by Paul Gilster | Mar 25, 2009 | Deep Sky Astronomy & Telescopes |
I, for one, would like to be in on the detection of gravitational waves. They flow naturally from the theory of General Relativity and ought to be out there, but none have ever been directly detected. What might make finding them easier would be a spectacular event, such as the merger of a pulsar and a neutron star or a black hole, an event that should cause a huge emission of gamma rays in its final moments. Short-period binaries are the ticket — find them and you have the chance to test General Relativity to high degrees of precision.
Some 200,000 volunteers have already signed up for the EINSTEIN@Home project, which searches for gravitational waves from rapidly spinning neutron stars. The project is now looking for volunteers for its new search, one that will use home computers to analyze data gathered at the Arecibo Observatory in Puerto Rico in the hunt for binary radio pulsars.
This is jazzy stuff, another opportunity, like SETI@Home and the Galaxy Zoo, for those of us with an astronomical bent to deploy our computing cycles productively. And what could be more exotic? Gravitational wave detection takes us into fundamental questions about the physics of gravity and may offer new tools for astronomical observations. Think of these waves as ripples in spacetime emitted by accelerating masses, an analog to the way accelerating charges produce electromagnetic waves.
The Laser Interferometer Gravitational-Wave Observatory (LIGO) offers four concrete goals that could be the result of current investigations:
- Verify directly General Relativity’s prediction that gravitational waves exist.
- Test General Relativity’s prediction that these waves propagate at the same speed as light, and that the graviton (the fundamental particle that accompanies these waves) has zero rest mass.
- Test General Relativity’s prediction that the forces the waves exert on matter are perpendicular to the waves’ direction of travel, and stretch matter along one perpendicular direction while squeezing it along the other; and also, thereby, test General Relativity’s prediction that the graviton has twice the rate of spin as the photon.
- Firmly verify that black holes exist, and test General Relativity’s predictions for the violently pulsating space-time curvature accompanying the collision of two black holes. This will be the most stringent test ever of Einstein’s General Relativity theory.
We’ve come to realize how effective distributed computing can be. A Cornell University news release points out that current searches of radio data are ineffective for pulsars in binary systems with orbital periods less than fifty minutes. The EINSTEIN@Home project will put enough computational power onto the job that we can begin to detect systems with much shorter periods, down to a brief eleven minutes. We’ll wind up with better estimates of binary system formation and disappearance in such scenarios, along with precisely identified targets for gravitational wave detectors.
From a propulsion standpoint, what intrigues us is that a deeper understanding of gravity and its coupling with electromagnetism could point to a theoretical basis for manipulating inertia or gravity itself. Mass, after all, warps the spacetime against which electromagnetism is measured. Now we’ve moved a long way from the original gravitational wave detection, and haven’t begun to get into the question of energy requirements that could render such thoughts chimerical, but finding gravitational waves takes us deeper into this force’s rich mysteries.
by Paul Gilster | Mar 24, 2009 | Culture and Society, Sail Concepts |
The current Carnival of Space is up at OrbitalHub, with a lively take on habitable planets from Charles Magee’s Lounge of the Lab Lemming. Magee, now a field geologist in central Australia, once operated a laboratory that analyzed crystalline and glassy solids — ‘everything from dead people to bits of the Moon,’ as Charles puts it — but he brings his analytical skills to bear this week on a much more theoretical problem: How much is a planet worth?
Greg Laughlin (UC-Santa Cruz) has been kicking the question around on his systemic site, creating a prize for the first planet to reach a million dollars in value on his scale, with Earth setting the baseline at four quadrillion. Mars weighs in at a mere $13,988 on this scale, yet no known exoplanet even comes near that disappointing valuation. Magee has fun with Greg’s equations and goes to work on Venus, focusing on its albedo. Assuming a terrestrial albedo (0.36), he quickly arrives at a Venusian temperature not dissimilar from the current weather near his home in Canberra, some 20 degrees Celsius.
But there’s always a catch:
Of course, pedantic observers may note that Venus’s blackbody behavior is tempered by the fact that the planet has a thick atmosphere, which warms the surface via the greenhouse effect. We can calculate this second order effect by substituting Teff (the model blackbody temperature — 230-330K depending on albedo) with Treal, the actual surface temperature of 737K. This drops the valuation from 1440 trillion dollars to 2.9×10-89 dollars, or 2.9×10-87 cents. But in the current financial environment, a mere 104 order of magnitude decrease is not a problem. Rather, it is a badge of honor. Who wouldn’t want ten thousand googles in times like these? Were I an investment manager instead of a geologist, I could use that as a justification for a million dollar bonus paid by congressional bailout.
