Centauri Dreams
Imagining and Planning Interstellar Exploration
DESTINY+: Mission to 3200 Phaethon
With successful operations at Ryugu (Hayabusa2) and Bennu (OSIRIS-REx), asteroid exploration seems to be moving full tilt, with the prospect of surface samples on the way. We can also look ahead to 16 Psyche, the object of interest for a NASA mission planned to launch in 2022, and the Lucy mission to Jupiter’s trojan asteroids, with launch now scheduled for 2021. The latest asteroid entry comes in the form of an interesting collaboration between the Japan Aerospace Exploration Agency (JAXA) and the German Aerospace Center (DLR) targeting asteroid 3200 Phaethon, a flyby mission designed to launch no earlier than 2024.
DESTINY+ is its name, the acronym standing for Demonstration and Experiment of Space Technology for INterplanetary voYage with Phaethon fLyby and dUst Science (try to say all that quickly before you’ve had your morning coffee). The agreement for the bilateral mission was signed on November 11 as part of a joint strategy dialogue between the two space agencies.
The new mission continues German and Japanese cooperation in space. You may recall that DLR and the French space agency CNES (National Center for Space Studies) developed the MASCOT rover that was deployed on Ryugu in 2018, with several papers already published on its findings. The BepiColombo mission to Mercury grows out of ESA and Japanese collaboration, while the proposed Martian Moons eXploration (MMX) mission, developed by JAXA, will include a rover developed by DLR and CNES, along with scientific instrumentation from NASA.
At Phaethon, we’re looking at an interesting object, an Apollo asteroid that appears to be the parent body of the Geminids meteor shower. The Apollo asteroids are the largest group of near-Earth objects, numbering over 10,000, but among them, Phaethon has a special claim to fame. Carsten Henselowsky is DESTINY+ project manager at DLR:
“With a minimum approach distance of approximately 21 million kilometres, Phaethon gets closer to the Sun than the planet Mercury. In the process, its surface heats up to a temperature of over 700 degrees Celsius, causing the celestial body to release more dust particles. The aim of the DESTINY+ mission is to investigate such cosmic dust particles and to determine whether the arrival of extraterrestrial dust particles on Earth may have played a role in the creation of life on our planet.”
Image: Animation of Phaethon’s orbit. Credit: Phoenix7777. Data source: JPL HORIZONS. CC BY-SA 4.0.
The flyby will take the spacecraft to within 500 kilometers of Phaethon at a time in its orbit when the asteroid is approximately 150 million kilometers from the Sun. Key to the success of the mission is the DDA dust analyzer built by DLR (DESTINY+ Dust Analyzer). The plan is to study the dust environment through the entire four-year cruise to Phaethon, with projected flyby in 2028. The DDA is, according to this recent presentation at the Europlanet Science Congress, “the technological successor to the Cosmic Dust Analyzer (CDA) aboard Cassini-Huygens, which prominently investigated the dust environment of the Saturnian system.”
With the goal of determining the origin of each dust particle, mission scientists will be focusing on the proportion of organic matter given the possible delivery of organics to the early Earth by such particles. The asteroid is on a highly eccentric orbit resembling that of a comet more than an asteroid, crossing the orbits of Mercury, Venus, Earth and Mars. Dust tails have been observed coming from the object, likely the result of solar heat creating surface fractures.
Launch will be aboard an Epsilon S vehicle from the Uchinoura Space Center in Japan. The spacecraft will use solar-electric propulsion, with a 1.5-year period after launch in which it will raise its orbit. A series of lunar flybys will then accelerate the probe into an interplanetary trajectory. The scientific payload will, in addition to the dust analyzer, include two cameras: The Telescopic Camera for Phaethon (TCAP) and the Multi-band Camera for Phaethon, MCAP.
By chance, I ran across a passage this morning in Oliver Morton’s book The Moon: A History for the Future (The Economist, 2019) that explains why it takes so long to get to objects that, like NEAs, sound as though they should be nearby. Such serendipity coaxes me into quoting it:
…what matters in space is delta-v, not distance. To get from the Moon to the Earth requires only about a fifth of the delta-v that is needed to make the same journey in the opposite direction. Another demonstration of this proposition is that the amount of delta-v it takes to get a spacecraft to the surface of the Moon can also get it to destinations much farther afield. “Near-Earth asteroids” (NEA) are only near inasmuch as they have orbits that very occasionally bring them moderately close to the Earth. At any given time a typical NEA will be 100 or 200 times as far away as the Moon. But in terms of delta-v, quite a lot of these asteroids are just as easy to reach as the Moon is; it is just a matter of getting on to the right trajectory and waiting. Indeed, it takes no more delta-v to reach the little moons of Mars than it does the great Moon of Earth (the surface of Mars is another matter).
