Centauri Dreams
Imagining and Planning Interstellar Exploration
Radioactive Elements and Planetary Habitability
A planetary dynamo may be a key factor in creating the conditions needed for life. And creating that dynamo seems to depend on the radioactive decay of thorium and uranium, generating internal heating and driving plate tectonics. Let’s carry this line of thought further, though, as the authors of a new paper out of UC-Santa Cruz do, and point out that these heavy elements are necessary to create a magnetic field like Earth’s, which protects us from damaging radiation.
In the rocky planets, magnetic fields are generated by convection in a metallic core, which in turn is driven by heat extracted into the mantle (Nimmo 2015; Labrosse 2015; Boujibar et al. 2020). Since mantle radiogenic heat production controls how much heat is extracted from the core, it will also influence the presence or absence of a dynamo. Similarly, heat production will control the mantle temperature and thus the rate of silicate melting and volcanism.
That quote is from the paper, whose lead author is Francis Nimmo, and it points to the key role of radiogenic heat in the mantle. Within the Earth, there are enough radioactive elements to generate a persistent ‘geodynamo,’ in which molten iron and nickel in motion in the planet’s outer core produce electric currents, forming a magnetic field as the Earth rotates. The authors find that Earth “had just enough radiogenic heating to maintain a persistent dynamo.” And that has implications for the atmosphere.
UC-Santa Cruz’ Natalie Batalha is director of the university’s Astrobiology Initiative, whose multidisciplinary work is designed to produce the kind of widespread collaboration found in this paper. Says Batalha:
“It has long been speculated that internal heating drives plate tectonics, which creates carbon cycling and geological activity like volcanism, which produces an atmosphere. And the ability to retain an atmosphere is related to the magnetic field, which is also driven by internal heating.”
To ponder how all these effects come together, we have only to look at Mars, where the lack of a magnetic field can be tied to the gradual loss of an atmosphere that was once thick enough to support water on the surface and, potentially, a living environment. The planet’s low gravity was one factor in the gradual thinning of the atmosphere, but the solar wind of charged particles from the Sun is likewise a problem, for without a protective magnetic field, the atmosphere erodes.
We have an angle here into habitability on planets around other stars, for the amount of radioactive material is going to vary from one stellar system to another. The so-called r-process elements — created by nuclear reactions that are responsible for producing roughly half of the atomic nuclei heavier than iron — owe their origin to supernova events and the mergers of neutron star remnants, events that will be stochastic and result in planets with varying degrees of radiogenic materials. Back to the paper on this point:
From the point of view of long-term radiogenic heating, the most important elements are potassium, thorium, and uranium. Th and U are both r-process elements, most likely produced in neutron star mergers (NSMs) (Kasen et al. 2017; Cowan et al. 2019), with possibly significant contributions also from massive star collapse explosions (Siegel et al. 2019; Macias & Ramirez-Ruiz 2019). Because such events are rare (an occurrence rate of tens/Myr in our Galaxy) and produce large quantities of r-process elements, the resulting concentration of r-process elements should vary considerably. This is especially true of low-metallicity stars, because turbulent mixing of the interstellar medium is inefficient in the early Galaxy (Shen et al. 2015; Naiman et al. 2018). This expectation is in agreement with measurements of r-process europium in low-metallicity stars (e.g., Macias & Ramirez-Ruiz 2018) and with r-process abundances in dwarf galaxies (e.g., Ji et al. 2016).
Lead author Francis Nimmo realized that if planets vary in the amount of radioactive elements, we should then expect geological activity and magnetic fields to vary as well. The UC-Santa Cruz scientists essentially adjusted radiogenic heat production in their models to see how this factor affected planetary habitability. As Nimmo puts it:
“We took a model of the Earth and dialed the amount of internal radiogenic heat production up and down to see what happens. Just by changing this one variable, you sweep through these different scenarios, from geologically dead to Earth-like to extremely volcanic without a dynamo.”
When radiogenic heating is dialed up higher than the Earth’s, no dynamo is produced. With most of the thorium and uranium in the mantle, the excessive heat becomes an insulator, so that the loss of heat from the molten core is slowed. The result: Heat loss from the core cannot generate convection strong enough to produce a magnetic field, with all that implies about the survival of an atmosphere and radiation flux on the surface.
Given all that, the associated volcanic activity produced by greater levels of radiogenic internal heating is almost lost in the shuffle, but it could obviously be a factor in mass extinction events. And while that implies that high levels of radiogenic heating are a key factor in suppressing a habitable environment, the study also shows that too little heat results in no volcanism whatsoever. We wind up with a planet that, like Mars, appears geologically dead.
