by Paul Gilster | Nov 24, 2006 | Culture and Society, Missions |
When do you decide to launch a starship? It’s a question based as much on cultural assumptions as technology. Start with the premise that we can ratchet up today’s velocities to 150 kilometers per second, roughly ten times the speed at which New Horizons will cross Pluto’s orbit. If we want to send a probe six light years to Barnard’s Star at that speed, we would be looking at a travel time of 12,000 years. That’s a lot of time, but better than Voyager’s 70,000-year plus travel time to the Centauri stars (if either Voyager were pointed in their direction).
Clearly, 12,000 years is too many, especially in an age that regards maximum mission time as the lifetime of a researcher working on the project. Besides, if we did launch that kind of mission, it would inevitably be passed enroute by a faster spacecraft. And that’s the conundrum: does there ever come a time when you do launch, or are you always waiting for better propulsion systems and faster travel times?
As Andrew Kennedy discovers in a paper we have just been discussing, there is indeed an optimum time, though one with a twist. Kennedy works with a doubling equation to describe how growth affects velocity of travel. From the paper:
If technology growth is likely to double every 100 years the speed at which this journey could be made, then…it would seem that a voyager need only wait 690 years or so to make the journey in 100 years or less (i.e. at a speed of 6/100 the speed of light). In other words, the star could be reached in well under a thousand years from now simply by waiting. Total time to destination is 690 years of wait + 100 years of travel = 790 years.
Barring unexpected breakthroughs, then, we get to Barnard’s star in roughly 800 years. Or should we wait even longer to launch as better technologies continue to emerge? Now it gets interesting: assuming continuous growth without such breakthroughs, there also comes a time when although growth continues to produce higher speeds, the waiting time for that growth is too long to make up the velocity difference.
After that minimum, the ship that leaves later arrives later. No getting to Barnard’s Star only to be greeted by those who launched a century after you did and got there first. Here’s Kennedy again, on a launch strategy for future mission directors:
If the civilisation has the capability to make several launches, then they could make use of the spread of arrival times to encourage individuals to leave on the basis that others would either be there first to welcome them or be following close behind bringing with them the future technologies.
And again:
…the wait calculation is crucial. Either side of the minimum, voyagers will arrive later than those who set off at the minimum. At the minimum wait time, growth will not catch the voyagers up during their journey. They will arrive to an unsettled destination, expecting others to follow, but not knowing if the vanguard of civilisation will appear on their horizon before much time has passed. If they leave before or after the minimum and find their destination still unsettled when they arrive, they will know that growth has slowed or stopped and that they will be alone for some time.
This is a rich paper that weaves economic growth patterns with the pace of technology over time and takes a sober look at how our culture might adapt to the possibilities of long-term missions. I wrote in a 2004 entry about van Vogt’s classic “Far Centaurus” story (Astounding, Jan. 1944) as an example of travelers being caught by faster technologies, but there are a number of related scenarios that science fiction writers could mine by pondering the equations in this paper. I was also interested to learn in an e-mail from Kennedy that he has a second paper on the subject in the works. We’ll look at it here when it appears.
by Paul Gilster | Nov 22, 2006 | Exotic Physics |
More on growth scenarios for interstellar flight soon. But I don’t want to let the recent dark matter news get past us, so a quick nod to the University of California at Santa Cruz, where researchers have run a powerful computer simulation to probe the dark matter halo that evidently surrounds our Milky Way. It’s a further step toward understanding the stuff that makes up 82 percent of the matter in the universe, and that in turns helps us see how the large scale structure of the cosmos has evolved.
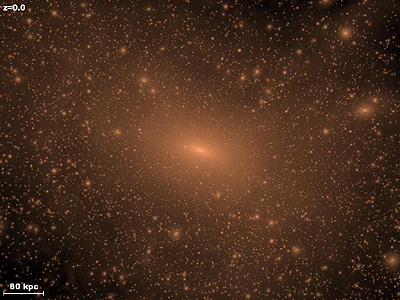
Image: Density map of dark matter in a halo the size of the Milky Way galaxy’s dark matter halo. Credit: J. Diemand, UC-SC.
I grew up in a time when it was thought that everything in the cosmos was explicable through gravitational forces produced by objects we could see. From solar systems on up to galaxies, it made sense — and the textbooks did this quite neatly with colorful diagrams — to show how matter found its way into configurations that would turn into celestial objects visible with our telescopes. Now we know the picture was wrong; the situation is far more complex and deeply mysterious.
