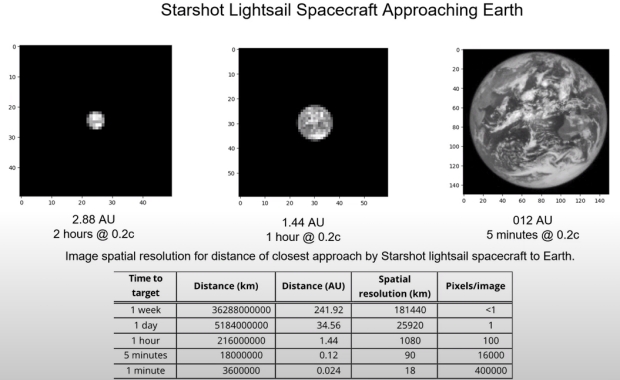
Image: What we would see if we had a Starshot-class sailcraft approaching the Earth, from the image at two hours away to within five minutes of its approach. Credit for this and the two earlier images: Harry Atwater/Breakthrough Starshot.
Using a laser-beamed sail technology to reach the nearest stars may be the fastest way to get images like those above. The prospect of studying a planet like Proxima b at this level of detail is enticing, but how far can we count on economic projections to bring costs down to the even remotely foreseeable range? We also have to factor in the possibility of getting still better images from a mission to the solar gravitational lens (much closer) of the kind currently being developed at the Jet Propulsion Laboratory.
Economic feasibility is inescapably part of the Starshot project, and is clearly one of the fundamental issues it was designed to address. I return to my initial point. Identifying the principles involved and defining the best concepts to drive design both now and in the future is the work of a growing scientific community, which the Starshot effort continues to energize. That in itself is no small achievement.
It is, in fact, a key building block in the scientific edifice that will define the best options for achieving the interstellar dream. And while this is not the place to go into the complexities of scientific funding, suffice it to say that putting out the cash to enable these continuing studies is a catalytic gift to a field that has always struggled for traction both financial and philosophical. The Starshot initiative has a foundational role in defining the best technologies for interstellar flight that will lead one day to its realization.
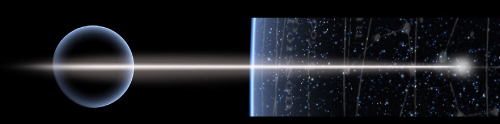
by Paul Gilster | May 14, 2019 | Missions |
Recent tests of a ‘wafer-craft’, an early prototype for what may one day be the ‘starchip’ envisioned by scientists involved with the Breakthrough Starshot project, have been successful. The work grows out of a NASA-funded effort led by Philip Lubin (UC Santa Barbara), whose investigations into large scale directed energy systems began in 2009. Lubin went on to perform multiple studies for NASA’s Innovative Advanced Concepts program developing the idea that would become known as DEEP-IN (Directed Energy Propulsion for Interstellar Exploration). His NIAC Phase 1 report studied as one option beamed propulsion driving a wafer-scale spacecraft.
Renamed Starlight, the proposal went on to Phase II funding as well as support from the private sector. A subsequent review by Breakthrough Initiatives led to endorsement of the concept within its Breakthrough Starshot effort. Breakthrough is devoting $100 million to studying the viability of sending a ‘starchip’ to a nearby star such as Proxima Centauri, a mission that, moving at 20 percent of lightspeed through laser-beamed propulsion, would arrive at its target within 20 years as opposed to the tens of thousands of years required for chemical propulsion.
But back to that prototype wafer-scale spacecraft, whose launch was conducted in collaboration with the United States Naval Academy. The craft rose into the stratosphere above Pennsylvania via balloon on April 12, 2019 — the 50th anniversary of the Gagarin flight — reaching an altitude of 32 kilometers. The test is part of what Lubin calls “a long-term program to develop miniature spacecraft for interplanetary and eventually for interstellar flight.”
Nic Rupert, a development engineer at UC Santa Barbara, describes the wafer-craft in its current incarnation:
“It was designed to have many of the functions of much larger spacecraft, such as imaging, data transmission, including laser communications, attitude determination and magnetic field sensing. Due to the rapid advancements in microelectronics we can shrink a spacecraft into a much smaller format than has been done before for specialized applications such as ours.”