Ouch. Not that there are many better bets in the Solar System, with Titan weighing in at 9.5X10-44 dollars, or our own Moon at 7X10-25 dollars. Yet Greg has a defense of the Venusian breakdown:
Point taken! I’m not sure though, that a top-dollar Venus necessarily points to a flaw. The valuations are a quantitative measure of potential for a planet to be habitable, given only bulk physical properties currently measurable across light years of space. One is still faced with the quandry of whether to invest in to finding out whether a given planet measures up. If Venus were sheathed in water clouds rather than sulfur dioxide clouds, it would quite possibly achieve its potential as a quadrillion-dollar world.
Among exoplanets, the Laughlin payscale isn’t kind to Gliese 581c, the world that provoked a boomlet in habitable planet speculation not so long ago. Laughlin’s methods bring in a value of $158.32. ‘Potentially habitable’ is not the same thing as ‘habitable,’ of course, but it’s interesting to see that Laughlin believes the first planet to fit the former description will likely be discovered within the next year using radial velocity methods from the ground. His formula for calculating its value is worth reading about.
All this is great fun. Ponder this: The US paid $15 million for the Louisiana Territory in 1803. As Laughlin notes, it’s a figure that works out to $750 million in today’s currency. The cost of the Kepler mission is roughly $600 million, a comparable figure. While the Louisiana purchase brought in two million square miles (one percent of Earth’s square mile total surface), Kepler may discover on the order of 100 terrestrial planets. How much is such a world worth? Hint: A habitable Alpha Cen Bb (not in Kepler’s field of view) clocks in at $6.4 billion.
But back to the Carnival: Ethan Siegel looks at Govert Schilling’s The Hunt for Planet X: New Worlds and the Fate of Pluto (Springer, 2008), an account of the unfolding discovery of the outer Solar System (I’ve had this one on my desk for some time and keep intending to get to it, but it’s quickly making its way to the top of the stack — more later). Also, don’t miss Nancy Atkinson’s Universe Today interview with John Mather, discussing the James Webb Space Telescope, where it’s been and where it’s going. JWST’s infrared views of Kepler-identified terrestrial worlds should provide much useful information, hopefully enough to clarify the difference between blue chip performers and toxic assets.
by Paul Gilster | Mar 23, 2009 | Astrobiology and SETI |
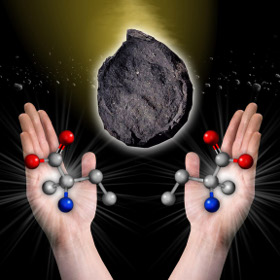
Twenty different amino acids go into making up the vast variety of proteins so essential to life. But why does life on Earth use only left-handed versions of amino acids to build them? After all, amino acids can be made in mirror images of each other. Jason Dworkin (NASA GSFC) notes the key issue. Mix left- and right-handed amino acids and “…life turns to something resembling scrambled eggs — it’s a mess. Since life doesn’t work with a mixture of left-handed and right-handed amino acids, the mystery is: how did life decide — what made life choose left-handed amino acids over right-handed ones?”
Image: This artist’s concept uses hands to illustrate the left and right-handed versions of the amino acid isovaline. Credit: NASA/Mary Pat Hrybyk-Keith.
It’s a question with ramifications for life elsewhere in the universe. Suppose the day comes when we finally get a robotic lander to Enceladus. The news flashes around the world: Life discovered on one of Saturn’s moons! But is it truly an extraterrestrial microbe, or the result of contamination from our own lander? Assuming the microbe is based on right-handed amino acids, its extraterrestrial credentials seem assured. But what if it’s left-handed? For that matter, what if left-handedness is fairly common?
We’re beginning to gain evidence for the possibility. In a study of meteorites with an abundance of carbon — carbonaceous chondrites — Dworkin and Daniel Glavin examined their samples for the amino acid isovaline. Chemical reactions in the presence of radiation or temperature can cause amino acids to switch from being left-handed to right and vice versa, but the beauty of using isovaline in these studies is that it preserves its handedness for billions of years. Moreover, there is no question of contamination, since isovaline is rarely used by life.