For if dark matter — whatever it is — so vastly outnumbers matter we can see, then its interactions are crucial for the development of the earliest large-scale structure. Galaxies are inevitably affected by the gravitational wells created by this material, emerging in their distinctive shapes within spherical dark matter haloes. These haloes appear to be much larger than the galaxy they contain, and the new simulations testify as to how clumpy they are, with sub-haloes that themselves exhibit substructure variations. Says Piero Madau (UC-SC):
“We find almost 10,000 subhalos, about one order of magnitude more than in any past simulations, and some of our subhalos exhibit ‘subsubstructure.’ This was expected theoretically, but we have shown it for the first time in a numerical simulation.”
All this despite continuing problems fitting dwarf satellite galaxies — clumpy normal matter — into the dark matter picture. Future gamma-ray detection attempts may provide some answers, though until we have a better read on what dark matter actually is, we’re shooting in the dark.
Centauri Dreams‘ take: The gamma ray connection has continued to bother me — how could missions like GLAST (the Gamma Ray Large Area Space Telescope) have a role to play in detecting dark matter? The best I can do on that is to quote this UC-SC news release:
Astronomers may be able to detect clumps of dark matter within the Milky Way’s halo with future gamma-ray telescopes, but only if the dark matter consists of the types of particles that would give rise to gamma-ray emissions. Certain dark matter candidates–such as the neutralino, a theoretical particle predicted by supersymmetry theory–could annihilate (that is, be mutually destroyed) in collisions, generating new particles and emitting gamma rays.
That’s a big if, of course, but a sound way for investigation to proceed.
The paper is Diemand et al., “Dark matter substructure and gamma-ray annihilation in the Milky Way halo,” accepted for publication in The Astrophysical Journal and available online as a preprint.
by Paul Gilster | Nov 21, 2006 | Culture and Society |
In a book so stuffed with insights and quirky oddments that it belongs on the shelf of anyone interested in interstellar flight, Carl Sagan and I.S. Shklovskii once made a stunning calculation. Their 1966 volume Intelligent Life in the Universe (San Francisco: Holden-Day) presents the argument that with an average annual growth rate of just 1/3 of one percent, energy demand will outstrip the total solar radiation falling on the Earth by a factor of 100,000 within 2500 years. The re-building of the Solar System into something like a Dyson sphere may be inevitable.
But isn’t it a pipe dream to assume that growth will continue at even these modest levels? That was certainly my initial response, for so many things can throw a spanner into a civilization’s works. But Andrew Kennedy (The Chronolith Project, Seville Spain) takes a hard look at growth issues in a recent paper with interesting results. Kennedy believes that growth is far more tenacious than generally accepted. Economic collapse? Ponder that the average recession time in past centuries was between 8-10 years, whereas all our recessions since the Great Depression are better measured in months rather than years.
Disease? Even the Black Death in Europe exerted only a temporary brake on growth. Figuring a death rate of perhaps one-third of the population (the figure varies widely depending on the source), the population did not rise to pre-1349 levels until 1600. Both warfare and trade were severely reduced. But note what happened next:
…150 years or so afterwards, America had been discovered, improved ship-building and navigation (using the recent Western version of the compass) made journeys to the Far East commonplace, ships had sailed around the world (in AD 1521), and Europe was trading with China and India. The spread of credit-based banking and the invention of double entry bookkeeping had brought investment funds into play, and warfare had picked up again.
Kennedy’s examples from both Europe and the Americas are too numerous to list, but I found his thoughts on climate change interesting. The ‘Little ice Age’ that began at the start of the 14th century produced widespread famine and economic contraction, raising the price of English wheat to six times the norm amidst squalor and desolation. But by 1330, Edward III was turning castles from military installations into palaces even as war continued on the Continent. Wealth was being accumulated, great cathedrals built and the shipping industry revitalized in a trade renaissance fueled by spices, silks, wines and furs. The Bay of Biscay became known as the ‘sea of the English.’
Can the momentum continue? As a medievalist by training, I find Kennedy’s optimism about growth hard to swallow, but then my worldview was shaped by the notion of fortune’s wheel continually turning, as medieval a conceit as is imaginable (though passed along from Rome via Boethius). Nonetheless, the growth argument has a place in interstellar discussions, and Kennedy’s purpose is to apply it to the broader issue of how long an interstellar voyage should be delayed given the expectation that further growth will produce faster spacecraft that will catch the original one.