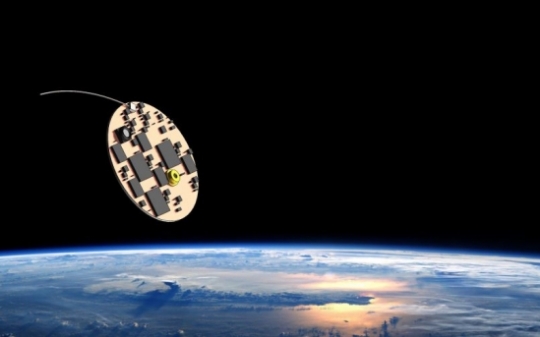
Image: An artist’s concept of the wafer-craft. Credit: UC Santa Barbara.
The good news is that the chip performed exceptionally well, returning over 4,000 images of Earth by way of testing what may eventually emerge as a space technology that could turn interstellar. The process is iterative, working with off-the-shelf components that can be pushed to increasingly difficult conditions that will test the wafer-craft’s viability under extreme conditions of temperature and radiation, as well as its potential to survive impact with dust particles.
In other words, to get to ‘starchips,’ we must first get to ‘spacechips,’ and that begins with balloon flights well within the atmosphere to shake out early data on performance. The goal: A one-gram chip that contains within itself a functional spacecraft. At UC-Santa Barbara, an undergraduate group drawing on students from physics, engineering, chemistry and biology is conducting the balloon flights that may result in future, mass-produced interstellar probes.
This news release from UC-Santa Barbara notes that the ramping up of testing points to a suborbital flight next year. Early applications of the technology should involve missions closer to home; indeed, a laser beaming infrastructure would have applications for fast interplanetary travel as well as planetary defense against asteroids and other space debris. Thus testing funded by NASA and private foundations examines the viability of miniaturized spacecraft that, given the beaming resources, could one day give us a close-up look at Proxima Centauri b.
For more on the Starlight effort, visit its website.
Tomorrow I’ll keep the focus on fast interstellar missions in the form of some interesting ideas from a paper by Bing Zhang, an astrophysicist from the University of Nevada, on what he is calling ‘relativistic astronomy’ and its possibilities on the way to distant destinations.
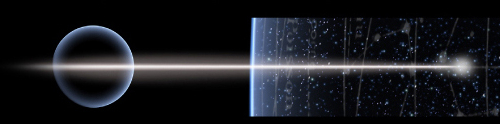
by Paul Gilster | Oct 5, 2018 | Missions |
Last Monday’s article on the Trillion Planet Survey led to an email conversation with Phil Lubin, its founder, in which the topic of Breakthrough Starshot invariably came up. When I’ve spoken to Dr. Lubin before, it’s been at meetings related to Starshot or presentations on his DE-STAR concept. Standing for Directed Energy System for Targeting of Asteroids and exploRation, DE-STAR is a phased laser array that could drive a small payload to high velocities. We’ve often looked in these pages at the rich history of beamed propulsion, but how did the DE-STAR concept evolve in Lubin’s work for NASA’s Innovative Advanced Concepts office, and what was the path that led it to the Breakthrough Starshot team?
The timeline below gives the answer, and it’s timely because a number of readers have asked me about this connection. Dr. Lubin is a professor of physics at UC-Santa Barbara whose primary research beyond DE-STAR has involved the early universe in millimeter wavelength bands, and a co-investigator on the Planck mission with more than 400 papers to his credit. He is co-recipient of the 2006 Gruber Prize in Cosmology along with the COBE science team for their groundbreaking work in cosmology. Below, he tells us how DE-STAR emerged.
By Philip Lubin
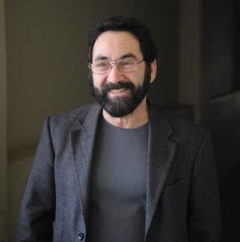
June 2009: Philip Lubin begins work on large scale directed energy systems at UC Santa Barbara. Baseline developed is laser phased array using MOPA [master oscillator power amplifier, a configuration consisting of a master laser (or seed laser) and an optical amplifier to boost the output power] topology. The DE system using this topology is named DE-STAR (Directed Energy System for Targeting Asteroids and exploRation). Initial focus is on planetary defense and relativistic propulsion. Development program begins. More than 250 students involved in DE R&D at UCSB since.