Image: Asteroid explorer DESTINY+. Credit: JAXA/Kashikagaku.
Exoplanet Atmospheres: Keeping Up with ARIEL
How is a planet’s composition related to its host star? The European Space Agency’s ARIEL mission (Atmospheric Remote-sensing Infrared Exoplanet Large-survey) is designed to probe the question, examining planetary atmospheres to determine the composition, temperature and chemical processes at work in a large sample of planetary systems.
Transmission spectroscopy is the method, examining spectra as known exoplanets pass in front of, then behind their host stars. Researchers will use light filtering through the atmospheres to unlock the chemical processes within each. ARIEL will survey about 1,000 planetary systems in both visible and infrared wavelengths, probing not just chemistry but the thermal conditions that affect their composition. The mission’s focus is on super-Earths to gas giants, all with temperatures greater than 320 Celsius.
I suspect that principal investigator Giovanna Tinetti (University College London) has been asked about the choice of targets to the point of exhaustion, but one reason for focusing on planets in this size and temperature range is our need to build up a catalog of atmospheres that will inform the entire field, so that when we drill down to small, rocky worlds using future instruments, we’ll have a context in which to place what we see. A high temperature atmosphere is helpful here because it remains in continuous circulation, without the obscuring clouds that make characterization difficult.
Image: Giovanna Tinetti (University College London), principal investigator for ARIEL.
ARIEL will be positioned around L2, the second Sun-Earth Lagrange point, 1.5 million kilometres directly ‘behind’ Earth as viewed from the Sun. A just released ESA report explains the issues the mission will investigate, noting the wide range of planets and stars:
This large and unbiased survey will contribute to answering the first of the four ambitious topics listed in the ESA’s Cosmic Vision: “What are the conditions for planet formation and the emergence of life?”. Thousands of exoplanets have now been discovered with a huge range of masses, sizes and orbits: from rocky Earth-like planets to large gas giants grazing the surface of their host star. There is no known, discernible pattern linking the presence, size, or orbital parameters of a planet to the nature of its parent star. We have little idea whether the chemistry of a planet’s surface and atmosphere is linked to its formation environment, or whether the type of host star drives the physics and chemistry of the planet’s birth and evolution.
Remember, too, the statistical nature of the inquiry. The sample population is large, so that we move from the small number of atmospheres currently characterized to hundreds. Our understanding of the early stages of planet and atmosphere formation during the first few million years in an infant system as it emerges from the nebular phase should help us relate the chemistry in exoplanets to their other parameters and to the chemical environment of the star.
Image: An example spectrum Ariel could measure from light passing through an exoplanet’s atmosphere. Credit: ESA/STFC RAL Space/UCL/UK Space Agency/ATG Medialab.
Key components — water vapor, carbon dioxide, methane — of planetary atmospheres will be detected, but also more unusual metallic compounds that define the chemical environment within each system studied. For a smaller number of target planets, the spacecraft will perform a survey of cloud systems and atmospheric changes at a seasonal and daily level. Says ARIEL project scientist Theresa Lueftinger: “Our chemical census of hundreds of solar systems will help us understand each planet in context of the chemical environment and composition of the host star, in turn helping us to better understand our own cosmic neighbourhood.”
ESA has just announced that ARIEL has moved from study to implementation phase, the step before negotiations begin with industrial contractors to build the spacecraft, which is scheduled for launch from Kourou (French Guiana) in 2029. Bids on spacecraft hardware will be requested within months, with the prime contractor chosen by the summer of 2021. 50 institutes from 17 European countries are involved, as is NASA, in the payload module, which will have at its heart a one-meter class cryogenic telescope along with associated science instruments.