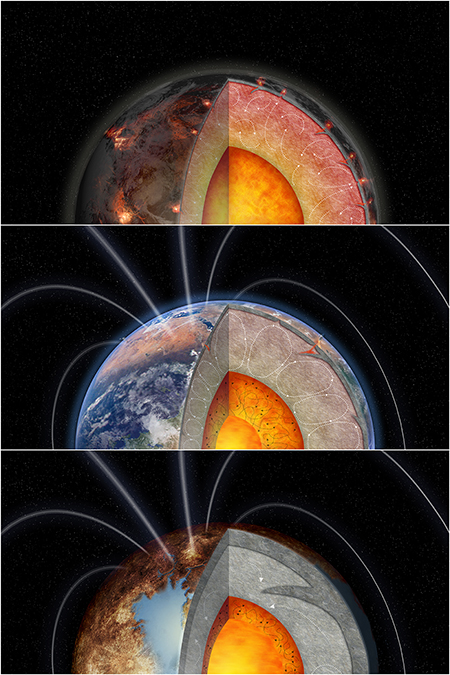
A new study identifies internal heating from radioactive decay as a critical factor in a planet’s ability to generate a magnetic field and retain an atmosphere. Credit: Illustration by Melissa Weiss for UC Santa Cruz
Image: These illustrations show three versions of a rocky planet with different amounts of internal heating from radioactive elements. The middle planet is Earth-like, with plate tectonics and an internal dynamo generating a magnetic field. The top planet, with more radiogenic heating, has extreme volcanism but no dynamo or magnetic field. The bottom planet, with less radiogenic heating, is geologically “dead,” with no volcanism. Caption credit: UC-Santa Cruz.
So we are working with a single variable, one that can be studied via spectroscopy to measure the abundance of the various elements in stars, the assumption being that planets will have the same composition as the stars they orbit. Here the rare earth element europium comes into play, readily observed in stellar spectra, and created by the same processes that produce thorium and uranium. In this work, europium is the tracer for studying radiogenic abundance.
We already have europium measurements for many nearby stars, which allowed Nimmo and team to set a range of inputs for their models of radiogenic heating, with the Sun’s composition establishing the middle of the range. Europium thus becomes a measurement that can flag radiogenic elements in exoplanetary systems as we look forward to the deployment of the James Webb Space Telescope. The europium measurement may well show us which stellar systems are likely to produce planets with the internal composition life would need to survive. From the paper:
…higher mantle heating rates increase volcanic activity and shut off the dynamo, both likely deleterious for habitability. In contrast, reduced mantle heating rates will at some point stop melt production. A potential consequence is that plate tectonics will then cease (Louren¸co et al. 2018), and with it, the dynamo, as at Venus (Nimmo 2002). It is tempting to speculate that the Earth is habitable in part because it possesses a “Goldilocks” concentration of radiogenic elements: high enough to permit long-lived dynamo activity and plate tectonics, but not so much that extreme volcanism and dynamo shutoff occur. As yet, however, our understanding of the complicated feedbacks involved is too rudimentary to make such a definitive conclusion; more detailed calculations, and better characterization of the radiogenic element abundances in planet-hosting stars, are both required.
The paper is Nimmo et al., “Radiogenic Heating and Its Influence on Rocky Planet Dynamos and Habitability,” Astrophysical Journal Letters Vol. 903, No. 2 (10 November 2020). Abstract / Preprint.
An Unusual (and Promising) Brown Dwarf Detection
The naming of names is quite a project when it comes to new astronomical objects, and given the sheer numbers — 300 million habitable planets around G- and K-class stars, for example — we might do better to stick with simple identifiers. On the other hand, it’s a bit charming that a new brown dwarf known by its identifier as BDR J1750+3809 has been dubbed ‘Elegast’ by the discovery team. This is the first substellar object found through radio observations.
The name is both appropriate and specific to the discovery space. Elegast appears in a poem in Middle Dutch (12th or 13th Century) called ‘Karel ende Elegast,’ with the character Elegast being a vassal of Charlemagne who seems to be king of the elves (Wikipedia to the rescue, vindicating once again my decision to send them a monthly donation).
The Dutch connection is that the radio work comes out of LOFAR (Low-Frequency Array), which is currently the largest radio telescope operating at the lowest frequencies that we can observe from Earth. Based in the Netherlands, LOFAR is an international effort with field stations in eight countries.