The result: Three types of carbonaceous meteorites showed more of the left-handed verson of isovaline than the right-handed kind. Is there a bias toward left-handedness in space? If so, that could make the search for extraterrestrial life a bit more tricky. Dworkin amplifies:
“If we find life anywhere else in our solar system, it will probably be microscopic, since microbes can survive in extreme environments. One of the biggest problems in determining if microscopic life is truly extra-terrestrial is making sure the sample wasn’t contaminated by microbes brought from Earth. If we find the life is based on right-handed amino acids, then we know for sure it isn’t from Earth. However, if the bias toward left-handed amino acids began in space, it likely extends across the solar system, so any life we may find on Mars, for example, will also be left-handed.”
So we may find that life based on left-handedness is the norm, a fact that could imply that the crucial choice of ‘handedness’ is readily solved. Dworkin goes on:
“On the other hand, if there is a mechanism to choose handedness before life emerges, it is one less problem prebiotic chemistry has to solve before making life. If it was solved for Earth, it probably has been solved for the other places in our solar system where the recipe for life might exist, such as beneath the surface of Mars, or in potential oceans under the icy crust of Europa and Enceladus, or on Titan.”
The paper is Glavin and Dworkin, “Enrichment of the amino acid l-isovaline by aqueous alteration on CI and CM meteorite parent bodies,” Proceedings of the National Academy of Sciences March 16, 2009 (early edition). Abstract online.
by Paul Gilster | Mar 21, 2009 | Exoplanetary Science |
Spotting transiting planets is what missions like CoRoT and Kepler are all about. The next step, getting a read on what’s in the atmosphere of any transiting, terrestrial world, is going to be tricky. The biomarkers like ozone and methane, so crucial for determining whether there’s life on a distant planet, are beyond the range of existing spacecraft. But the the next generation James Webb Space Telescope is also in the works, scheduled for launch in 2013.
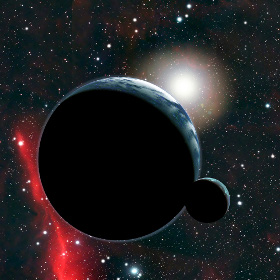
For nearby Earth-class worlds, JWST may be up to the task, at least for terrestrial planets that transit. In fact, if Alpha Centauri A turns out to have a transiting Earth-like planet (a major if!), it would take only a few transits to study the light filtering through its atmosphere to look for signs of life. Alpha Centauri is problematic in any case, but a recent study shows that the method — breaking down the star’s light during a transit to look for the characteristic markers — could be extended to other stars, provided enough transits can be measured.
Image: This artist’s conception shows a hypothetical twin Earth orbiting a Sun-like star. A new study shows that characterizing a distant Earth’s atmosphere will be difficult, even using next-generation technology like the James Webb Space Telescope. If an Earth-like world is nearby, though, then by adding observations of a number of transits, astronomers should be able to detect biomarkers like methane or ozone. Credit: David A. Aguilar (CfA).
Lisa Kaltenegger (Harvard-Smithsonian Center for Astrophysics) and Wesley Traub (Jet Propulsion Laboratory) have been studying JWST in this context. Says Kaltenegger:
“We’ll have to be really lucky to decipher an Earth-like planet’s atmosphere during a transit event so that we can tell it is Earth-like. We will need to add up many transits to do so – hundreds of them, even for stars as close as 20 light-years away.”
If we’re looking at a planet in an Earth-like orbit around a G-class star, then a ten-hour transit once a year is the best we can expect, meaning that collecting a hundred hours of transit data would take ten years. But the same world orbiting in the habitable zone of a red dwarf would make many more transits in the same amount of time because of its proximity to the primary star, which is why Kaltenegger says nearby M-dwarfs “…offer the best possibility of detecting biomarkers in a transiting Earth’s atmosphere.”