Three ESA missions with an exoplanet charter are thus framed within a ten-year window, with ARIEL joined by CHEOPS (CHaracterising ExOPlanet Satellite), launched in December 2019, and PLATO (PLAnetary Transits and Oscillations of stars), to be launched in 2026. The latter emphasizes rocky planets in the habitable zone of Sun-like stars.
To keep up with ARIEL, you may want to follow @ArielTelescope on Twitter. You’ll find background information in the recently published ARIEL Definition Study Report, available here.
We're taking an open approach in data access, even encouraging enthusiasts to help select targets & characterise stars. Much of the data will be available to the science community & public immediately.
(In the meantime check out our Data Challenges! ??)https://t.co/mEEvBrtO5s
— Ariel Space Mission (@ArielTelescope) November 16, 2020
A New Source for Plumes on Europa
How salty should we expect the ice on Europa’s surface to be? It would be helpful to know, because the salinity of the surface will be a factor in how transparent the ice shell is to radar waves. Europa Clipper will fly with an instrument called REASON — Radar for Europa Assessment and Sounding: Ocean to Near-surface — which will be investigating both the surface ice and the ocean beneath. Recent research, in which its principal investigator, Don Blankenship (University of Texas), is involved is offering insights into the salinity of the ice.
Here’s a bit of background on REASON from a NASA page on Europa Clipper:
Depending on their wavelength, radio waves can either bounce off or penetrate different materials. REASON will use high frequency (HF) and very high frequency (VHF) radio signals to penetrate up to 18 miles (30 kilometers) into Europa’s ice to look for the moon’s suspected ocean, measure ice thickness, and better understand the icy shell’s structure. The instrument will also study the elevation, composition, and roughness of Europa’s surface, and will search Europa’s upper atmosphere for signs of plume activity.
Image: Europa Clipper, scheduled for launch in 2024. Credit: NASA/JPL-Caltech.
So plumes are in play for Europa Clipper, and the new research finds evidence of a process that can produce them. The paper in Geophysical Research Letters focuses on what the authors call brine migration, in which small pockets of salty water migrate within the ice to warmer icy areas. We get into questions of salinity in this work because migration of icy brines, analyzed here through study of the impact crater Manannán, may have resulted in the creation of a plume. Imaging data from the Galileo mission allowed the team to study the resulting surface feature and to calculate that Europa’s ocean is about a fifth as salty as Earth’s.
But let’s back up to that plume. If eruptions from the ocean below periodically break through the ice, we might have a way to sample ocean materials without ever trying to drill through the surface shell. The new work, led by Gregor Steinbrügge (Stanford University) homes in on Manannán, a 30-kilometer wide crater on Europa that is the result of an impact some tens of millions of years ago. The paper models the melting and refreezing of water following the event. And it suggests that not all plumes carry materials from the ocean below.
Manannán is located on Europa’s trailing hemisphere at 3°0’N and 120°50’E. The crater was imaged by the Galileo spacecraft’s Near-Infrared Mapping Spectrometer. Subsequent geological mapping of Manannán has shown what appear to be impact melt materials filling the crater floor, along with ejecta deposits that would have been brought up from below at the time of impact.
According to the researchers, as water transformed back into ice following the impact, pockets of water with higher salt content were created in the surface, migrating sideways through the shell by melting adjacent areas of less salty ice. “We developed a way that a water pocket can move laterally – and that’s very important,” says Steinbru?gge. “It can move along thermal gradients, from cold to warm, and not only in the down direction as pulled by gravity.”
Image: This illustration of Jupiter’s icy moon Europa depicts a cryovolcanic eruption in which brine from within the icy shell could blast into space. A new model proposing this process may also shed light on plumes on other icy bodies. Credit: Justice Wainwright.
Pockets of brine moving about within the surface become saltier as they move through less salty water around them and eventually erupt, according to this model, which shows freezing and pressurization as the factors leading to a cryovolcanic event. At Manannán crater, a migrating brine pocket finally freezing at the center generated a plume estimated to be 1-2 kilometers high, leaving a surface feature, roughly spider-like in shape, that turned up in the Galileo data. The spider-shaped fractures consist of 17 segments, surrounded by a series of concentric faults. This would have been a relatively small plume, and the Manannán findings do not explain what may be larger plumes hypothesized based on Hubble and Galileo data.
Image: The ‘spider’ feature within Manannán impact crater. Credit: NASA.