Elegast breaks from the norm in not being discovered from the kind of infrared sky surveys through which most other brown dwarfs have been found. You might recall our discussions of the WISE mission and the possibility, not realized, of finding a brown dwarf closer than the Alpha Centauri stars. But it’s also true that infrared surveys can’t detect objects that are sufficiently cold and faint, whereas we now have radio wavelengths to fill the gap.
Is there still some faint possibility of a closer brown dwarf? T-class dwarfs are defined as being less than 1500 Kelvin, but WISE identified Y-dwarfs with temperatures as cool as 25 °C (298 K), which gets us down to where my thermostat is set as I write this. WISE 1828+2650, I subsequently learned, is so cold that it emits no visible light at all and is more or less indistinguishable from a free-floating planet. Get any colder and infrared surveys won’t find such worlds, which is why the news about Elegast is so encouraging.
Given that the population of so-called ‘rogue’ planets may be vast, it’s wonderful to see a new method becoming available with which to study nearby examples. Brown dwarfs may not be able to create the kind of fusion reactions found in stars like the Sun, but they emit at radio wavelengths just as gas giant planets do. Jupiter’s emissions are well studied, the result of powerful magnetic fields around the planet accelerating charged particles to produce radio waves as well as aurorae. Brown dwarfs are faint at these wavelengths but detectable.
Image: Artist’s impression of Elegast. The blue loops depict the magnetic field lines. Charged particles moving along these lines emit radio waves that LOFAR detected. Some particles eventually reach the poles and generate aurorae similar to the northern lights on Earth. Credit: ASTRON / Danielle Futselaar.
The LOFAR observations had to contend with background galaxies when investigating potential brown dwarfs. The key turned out to be identifying signals that were circularly polarized, which distinguishes planets and brown dwarfs from the background ‘noise.’ What this kind of polarization means is that the electromagnetic field of the radio waves rotates in a characteristic circular pattern. Says LOFAR project scientist Tim Shimwell: “We could not have picked out Elegast in our standard radio images from among the crowd of millions of galaxies, but Elegast immediately stood out when we made circularly polarised images.”
In the case of Elegast, the Gemini-North Telescope had produced archival imagery used to confirm the discovery, and the NASA Infrared Telescope Facility (IRTF) deployed an infrared spectrometer known as SpeX, recently upgraded, to obtain a spectrum of Elegast. The signature of methane, common in brown dwarfs and gas giant planets, was revealed.
Beyond its possibilities at identifying the faintest brown dwarfs and free-floating planets, radio astronomy may also be used to study exoplanet magnetic fields, testing theories on magnetic field strength on objects that have enough similarities to gas giants to make the investigation worthwhile. The team is now making follow-up observations on Elegast to measure its magnetic field even as they use LOFAR to look for more such objects. Harish Vedantham, an astronomer at the Netherlands Institute for Radio Astronomy (ASTRON) is lead author of the study:
“Our ultimate goal is to understand magnetism in exoplanets and how it impacts their ability to host life. Because magnetic phenomena of cold brown dwarfs like Elegast are so similar to what is seen on solar system planets, we expect our work to provide a vital datapoint to test theoretical models that predict the magnetic fields of extrasolar bodies.”
The paper is Vedantham et al., “Direct radio discovery of a cold brown dwarf,” Astrophysical Journal Letters Vol. 903, No. 2 (9 November 2020). Abstract / Preprint.
Europa: Night-time Glow a New Tool for Analysis
When it comes to Europa, it’s the surface that counts as we try to learn more about the ocean beneath. Maybe one day we’ll be able to get some kind of probe through the ice, but for now we have to think about things like upwellings of water that percolate up through cracks in the frozen landscape, and unusual areas like Europa’s ‘chaos’ terrain. Here, fractures and evident ‘rafts’ of ice show disruptions where the icy surface of the moon experiences Jupiter’s tidal effects.
Image: The surface of Jupiter’s moon Europa features a widely varied landscape, including ridges, bands, small rounded domes and disrupted spaces that geologists called “chaos terrain.” This newly reprocessed image, along with two others along the same longitude, was taken by NASA’s Galileo spacecraft on Sept. 26, 1998, and reveals details of diverse surface features on Europa. Credit: NASA/JPL-Caltech/SETI Institute.
What kind of materials might we find frozen into the cracks and grooves of such terrain? Europa Clipper will doubtless tell us more as it updates Galileo’s two-decade old data. Meanwhile, we recently learned about another way to study the moon’s surface composition. At the Jet Propulsion Laboratory, Murthy Gudipati and team have been doing laboratory work that informs our understanding of how Europa reacts to the inflow of high-energy radiation from Jupiter. The high flux of charged particles occurs because of interactions with Jupiter’s magnetic field.