February 14, 2013: UCSB group has press release about DE-STAR program to generate public discussion about applications of DE to planetary defense in anticipation of February 15 asteroid 2012 DA14, which was to come within geosync orbit. On February 15 Chelyabinsk meteor/asteroid hit. This singular coincidence of press release and hit the next day generated a significant change in interest in possible use of large scale DE for space applications. This “pushed the DE ball over the hill”.
August 2013: Philip Lubin and group begin publication of detailed technical papers in multiple journals. DE-STAR program is introduced at invited SPIE [Society of Photo-optical Instrumentation Engineers] plenary talk in San Diego at Annual Photonics meeting. More than 50 technical papers and nearly 100 colloquia from his group have emerged since then. List of DE-STAR papers can be found here.
August 2013: 1st Interstellar Congress held in Dallas, Texas by Icarus Interstellar. Eric Malroy introduces concepts for the use of nanomaterials in sails.
August 2013: First proposal submitted to NASA for DE-STAR system from UC Santa Barbara.
January 2014: Work begins on extending previous UCSB paper to much longer “roadmap” paper which becomes “A Roadmap to Interstellar Flight” (see below).
February 11, 2014 – Lubin gives colloquium on DE-STAR at the SETI Institute in Mountain View, CA. Summarizes UCSB DE program for planetary defense, relativistic propulsion and implications for SETI. SETI Institute researchers suggest Lubin speak with NASA Ames director Pete Worden as he was not at the talk. Worden eventually leaves Ames a year later on March 31, 2015 to go to the Breakthrough Foundation. Lubin and Worden do not meet until 18 months later at the Santa Clara 100YSS meeting (see below).
August 2014: Second proposal submitted to NASA for DE-STAR driven relativistic spacecraft. Known as DEEP-IN (Directed Energy Propulsion for Interstellar Exploration). Accepted and funded by NASA NIAC program as Phase I program. Program includes directed energy phased array driving wafer scale spacecraft as one option [Phase 1 report “A Roadmap to Interstellar Flight” available here].
April 2015: Lubin submits the “roadmap” paper to the Journal of the British Interplanetary Society.
June 2015: Lubin presents DE driven relativistic flight at Caltech Keck Institute meeting. Meets with Emmett and Glady W Technology Fund.
August 31, 2015: August 31, 2015: Lubin and Pete Worden attend 100YSS (100 Year Star Ship) conference in Santa Clara, CA [Worden is now executive director, Breakthrough Starshot, and former director of NASA Ames Research Center]. Lubin is invited by Mae Jemison (director of 100YSS) to give a talk about the UCSB NASA DE program as a viable path to interstellar flight. Worden has to leave before Lubin’s talk, but in a hallway meeting Lubin informs Worden of the UCSB NASA Phase I NASA program for DE driven relativistic flight. This meeting takes places as Lubin recalls Feb 2014 SETI meeting where a discussion with Worden is suggested. Worden asks for further information about the NASA program and Lubin sends Worden the paper “A Roadmap to Interstellar Flight” summarizing the NASA DEEP-IN program. Worden subsequently forwards paper to Yuri Milner.
December 16, 2015: Lubin, Worden and Pete Klupar [chief engineer at Breakthrough Prize Foundation] meet at NASA Ames to discuss DEEP-IN program and “roadmap” paper.
December 2015: Milner calls for meeting with Lubin to discuss DEEP-IN program, “roadmap” paper and the prospects for relativistic flight.
January 2016: Private sector funding of UCSB DE for relativistic flight effort by Emmett and Glady W Technology Fund begins. Unknown to public – anonymous investor greatly enhances UCSB DE effort.
January 2016: First meeting with Milner in Palo Alto. Present are Lubin, Milner, Avi Loeb (Harvard University), Worden and Klupar. Milner sends “roadmap” paper to be reviewed by other physicists. A long series of calls and meetings ensue. This begins the birth of Breakthrough Starshot program.
March 2016 – NASA Phase II proposal for DEEP-IN submitted. Renamed Starlight subsequently. Accepted and funded by NASA.
March 2016: After multiple reviews of Lubin “roadmap” paper by independent scientists, Breakthrough Initiatives endorses idea of DE driven relativistic flight.
April 12, 2016: Public release of Breakthrough Starshot. Hawking endorses idea at NY public announcement.