The point is that brine pocket migration is a surface phenomenon that can generate plumes, implying that such plumes do not require a connection with the ocean beneath. Whether there are other, larger plumes that do make such connections has not yet been determined.
Oceanic origin or not, all of this makes Europa’s surface an even more dynamic place than we’ve been considering, while somewhat tempering our expectations for astrobiology in plume activity even as we continue to observe the moon for future, perhaps much larger plumes. The team’s modeling of how melting and subsequent freezing of a water pocket within the icy shell would produce an eruption should have implications for other icy bodies within the Solar System. Robert Pappalardo (JPL) is a project scientist on the Europa Clipper mission:
“The work is exciting, because it supports the growing body of research showing there could be multiple kinds of plumes on Europa. Understanding plumes and their possible sources strongly contributes to Europa Clipper’s goal to investigate Europa’s habitability.”
The paper is Steinbru?gge et al. ,”Brine Migration and Impact?Induced Cryovolcanism on Europa,” Geophysical Research Letters 5 November 2020 (abstract).
Radioactive Elements and Planetary Habitability
A planetary dynamo may be a key factor in creating the conditions needed for life. And creating that dynamo seems to depend on the radioactive decay of thorium and uranium, generating internal heating and driving plate tectonics. Let’s carry this line of thought further, though, as the authors of a new paper out of UC-Santa Cruz do, and point out that these heavy elements are necessary to create a magnetic field like Earth’s, which protects us from damaging radiation.
In the rocky planets, magnetic fields are generated by convection in a metallic core, which in turn is driven by heat extracted into the mantle (Nimmo 2015; Labrosse 2015; Boujibar et al. 2020). Since mantle radiogenic heat production controls how much heat is extracted from the core, it will also influence the presence or absence of a dynamo. Similarly, heat production will control the mantle temperature and thus the rate of silicate melting and volcanism.
That quote is from the paper, whose lead author is Francis Nimmo, and it points to the key role of radiogenic heat in the mantle. Within the Earth, there are enough radioactive elements to generate a persistent ‘geodynamo,’ in which molten iron and nickel in motion in the planet’s outer core produce electric currents, forming a magnetic field as the Earth rotates. The authors find that Earth “had just enough radiogenic heating to maintain a persistent dynamo.” And that has implications for the atmosphere.
UC-Santa Cruz’ Natalie Batalha is director of the university’s Astrobiology Initiative, whose multidisciplinary work is designed to produce the kind of widespread collaboration found in this paper. Says Batalha:
“It has long been speculated that internal heating drives plate tectonics, which creates carbon cycling and geological activity like volcanism, which produces an atmosphere. And the ability to retain an atmosphere is related to the magnetic field, which is also driven by internal heating.”
To ponder how all these effects come together, we have only to look at Mars, where the lack of a magnetic field can be tied to the gradual loss of an atmosphere that was once thick enough to support water on the surface and, potentially, a living environment. The planet’s low gravity was one factor in the gradual thinning of the atmosphere, but the solar wind of charged particles from the Sun is likewise a problem, for without a protective magnetic field, the atmosphere erodes.
We have an angle here into habitability on planets around other stars, for the amount of radioactive material is going to vary from one stellar system to another. The so-called r-process elements — created by nuclear reactions that are responsible for producing roughly half of the atomic nuclei heavier than iron — owe their origin to supernova events and the mergers of neutron star remnants, events that will be stochastic and result in planets with varying degrees of radiogenic materials. Back to the paper on this point:
From the point of view of long-term radiogenic heating, the most important elements are potassium, thorium, and uranium. Th and U are both r-process elements, most likely produced in neutron star mergers (NSMs) (Kasen et al. 2017; Cowan et al. 2019), with possibly significant contributions also from massive star collapse explosions (Siegel et al. 2019; Macias & Ramirez-Ruiz 2019). Because such events are rare (an occurrence rate of tens/Myr in our Galaxy) and produce large quantities of r-process elements, the resulting concentration of r-process elements should vary considerably. This is especially true of low-metallicity stars, because turbulent mixing of the interstellar medium is inefficient in the early Galaxy (Shen et al. 2015; Naiman et al. 2018). This expectation is in agreement with measurements of r-process europium in low-metallicity stars (e.g., Macias & Ramirez-Ruiz 2018) and with r-process abundances in dwarf galaxies (e.g., Ji et al. 2016).