The key to the work is the fact that different salty compounds produce their own unique signature as they respond to this radiation bath. A glow emerges — sometimes green to the eye, sometimes edging into blue or white — depending on the materials in question. Gudipati’s team used a spectrometer, as we would expect, to study the surface, but here the observations are taking place not with reflected sunlight on the dayside but Europa’s own glow at night. The paper on this work, which appeared in Nature Astronomy, shows us how much data could be gathered by a method that seems to have surprised the scientists involved.
JPL co-author Bryana Henderson explains:
“[W]e never imagined that we would see what we ended up seeing. When we tried new ice compositions, the glow looked different. And we all just stared at it for a while and then said, ‘This is new, right? This is definitely a different glow?’ So we pointed a spectrometer at it, and each type of ice had a different spectrum.”
Image: This illustration of Jupiter’s moon Europa shows how the icy surface may glow on its nightside, the side facing away from the Sun. New lab experiments re-created the environment of Europa and find that the icy moon shines, even on its nightside, due to an ice glow. As Jupiter bombards Europa with radiation, the electrons penetrate the surface, energizing the molecules underneath. When those molecules relax, they release energy as visible light. Variations in the glow and the color of the glow itself could reveal information about the composition of ice on Europa’s surface. Different salty compounds react differently to the radiation and emit their own unique glimmer. Color will vary based on the real composition of Europa’s surface. Credit: NASA/JPL-Caltech.
The process seems straightforward, but sometimes it takes replicating known conditions as closely as possible in a lab to discover the consequences. Start with ice mixed with salts like magnesium sulfate and sodium chloride. Bathe the mix in radiation. The glow is a natural result, its variations linked to different compositions of the ice. Those variations are what gives this work implications for missions like Europa Clipper.
Sodium chloride brine, for example, turned out to produce a lower level of glow in the team’s setup, which used an instrument built at JPL known delightfully as ICE-HEART (Ice Chamber for Europa’s High-Energy Electron and Radiation Environment Testing). The experiments were run at a high-energy electron beam facility in Gaithersburg, Maryland once ICE-HEART had been taken there, the original plan being to study organic material under the Europan ice. The continuous glow on Europa’s night side now emerges as a source of future data, one that can be compared with these laboratory results to identify salty components on the surface.
“It’s not often that you’re in a lab and say, ‘We might find this when we get there,'” Gudipati said. “Usually it’s the other way around – you go there and find something and try to explain it in the lab. But our prediction goes back to a simple observation, and that’s what science is about.”
So Europa Clipper can use night-time flybys to delve into the chemical composition below, a method that may turn out to be helpful on other Galilean moons, or wherever high doses of ionizing radiation sleet down upon a frozen surface. Specific to Europa Clipper’s instruments, we learn the following:
Observation of night-time, high-energy, radiation-induced ice glow on the trailing hemisphere could thus provide more constraints on the chemical composition of non-ice material, revealing whether sulfates or chlorides are present, what their counterions could be, and whether pure water-ice patches are detectable. Dark regions could imply sodium- and chloride-dominant surfaces, whereas brighter regions could imply magnesium- and sulfate-dominant surfaces in the absence of water ice. The presence or absence of water ice could unambiguously be determined by the MISE [Mapping Imaging Spectrometer for Europa] instrument, designed to span a wavelength range of 0.8 μm to 5 μm, covering ice absorption features in the daytime.
In addition, the glow of night-time ice can be measured against daylight observations from Europa Clipper’s Wide Angle Camera and the MISE spectrometer to dig deeper into the chemical composition below. Some ultraviolet features of the night-time spectra will allow mission scientists to flag temperature anomalies on the surface for future observations.
The paper is Gudipati et al., “Laboratory predictions for the night-side surface ice glow of Europa,” Nature Astronomy 9 November 2020 (abstract).
On 300 Million Habitable Zone Planets
We’ve talked about the Drake Equation a good deal over the years, but I may not have mentioned before that when Frank Drake introduced it in 1961, it was for the purpose of stimulating discussion at a meeting at the National Radio Astronomy Observatory in Green Bank, West Virginia that was convening to discuss the nascent field of SETI.
This was in the era of Drake’s Project Ozma and the terms of the SETI debate were hardly codified. Moreover, as Nadia Drake recounts in this absorbing look back at her father’s work in that era, Drake had spent the time immediately before the meeting trying to line up Champagne for UC-Berkeley biochemist Melvin Calvin, who was about to win the Nobel Prize.