To keep up with developments, the following websites are useful:
NASA Starlight (DE-STAR for interstellar relativistic flight):
http://www.deepspace.ucsb.edu/projects/starlight
Planetary Defense Application of DE-STAR:
http://www.deepspace.ucsb.edu/projects/directed-energy-planetary-defense
Implications for SETI:
http://www.deepspace.ucsb.edu/projects/implications-of-directed-energy-for-seti
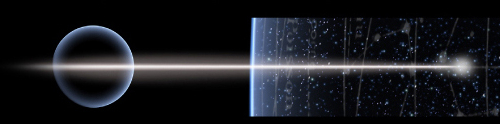
by Paul Gilster | Aug 17, 2018 | Missions |
When we think about what is usually called ‘planetary protection,’ we’re talking about the probes we send to possibly life-bearing places like Mars or Europa. It would confound our investigations if we couldn’t be sure we hadn’t contaminated such a place with microorganisms from Earth, unwittingly carried aboard a lander that was not properly stripped of such passengers. Even our Cassini Saturn orbiter was guided into the planet as a way of ensuring that it would not, at some future date, crash into a place as biologically interesting as Enceladus.
Yesterday, having looked at an essay by Ethan Siegel, I asked rhetorically whether we should think up some kind of exoplanetary protection policy as well. After all, we’re fleshing out an actual mission design through Breakthrough Starshot, aiming to reach nearby stars in coming decades. Siegel (Lewis & Clark College) had expressed his concern that Breakthrough Starshot might inadvertently start an interstellar war. The idea is extreme, but I use the word ‘war’ because it was right there in the title of Siegel’s piece.
What Siegel worries about is that among the large number of payloads Breakthrough Starshot would like to send to nearby stars there might be one that accidentally impacted the planet under study. He’s concerned about the ‘cone of uncertainty’ that would be involved in any trajectory aimed at making a close pass of a planet like Proxima b and argues that at 60,000 km/sec, which is the Starshot goal of 20 percent of c, a tiny 1-gram payload will strike with the force of a 1 tonne asteroid moving at 60 km/sec, a hit like Chelyabinsk.
Harvard’s Avi Loeb, who chairs the Breakthrough Starshot advisory board, finds little merit in the concern. I had been wondering as I wrote yesterday’s post just what Dr. Loeb would have to say about Siegel’s views, only to find an email with a link to his new essay in Forbes in my mailbox shortly after I published — his piece had already appeared that morning. Loeb points out that at a tenth of the speed of light, a payload of Starshot size would “…merely carry the energy of a common asteroid, only a few meters in size — of order the height of a person. Such asteroids hit the Earth a few times per year and burn up in the atmosphere.”
Here is a map of asteroid impacts that Loeb linked to in his essay.
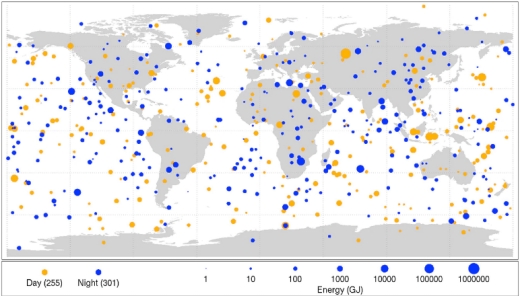
Image: This diagram maps the data gathered from 1994-2013 on small asteroids impacting Earth’s atmosphere to create very bright meteors, technically called “bolides” and commonly referred to as “fireballs”. Sizes of red dots (daytime impacts) and blue dots (nighttime impacts) are proportional to the optical radiated energy of impacts measured in billions of Joules (GJ) of energy, and show the location of impacts from objects about 1 meter (3 feet) to almost 20 meters (60 feet) in size. Credit: Planetary Science.
The map, the work of NASA’s Near Earth Object Program, is instructive. All told, 556 small asteroids impacted our atmosphere in a 20-year period, almost all of them disintegrating in the atmosphere, with the obvious exception of the Chelyabinsk event, which was caused by the largest asteroid to strike in this timeframe. My understanding about Chelyabinsk is that the meteor was approximately 20 meters in size, moving at roughly 19 kilometers per second.