Lead author Francis Nimmo realized that if planets vary in the amount of radioactive elements, we should then expect geological activity and magnetic fields to vary as well. The UC-Santa Cruz scientists essentially adjusted radiogenic heat production in their models to see how this factor affected planetary habitability. As Nimmo puts it:
“We took a model of the Earth and dialed the amount of internal radiogenic heat production up and down to see what happens. Just by changing this one variable, you sweep through these different scenarios, from geologically dead to Earth-like to extremely volcanic without a dynamo.”
When radiogenic heating is dialed up higher than the Earth’s, no dynamo is produced. With most of the thorium and uranium in the mantle, the excessive heat becomes an insulator, so that the loss of heat from the molten core is slowed. The result: Heat loss from the core cannot generate convection strong enough to produce a magnetic field, with all that implies about the survival of an atmosphere and radiation flux on the surface.
Given all that, the associated volcanic activity produced by greater levels of radiogenic internal heating is almost lost in the shuffle, but it could obviously be a factor in mass extinction events. And while that implies that high levels of radiogenic heating are a key factor in suppressing a habitable environment, the study also shows that too little heat results in no volcanism whatsoever. We wind up with a planet that, like Mars, appears geologically dead.
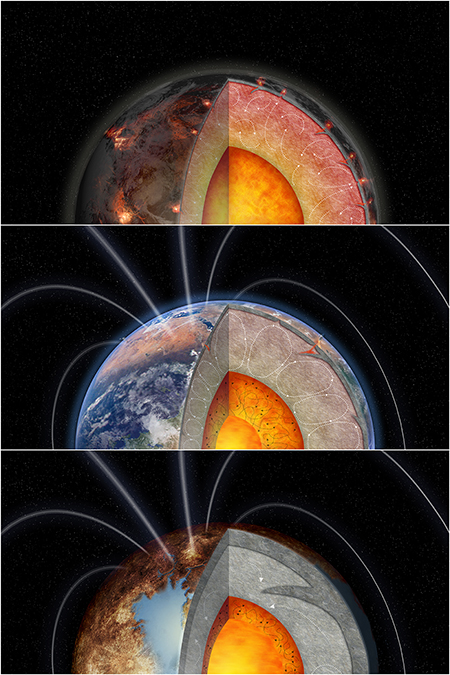
A new study identifies internal heating from radioactive decay as a critical factor in a planet’s ability to generate a magnetic field and retain an atmosphere. Credit: Illustration by Melissa Weiss for UC Santa Cruz
Image: These illustrations show three versions of a rocky planet with different amounts of internal heating from radioactive elements. The middle planet is Earth-like, with plate tectonics and an internal dynamo generating a magnetic field. The top planet, with more radiogenic heating, has extreme volcanism but no dynamo or magnetic field. The bottom planet, with less radiogenic heating, is geologically “dead,” with no volcanism. Caption credit: UC-Santa Cruz.
So we are working with a single variable, one that can be studied via spectroscopy to measure the abundance of the various elements in stars, the assumption being that planets will have the same composition as the stars they orbit. Here the rare earth element europium comes into play, readily observed in stellar spectra, and created by the same processes that produce thorium and uranium. In this work, europium is the tracer for studying radiogenic abundance.
We already have europium measurements for many nearby stars, which allowed Nimmo and team to set a range of inputs for their models of radiogenic heating, with the Sun’s composition establishing the middle of the range. Europium thus becomes a measurement that can flag radiogenic elements in exoplanetary systems as we look forward to the deployment of the James Webb Space Telescope. The europium measurement may well show us which stellar systems are likely to produce planets with the internal composition life would need to survive. From the paper:
…higher mantle heating rates increase volcanic activity and shut off the dynamo, both likely deleterious for habitability. In contrast, reduced mantle heating rates will at some point stop melt production. A potential consequence is that plate tectonics will then cease (Louren¸co et al. 2018), and with it, the dynamo, as at Venus (Nimmo 2002). It is tempting to speculate that the Earth is habitable in part because it possesses a “Goldilocks” concentration of radiogenic elements: high enough to permit long-lived dynamo activity and plate tectonics, but not so much that extreme volcanism and dynamo shutoff occur. As yet, however, our understanding of the complicated feedbacks involved is too rudimentary to make such a definitive conclusion; more detailed calculations, and better characterization of the radiogenic element abundances in planet-hosting stars, are both required.