So there was a certain ad hoc flavor to the equation, one that Drake assembled more or less on the fly to clarify the factors to be considered in looking for other civilizations. How Drake did all this while trying to locate a sufficient quantity of good Champagne in the rural West Virginia of 1961 is beyond me and adds to his mystique.
Image: Astronomer Frank Drake speaking at Cornell University in Schwartz Auditorium, 19 October 2017. Credit: Wikimedia Commons CC BY-SA 4.0.
Sparkling wine aside, the Drake Equation in various forms has continued to inform discussion. The likelihood of detecting alien civilizations could be approached by multiplying the seven factors Drake came up with, which are shown in the figure below. The number of detectable alien civilizations is N. The Drake Equation obviously relied on guesswork at the time, given that we knew little about the factors involved other than the rate of star formation.
Image: The Drake Equation. Credit: Ming Hsu (UC-Berkeley).
There’s still a lot of play in these numbers, of course, but it’s fascinating to watch the progress of exoplanetary science as we begin to fill in the numbers through actual observation. Notice in particular ne the number of planets, per star, that could support life. This value is what gets play in the recently released (on arXiv) paper from Steve Bryson and a large number of colleagues at the SETI Institute, NASA and a variety of other organizations.
What catches the eye is the figure of 300 million, which is the number the researchers give for potentially habitable planets in the Milky Way. Let’s drill into this a bit: The researchers are computing the occurrence of rocky worlds, defined here as planets within a certain range of radius (0.5 R? ? r ? 1.5 R?), orbiting stars with effective temperatures of 4,800-6,300 K. The host stars cover main-sequence dwarf stars from Kepler’s DR25 planet candidate catalog as well as stars in data compiled by the European Space Agency’s Gaia mission. As the authors note: “We base our occurrence rates on differential population models dependent on radius, instellation flux and host star effective temperature.”
This is a change of pace from the norm, so let’s turn to the paper:
Most of the existing literature on habitable zone occurrence rates are in terms of orbital period, where a single period range is adopted to represent the bounds of the habitable zone for the entire stellar population considered. However, no single period range covers the habitable zone for a wide variety of stars…While these period ranges cover much of the habitable zone for G stars, they miss significant portions of the habitable zones of K and F stars, and include regions outside the habitable zone even when restricted to G stars. This will be true for any fixed choice of orbital period range for the range of stellar effective temperatures required for good statistical analysis. Such coverage will not lead to accurate occurrence rates of planets in the habitable zone.
Hence the decision to work with instellation flux, which measures the photon flux on each planet as received from its host star. The authors say that this is the first paper on occurrence rates for habitable zone planets that operates on star-dependent photon output. In terms of effective temperature, G-class stars like the Sun are in the range of 5,200–6,000 K. F-class is 6,000–7,500 K, but as the paper notes, the paucity of F stars in the sampled data leads to the authors setting the temperature limits lower. K-class stars show up at effective temperatures of 3,700–5,200 K. The range used in this paper — 4,800-6,300 K — also excludes M-dwarfs, whose effective temperatures range from 2,400–3,700 K.
Leaving out M-dwarfs could substantially under-count habitable zone worlds, but we also have enough concerns about tidal lock, stellar flare activity and atmospheric loss that we can’t assume M-dwarf planets are habitable. In any case, the authors have other reasons for the decision, including a very practical matter of future observation. After all, an analysis like this may well be useful as we ponder our target lists, and we also have to remember the limits of transit observation Kepler had to deal with:
The reason for limiting to Teff > 4800 K is two fold: (1) The inner working angle (IWA, the smallest angle on the sky at which a direct imaging telescope can reach its designed ratio of planet to star flux) for the LUVOIR coronagraph instrument ECLIPS falls off below 48 milliarc sec at 1 micron (3?/D) for a planet at 10 pc for Teff ? 4800 K, and (2) Planets are likely tidal-locked or synchronously rotating below 4800 K that could potentially alter the inner HZ limit significantly…The upper limit of 6300 K is a result of planets in the HZs having longer orbital periods around early F-stars, where Kepler is not capable of detecting these planets…
So bear this in mind: Excluding what could be vast numbers of habitable planets in M-dwarf orbits, we still wind up with 300 million possibilities in the broad range of K-class through G-class stars. Co-author Jeff Coughlin is director of Kepler’s Science Office:
“This is the first time that all of the pieces have been put together to provide a reliable measurement of the number of potentially habitable planets in the galaxy. This is a key term of the Drake Equation, used to estimate the number of communicable civilizations — we’re one step closer on the long road to finding out if we’re alone in the cosmos.”