But wouldn’t a one-gram payload moving at Starshot speeds still wreak havoc if it fell on a planetary surface? Evidently the event wouldn’t happen. Let me quote Loeb on this:
…a gram-scale starchip would burn up in the atmosphere of a planet much more easily than a tonne-mass asteroid. Its impact on a planet would be no more irritating than the impact of a dust grain on the skin of a grazing cow. The interplanetary medium is full of debris that poses far greater risks to a planet, as the dinosaurs realized when wiped out by the impact of a 10-15 kilometer asteroid (a trillion times more massive than we just considered) some sixty five million years ago.
In any case, Loeb sees the chances of collision at something less than one part in a million, given the fact that knowing the relative positions of planet and spacecraft over a journey lasting decades is impossible with the payload as envisioned. What Breakthrough Starshot does expect is a closest approach thousands of times larger than the surface of any planet.
We should pause on the deceleration question, which comes up repeatedly whenever I talk about Starshot probes with people new to the project. While it is certainly true that deceleration and orbit in, say, the Proxima Centauri system would be a huge benefit, there is no way to make it happen given the current constraints on the payload, which relies on miniaturization and nonetheless requires vast energies to be brought up to cruising speed. Our initial interstellar efforts will surely be flybys for this reason, and there is a wealth of science that can be gathered.
The other thing to be remembered is that Breakthrough Starshot is an ongoing effort to demonstrate the feasibility of beamed laser sail technologies. Submissions from the first Requests for Proposals are being evaluated as sail stability and materials are considered by way of moving toward experimental work in what we can hope will be a dedicated sail facility. The laser array that would drive these craft presents huge challenges of its own, as do the many other issues, like communications, that will have to be resolved before any interstellar mission can be flown.
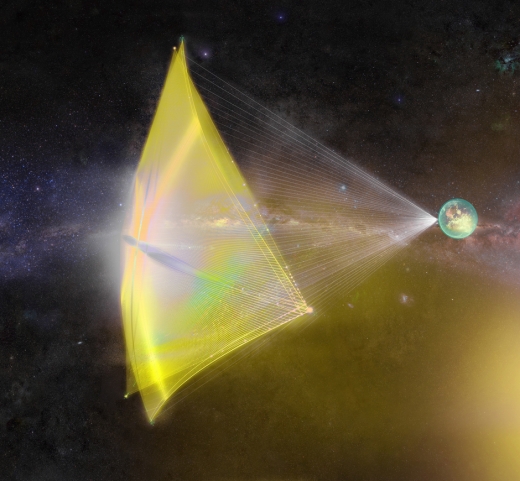
We’re looking at an interstellar capability that, if everything were to go well, would still take 30 years to produce a working infrastructure. To those new to Breakthrough Starshot, I recommend looking at the list of technical problems that face the project as it determines whether the concept is sound enough to move to prototype stage enroute to a full deployment. This is going to take time, and we won’t know for quite a while whether the Starshot concept, as is, is workable.
What we do already know is that the commitment of resources underlying the project is already paying off, and will continue to pay off, in the form of newly funded research.
Anyone familiar with the history of interstellar flight studies will know how rare it is to have this level of cooperation and participation among the scientists best equipped to tackle the problem. For most of the past century, researchers interested in the interstellar conundrum did their work in their spare time and met only at the occasional conference, with infrequent journal articles maintaining the thread. The research Breakthrough Starshot will produce will thus be a solid advance for the entire field, no matter if, when or how often the actual missions are flown.
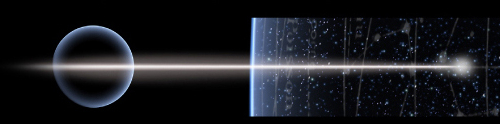
by Paul Gilster | Jun 4, 2018 | Missions |
Breakthrough Starshot held an ‘industry day’ on Wednesday May 23rd devoted to its lightsail project to take nanocraft to another star, framing the release of a Request for Proposals during its early concepts and analysis phase. The RFP focuses on the sail itself, investigating sail materials and stability under thrust. Step A proposals are due June 22, step B proposals on July 10, with finalists to be notified and contracts awarded this summer. The intent of the RFP is laid out in documents and slides from the meeting that Breakthrough has now placed online.
From the RFP itself:
The scope of this RFP addresses the Technology Development phase – to explore LightSail concepts, materials, fabrication and measurement methods, with accompanying analysis and simulation that creates advances toward a viable path to a scalable and ultimately deployable LightSail.