The paper is Kaltenegger and Traub, “Transits of Earth-like Planets,” accepted by The Astrophysical Journal and available online.
by Paul Gilster | Mar 19, 2009 | Missions |
by Kelvin Long
Project Daedalus was the first thoroughly detailed study of an interstellar vehicle, producing a report that has become legendary among interstellar researchers. But Daedalus wasn’t intended to be an end in itself. Tau Zero practitioner Kelvin Long here offers news of Project Icarus, a follow-up that will re-examine Daedalus in light of current technologies. A scientist in the plasma physics industry and an aerospace engineer, Long is assembling the team that will begin this work in 2010, following a ‘Daedalus After 30 Years’ symposium scheduled for September at the headquarters of the British Interplanetary Society. Can we improve Daedalus’ propulsion systems, change its targets, modify its shielding? Numerous theoretical studies await.
During the period 1973-1978 members of the British Interplanetary Society undertook a theoretical study of a flyby mission to Barnard’s star, some 5.9 light years away. This was Project Daedalus, which remains the most detailed study of an interstellar probe ever attempted. The 54,000 ton two-stage vehicle was to be powered by inertial confinement fusion using electron beams to compress deuterium/helium-3 fusion capsules to ignition.
Daedalus was to have obtained an eventual cruise velocity of 36,000 km/s or 12% of light speed from over 700 kN of thrust, burning at a specific impulse of 1 million seconds. Travel time to flyby at destination would be approximately 50 years.
Daedalus had three stated guidelines:
- The spacecraft must use current or near-future technology.
- The spacecraft must reach its destination within a human lifetime.
- The spacecraft must be designed to allow for a variety of target stars.
Announcing Project Icarus
Project Icarus: Son of Daedalus – flying closer to another star. This is the full name of the new study, a Tau Zero Foundation initiative in collaboration with the British Interplanetary Society (BIS). Over three decades have passed since the Daedalus work, making this a good time to revisit the design study in light of scientific and technological advancements.
The purpose of Project Icarus is as follows:
- To design a credible interstellar probe that is a concept design for a potential mission this century.
- To allow a direct technology comparison with Daedalus and provide an assessment of the maturity of fusion based space propulsion for future precursor missions.
- To generate greater interest in the real term prospects for interstellar precursor missions that are based on credible science.
- To motivate a new generation of scientists to be interested in designing space missions that go beyond our solar system.
Certain reference points follow on from the original Daedalus study, modified to reflect changes of time and circumstance. Thus the spacecraft design must use current or near-future technology so that it could be credibly launched by 2050. It must be designed to reach its destination as quickly as possible, in a time-frame not exceeding sixty years but, hopefully, much sooner. The propulsion system must be mainly fusion-based. Assuming realistic maximum cruise speeds of 0.3 c and a sixty year flight duration, this places approximately forty-eight stars within an 18 light year distance within range of Icarus.
Genesis of a New Starship Study
In the introduction to the Daedalus study report Alan Bond states that:
“…it is hoped that these ‘cunningly wrought’ designs of Daedalus will be tested by modern day equivalents of Icarus, who will hopefully survive to suggest better methods and techniques which will work where those of Daedalus may fail, and that the results of this study will bring the day when mankind will reach out to the stars a step nearer.”
So in essence, the naming of the successor project as Icarus was suggested by the original study group.
Daedalus and Icarus were characters from ancient Greek mythology. In an attempt to escape the labyrinthine prison of King Minos, Icarus’ father Daedalus fashioned a pair of wings made of feathers and wax for both himself and his son. But soaring joyfully through the sky, Icarus flew too close to the sun, melting the wax on his wings. He fell into the sea and died after having ‘touched’ the sky. Project Icarus aims to ‘touch’ the stars and escape from the bounds of mother Earth.
Toward an Evolving Design
An assessment of the many dozens of propulsion concepts for interstellar flight made it clear that one way to advance the prospects for interstellar travel would be to focus on a specific design proposal. This way, a concept design could be derived, iterated and improved. Over time, the concept would be worked upon by future generations and could ultimately lead to a design blueprint for an interstellar probe. As the Daedalus study was performed three decades ago, it seemed appropriate to start by re-designing the Daedalus probe with updated scientific knowledge.
Thus the genesis of Project Icarus. It is hoped that other teams around the world will eventually be assembled, working on specific propulsion proposals that have been investigated in the past, such as Starwisp, VISTA, AIMStar or one of the many others. In this way, the technological maturity of different propulsion schemes can be improved over time, drawing on a common background of study rather than diverse and uncoordinated research efforts.
Needless to say, Centauri Dreams will provide regular updates on the progress of the Icarus design team, which will also be developing its own Web site in conjunction with the September symposium.