Image: This illustration depicts Kepler-186f, the first validated Earth-size planet to orbit a distant star in the habitable zone. Credit: NASA Ames/JPL-Caltech/T. Pyle.
When you go through this paper, bear in mind what Centauri Dreams associate editor Alex Tolley pointed out to me — The Drake ne factor refers to the number of planets per star that can support life. What the Bryson et al. paper takes as its starting point is the number of rocky planets in the habitable zone, and this could mean that the figure of 300 million ‘habitable’ worlds takes in planets that resemble Venus more than Earth. It may also include water worlds, where the likelihood of technological civilization is unknown.
So Drake’s term ne is not the same value as taken up in the new paper. Nonetheless, let’s return to that dazzling figure of 300 million, because when we’re dealing with that many planets of interest, we can afford to lose a number that turn out to be uninhabitable and still consider ourselves overwhelmed with possibilities for life.
Numbers like these have implications for stars relatively near the Sun. The authors look at both the conservative and optimistic habitable zone, with the narrower ‘conservative habitable zone’ bounded by the ‘moist greenhouse’ and ‘maximum greenhouse’ limits, and the wider ‘optimistic habitable zone’ bounded by the ‘current Venus’ and ‘early Mars’ limits. I’m drawing this descrtiption from the Planetary Habitability Laboratory’s summary of work by Ravi kumar Kopparapu and colleagues (citation below).
Image: Habitable Zone of around main sequence FGKM stars. The warm ‘habitable’ zone is divided into a ‘conservative habitable zone’ (light green) and an ‘optimistic habitable zone’ (dark green). Earth is at the inner edge of the ‘conservative habitable zone.’ Credit: PHL.
Filtering their results using calculations for the conservative habitable zone, the authors maintain they can say with 95 percent confidence that the nearest rocky habitable zone planet around either a G- or K-class star is within 6 parsecs (roughly 20 light years). There could be four habitable zone rocky planets around G- and K-dwarfs within 10 parsecs of the Sun.
How to build our small planet catalog to reduce uncertainties in the calculations? The answer is clearly more space-based observations even as new ground-based telescopes come online. Let’s also remember what we lost because of Kepler’s mechanical problems. While we did get a K2 extended mission, the original Kepler extended mission was meant to continue the ‘long stare’ at the original starfield, adding four more years of precision photometric data. The number of small planets in the habitable zone would have been significantly extended.
…by definition, Kepler planet candidates must have at least three observed transits. The longest orbital period with three transits that can be observed in the four years of Kepler data is 710 days (assuming fortuitous timing in when the transits occur). Given that the habitable zone of many F and late G stars require orbital periods longer than 710 days, Kepler is not capable of detecting all habitable-zone planets around these stars.
Given that upcoming missions like PLATO do not include such long stares on a single field of stars (PLATO plans no more than 3 years of continuous observation of a single field), we will need future missions to achieve what the original Kepler extended mission might have done, which would have been a doubling of the DR25 dataset and a large yield of small habitable zone planets.
The paper is Bryson et al., “The Occurrence of Rocky Habitable Zone Planets Around Solar-Like Stars from Kepler Data,” in process at the Astronomical Journal (abstract). The Kopparapu et al. paper is “Habitable Zones Around Main-Sequence Stars: New Estimates,” Astrophysical Journal Vol. 765, No. 2 (26 February 2013). Abstract.
A Fast Radio Burst in the Milky Way
A sequence of new observations gives us a leading candidate to explain Fast Radio Bursts (FRBs). These powerful bursts of radio waves, lasting but milliseconds, first turned up in our data in 2007 and have been a mystery ever since. As they were found in other galaxies, it has been difficult to determine their exact location, and they were impossible to predict as most seemed to be one-off events, although astronomers have subsequently found some that do repeat.
Among the possible causes of FRBs, stellar remnants have been put forward, with the kind of highly magnetic neutron stars called magnetars receiving close scrutiny because their magnetic fields could be the engine driving the bursts. We now have three papers in Nature that give us tight observational evidence of the kind that has been lacking. Between the three, we have data that for the first time link an FRB in our own galaxy to a magnetar, the object known as SGR 1935+2154, located in the constellation Vulpecula.
Image: Artist’s impression of a magnetar in outburst, showing complex magnetic field structure and beamed emission, here imagined as following a crust cracking episode. Credit: McGill University Graphic Design Team.