We’ve been talking about Breakthrough Starshot in these pages for a long time, as a search through the archives will reveal. The intention is to send gram-scale probes commonly referred to as ‘starchips’ attached to sails on the scale of meters to a nearby star to investigate its planets, with the highly interesting Proxima Centauri b an obvious target given that it is also the closest star to the Sun. Propelling the sail will be a gigawatt-scale ground-based laser. 20 years of flight time sets up the flyby, with data transmission returning images of the star system.
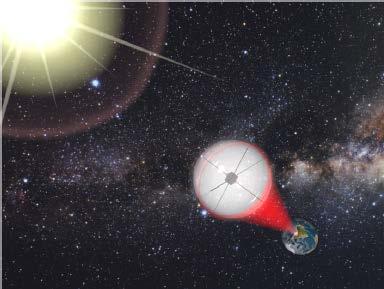
$100 million in research and development is to be spent over the next 5 years to determine the feasibility of both laser and sail. Overall, the five-year technology development period will be managed by three groups: Starshot’s sail committee, a photon engine committee and a systems engineering committee. After that, the goal from years 6 to 11 is to build a low-power prototype for space testing. After that comes a full scale laser system (called the ‘photon engine’) over the next 20 years, with launch of an interstellar mission occurring in approximately 30 years.
It’s an ambitious schedule, to be sure, but the early conceptual steps are now being taken, with initial sail work investigating candidate materials and sail stability. Keeping a sail stable under the high accelerations induced by the laser array is obviously critical and has already involved discussions and papers on different sail configurations, while the equally critical issue of sail materials is likewise considered in the RFP, which is Phase 1 of the sail’s development.
Phase 2 of the technology development program for the lightsail will validate lightsail materials and stable designs by proof of concept laboratory demonstrations, while Phase 3 takes us into laboratory testing of scalable prototypes. All of this points toward the eventual goal of a prototype mission that would launch nanocraft to a target here in the Solar System.
With this RFP focusing on Phase 1, Breakthrough Starshot seeks proposals for quantitative models that can produce testable predictions of sail performance, mathematical models defining the necessary conditions for sail stability, experimental methods for lightsail material fabrication and precision measurements to ‘validate optical, thermal and mechanical stability of materials.’ Here is the RFP statement of key issues involved in identifying candidate materials and designs:
- Design of a reflector consistent with the mission requirement of achieving 0.2c for ~1g payload and LightSail area of >1 m2
- Design of passive, adaptive or active features that enable or enhance stability, damage resistance, thermal robustness, and durability under deformation
- Assessment of candidate materials (including thin-films, micro/nanopatterned structures, 2D materials) for thermal/mechanical stability
- Development of measurement techniques and protocols for LightSail material properties (absorption, reflectivity, temperature, stress state, etc.)
- Identification of materials for which scale-up and manufacture at the >1 m2 scale is feasible
- Materials that facilitate integration of the LightSail with the Starchip
- Development of the next generation Starchip scale spacecraft with a path towards incorporation into the Nanocraft
The second objective of the RFP is to identify and assess optimally-shaped designs for a stable sail that can withstand the temperatures and accelerations involved in pushing nanoncraft to 20 percent of the speed of light in a matter of minutes. The RFP notes the key issues here as:
- Validation via, e.g., multi-physics simulation (optical, mechanical, thermal, etc.) of LightSail durability and dynamic stability and sensitivity to Photon Engine laser propulsion beam geometry and ground demonstrations
- Evaluation of spacecraft stability in the context of a LightSail integrated with a Starchip payload
- Development of optimization-based tools for evaluating LightSail designs matched to corresponding laser beam profiles.
- Defining a roadmap for test and verification, including:
o Measurement techniques for thin membranes at a small (<1 cm2) scale
o Developing diagnostics and instrumentation needed for LightSail stability
measurements
You can download the RFP from the Breakthrough Starshot site for bidding information; note that multiple awards are anticipated. A layout of the proposal process and requirements is provided there, with submission information. The procurement is a two-step process with initial (short) white paper proposal evaluated by the Starshot lightsail committee and experts in beamed propulsion, and a second round in which finalists are invited to make final proposals. Contract negotiations will then be performed by the Starshot lightsail committee.
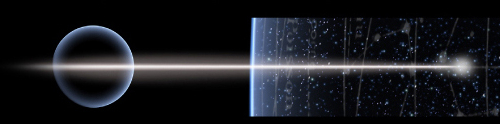