We’ll look at how Kennedy handles this again shortly, and I also want to bring in the thoughts of Marc Millis, who addressed these issues at the latest New Trends in Astrodynamics conference in Princeton. I have that presentation here and want to get into the meat of an argument that was so neatly captured by A.E. van Vogt in his story “Far Centaurus,” in which interstellar travelers finally reach the Centauri stars only to find that Earthmen with faster ships have landed long before them. Millis calls this issue ‘Zeno’s paradox in reverse.’ More about Zeno, paradoxes and Centauri missions as we move past the Thanksgiving holiday here in the States.
The paper is Kennedy, “Interstellar Travel: The Wait Calculation and the Incentive Trap of Progress,” Journal of the British Interplanetary Society Vol. 59, No. 7 (July, 2006), pp. 239-247.
by Paul Gilster | Nov 20, 2006 | Culture and Society, Sail Concepts |
Those who ponder the Fermi Paradox might want to consider Myrhaf’s solution, one based on economics. If advanced technolgical civilizations really are out there, maybe they simply can’t afford to build interstellar spacecraft. Myrhaf assumes that the only realistic way to travel between the stars is via a slow generation ship, what Isaac Asimov once called a ‘spome’ or ‘space home.’ And he doubts anyone would attempt it.
Expensive? You bet. And maybe there’s no one with the deep pockets to build it. Governments are too inefficient, while capital investment is unlikely because interstellar travel has such a long timeline. Corporate heads looking for return on their investments aren’t likely to have enough patience for a slow boat to Centauri. Charity? Perhaps there’s a hope through what Myrhaf calls ‘committed visionaries,’ but we’re talking investment over the course of generations.
Does any culture have that kind of long-term vision once it develops the technologies that could build a generation ship? There’s a case to be made that by the time the tools are available, the will won’t be there, and thus the solution to the paradox is what Myrhaf says: “Where are the aliens? They’re at home watching TV. When their visionaries knock on the door, they say, ‘I gave at the office,’ then resume watching ‘Alien Idol.'”
Or maybe not. Posit this scenario: A culture at the end of its star’s life must make a decision about how to save itself. Its G-type star, much like our Sun, will swell into a red giant, destroying all life on the inner worlds of its system. But in the process, that star becomes the perfect launching pad for solar sail missions of enormous scope, the kind that could get a generation ship on its way.
Would government, private industry and charity all contribute toward a starship not for exploratory purposes but for survival? The betting here is yes, and in a galaxy filled not with Sagan’s million technological civilizations but perhaps five or ten, star-crossing expeditions like these would hardly be visible to astronomers on Earth. Myrhaf may be right about the cost and the political will, but sheer self-preservation may eventually get at least a few societies to other stars.
One other thought: never rule out the power of compound interest. Couple it with a truly long-term perspective — think centuries instead of single lifetimes — and philanthropy properly applied can work wonders.
by Paul Gilster | Nov 18, 2006 | Exoplanetary Science |
If we’re lucky, the COROT mission, to be launched December 21, will be the first to detect rocky planets not much larger than the Earth around other stars. We’ve looked at COROT recently, and discussed how it and the Kepler space telescope will use transit methods to find these distant worlds. But as you go beyond Kepler (to be launched in 2008), the need for new technologies becomes apparent, which is why planet-finder designs like New Worlds are so significant.
Now I see that Claude Catala (Observatoire de Paris-Meudon) is proposing a new take on space-borne telescopes for this purpose. Catala suggests a survey that would gather light from literally hundreds of 10-centimeter telescopes working in tandem (COROT itself is built around a single 27-centimeter telescope). These are small instruments, to be sure, and in some ways less impressive than high-end amateur equipment now on the market. But each boasts a wide field of view, roughly 60 times that of the full Moon.
And that helps, because transit detections require monitoring as many stars as possible to find the small percentage aligned properly to Earth. What Catala is thinking is that each of these tiny telescopes could provide interferometry data to a computer aboard the spacecraft. The cumulative effect would be to detect transits over a wide swath of sky. Think of this as a tuned-up Kepler, and a transitional mission between it and later, direct imaging flights like the ESA’s Darwin and, let’s hope, New Worlds.