Let’s look at the events involved here. We begin with two space-based observatories. Both the Fermi Gamma-ray Space Telescope and the Neil Gehrels Swift Observatory registered rapid bursts of gamma- and X-rays from this object in late April. The spate of burst activity lasted for hours and was also observed by NASA’s Neutron star Interior Composition Explorer, an X-ray telescope mounted on the International Space Station.
This was followed by observations about thirteen hours later of another X-ray burst, once again seen by numerous instruments, including the European Space Agency’s INTEGRAL mission as well as China’s Huiyan X-ray satellite and the Russian Konus gamma-ray burst monitor, a Russian experiment that flies aboard NASA’s GGS-Wind spacecraft. This was a burst lasting about half a second, but as it flared, the Canadian Hydrogen Intensity Mapping Experiment (CHIME) and the Survey for Transient Astronomical Radio Emission 2 (STARE2), located at CalTech, detected a Fast Radio Burst (FRB 200428) in the same part of the sky.
Scientists at the Chinese Five-hundred-meter Aperture Spherical radio Telescope (FAST) had been observing SGR 1935+2154 for some weeks. As I understand it, they did not see FRB 200428, but were subsequently able to detect an FRB at the same location (FAST was not observing the object when FRB 200428 was detected by the other instruments).
Citations for papers from both the CHIME/FRB Collaboration and the STARE2 team are given below, along with a citation for a paper from FAST astronomers that provides limits to the radio flux of the FRB. The authors of the latter find a ‘weak correlation’ between FRBs and SGR 1935+2154, as co-author Zhang Bing (University of Nevada) explains:
“The weak correlation could be explained by special geometry and/or limited bandwidth of FRBs. The observations of SGR J1935 start to reveal the magnetar origin of FRBs, although other possibilities still exist.”
So we have a burst of X-rays associated with an FRB, the latter radio component detected first at CHIME and then STARE2. Moreover, the radio burst from SGR 1935+2154 was thousands of times brighter than any radio emissions previously observed from magnetars in the Milky Way, and in fact would have registered as a weak FRB had it occurred in another galaxy. The arrival of the radio pulse during an X-ray burst points to this magnetar as the source.
Paul Scholz is a researcher at the University of Toronto’s Dunlap Institute for Astronomy & Astrophysics and a member of the CHIME/FRB Collaboration:
“The radio burst was far brighter than anything we had seen before, so we immediately knew it was an exciting event. We’ve studied magnetars in our galaxy for decades, while FRBs are an extragalactic phenomenon whose origins have been a mystery. This event shows that the two phenomena are likely connected.”
Image: A powerful X-ray burst erupts from a magnetar – a super-magnetized version of a stellar remnant known as a neutron star – in this illustration. A radio burst detected April 28 occurred during a flare-up like this on a magnetar called SGR 1935. Credit: NASA’s Goddard Space Flight Center/Chris Smith (USRA).
The magnetic field of a magnetar can be a thousand times stronger than that of a typical neutron star, offering us a generous source of power, but at the same time, the radio burst from SGR 1935+2154 was thousands of times brighter than any radio emissions ever detected from magnetars in our galaxy. Paul Scholz has speculated that it may require the youngest, most active magnetars to explain the range of FRB sources we’ve thus far observed elsewhere, although we have yet to make a simultaneous detection tying an FRB with an X-ray burst in another galaxy, a signature that efforts like CHIME will continue to look for.
George Younes (George Washington University) is lead author on two upcoming papers on the FRB. Here he speculates on the nature of the burst activity:
“The bursts seen by NICER and Fermi during the storm are clearly different in their spectral characteristics from the one associated with the radio blast. We attribute this difference to the location of the X-ray flare on the star’s surface, with the FRB-associated burst likely occurring at or close to the magnetic pole. This may be key to understanding the origin of the exceptional radio signal.”
Image: This aerial view shows the Canadian Hydrogen Intensity Mapping Experiment (CHIME), a radio telescope located at Dominion Radio Astrophysical Observatory in British Columbia. Credit: Richard Shaw/UBC/CHIME Collaboration.
So we have the first FRB found in the Milky Way, one whose association with a magnetar may help us place these bursts in context now that we know magnetars can drive FRB activity. On exactly how a magnetar produces an FRB, I want to quote Amanda Weltman and Anthony Walters, who provide a fine overview of this work in an article in Nature that is not behind the journal’s firewall:
…there are several mechanisms by which magnetars can drive FRBs, each of which has a distinct observational signature. The new results thus open up a host of exciting problems to explore. For example, what theoretical mechanism could give rise to such bright, yet rare, radio bursts with X-ray counterparts? One promising possibility is that a flare from a magnetar collides with the surrounding medium and thereby generates a shock wave. Observations of nearby rapidly star-forming galaxies will be crucial for finding events similar to FRB 200428, to help pin down the actual mechanism.
The paper from the STARE team is Bochenek et al., “A fast radio burst associated with a Galactic magnetar,” Nature 587 (2020), pp. 59-62 (abstract). The CHIME/FRB paper is “A bright millisecond-duration radio burst from a Galactic magnetar,” Nature 587 (2020), pp. 54-58 (abstract). The Lin paper is “No pulsed radio emission during a bursting phase of a Galactic magnetar,” Nature 587 (2020), pp. 63-65 (abstract).
Voyager 2: Back in Two-Way Communication
It’s reassuring to hear that we’re in two-way contact once again with Voyager 2. Since last March, controllers have been limited to receiving X-band (8 to 12 GHz) downlink data, with no capability to uplink commands to the craft via S-band (2 to 4 GHz). This has been a problem unique to Voyager 2 thanks to its trajectory. The Deep Space Network’s three radio antenna facilities — Canberra, Australia; Goldstone, California and Madrid, Spain — are positioned so that at least one facility is available for communications with our far-flung space probes.
While Voyager 1 can talk to us via the two northern hemisphere DSN stations, Voyager 2’s close flyby of Neptune’s large moon Triton in 1989 bent its course well south of the ecliptic. 18.8 billion kilometers from Earth, Voyager 2 can only line up on Canberra, and the antenna called Deep Space Station 43 (DSS43) has been the only southern hemisphere dish with a transmitter capable of reaching the craft at the right frequency to send commands. DSS43 went offline for equipment upgrades to handle increasingly problematic aging equipment.
Philip Baldwin is operations manager for NASA’s Space Communications and Navigation (SCaN) Program;
“The DSS43 antenna is a highly specialized system; there are only two other similar antennas in the world, so having the antenna down…is not an ideal situation for Voyager or for many other NASA missions. The agency made the decision to conduct these upgrades to ensure that the antenna can continue to be used for current and future missions. For an antenna that is almost 50 years old, it’s better to be proactive than reactive with critical maintenance.”
Image: Crews conduct critical upgrades and repairs to the 70-meter-wide radio antenna Deep Space Station 43 in Canberra, Australia. In this image, one of the antenna’s white feed cones (which house portions of the antenna receivers) is being moved by a crane. Credit: CSIRO.
Only DSS43 has the S-band transmitter powerful enough to reach and communicate with Voyager’s dated technology, but the upgrades will also be significant for Moon and Mars missions going forward. From the Voyager 2 perspective, the craft has been in a quiescent mode that still allowed return of science data, with Canberra’s three 34-meter dishes configured to listen to its signal, though unable to transmit commands. Now DSS43 has successfully tested its new hardware and we can get back to uploading commands as needed to the probe.
“What makes this task unique is that we’re doing work at all levels of the antenna, from the pedestal at ground level all the way up to the feedcones at the center of the dish that extend above the rim,” said Brad Arnold, the DSN project manager at NASA’s Jet Propulsion Lab in southern California. “This test communication with Voyager 2 definitely tells us that things are on track with the work we’re doing.”
All of which is excellent news, as Voyager 2 seems healthy. Recall that the spacecraft accidentally overdrew its power supply last January, leading to the automatic shutdown of its science instruments. The upgrade of DSS43 began after that problem had been resolved. The station now has two new radio transmitters as well as upgraded heating and cooling equipment, power supply and support upgrades demanded by the new transmitters.
So both our active craft in interstellar space are in two-way communication again. It’s instructive, and a bit awe-inspiring, to remember how difficult it is to track a signal as weak as the one Voyager can produce. At Jupiter, the craft could transmit at 115,000 kilobits per second. This is a 23-watt radio transmitter that produced, at the giant planet, a signal that was one hundred-millionth as powerful as a cell phone battery by the time it reached the DSN on Earth. And of course Voyager keeps going. Even before it went interstellar, Voyager 2’s power levels received at Earth were more than five hundred times fainter than at the Jupiter encounter.
We may be able to keep the Voyagers alive into the middle of this decade by clever cycling of their instruments and systems, with the last surviving science instrument likely being the magnetometer, which has the lowest power requirement. A faint engineering signal might still be feasible into the 2030s, but no one knows exactly. It would be wonderful if we could stay in touch with these craft until 2027, which would mark their 50th year in flight. Go Voyager.