The paper is Nimmo et al., “Radiogenic Heating and Its Influence on Rocky Planet Dynamos and Habitability,” Astrophysical Journal Letters Vol. 903, No. 2 (10 November 2020). Abstract / Preprint.
An Unusual (and Promising) Brown Dwarf Detection
The naming of names is quite a project when it comes to new astronomical objects, and given the sheer numbers — 300 million habitable planets around G- and K-class stars, for example — we might do better to stick with simple identifiers. On the other hand, it’s a bit charming that a new brown dwarf known by its identifier as BDR J1750+3809 has been dubbed ‘Elegast’ by the discovery team. This is the first substellar object found through radio observations.
The name is both appropriate and specific to the discovery space. Elegast appears in a poem in Middle Dutch (12th or 13th Century) called ‘Karel ende Elegast,’ with the character Elegast being a vassal of Charlemagne who seems to be king of the elves (Wikipedia to the rescue, vindicating once again my decision to send them a monthly donation).
The Dutch connection is that the radio work comes out of LOFAR (Low-Frequency Array), which is currently the largest radio telescope operating at the lowest frequencies that we can observe from Earth. Based in the Netherlands, LOFAR is an international effort with field stations in eight countries.
Elegast breaks from the norm in not being discovered from the kind of infrared sky surveys through which most other brown dwarfs have been found. You might recall our discussions of the WISE mission and the possibility, not realized, of finding a brown dwarf closer than the Alpha Centauri stars. But it’s also true that infrared surveys can’t detect objects that are sufficiently cold and faint, whereas we now have radio wavelengths to fill the gap.
Is there still some faint possibility of a closer brown dwarf? T-class dwarfs are defined as being less than 1500 Kelvin, but WISE identified Y-dwarfs with temperatures as cool as 25 °C (298 K), which gets us down to where my thermostat is set as I write this. WISE 1828+2650, I subsequently learned, is so cold that it emits no visible light at all and is more or less indistinguishable from a free-floating planet. Get any colder and infrared surveys won’t find such worlds, which is why the news about Elegast is so encouraging.
Given that the population of so-called ‘rogue’ planets may be vast, it’s wonderful to see a new method becoming available with which to study nearby examples. Brown dwarfs may not be able to create the kind of fusion reactions found in stars like the Sun, but they emit at radio wavelengths just as gas giant planets do. Jupiter’s emissions are well studied, the result of powerful magnetic fields around the planet accelerating charged particles to produce radio waves as well as aurorae. Brown dwarfs are faint at these wavelengths but detectable.
Image: Artist’s impression of Elegast. The blue loops depict the magnetic field lines. Charged particles moving along these lines emit radio waves that LOFAR detected. Some particles eventually reach the poles and generate aurorae similar to the northern lights on Earth. Credit: ASTRON / Danielle Futselaar.
The LOFAR observations had to contend with background galaxies when investigating potential brown dwarfs. The key turned out to be identifying signals that were circularly polarized, which distinguishes planets and brown dwarfs from the background ‘noise.’ What this kind of polarization means is that the electromagnetic field of the radio waves rotates in a characteristic circular pattern. Says LOFAR project scientist Tim Shimwell: “We could not have picked out Elegast in our standard radio images from among the crowd of millions of galaxies, but Elegast immediately stood out when we made circularly polarised images.”
In the case of Elegast, the Gemini-North Telescope had produced archival imagery used to confirm the discovery, and the NASA Infrared Telescope Facility (IRTF) deployed an infrared spectrometer known as SpeX, recently upgraded, to obtain a spectrum of Elegast. The signature of methane, common in brown dwarfs and gas giant planets, was revealed.
Beyond its possibilities at identifying the faintest brown dwarfs and free-floating planets, radio astronomy may also be used to study exoplanet magnetic fields, testing theories on magnetic field strength on objects that have enough similarities to gas giants to make the investigation worthwhile. The team is now making follow-up observations on Elegast to measure its magnetic field even as they use LOFAR to look for more such objects. Harish Vedantham, an astronomer at the Netherlands Institute for Radio Astronomy (ASTRON) is lead author of the study:
“Our ultimate goal is to understand magnetism in exoplanets and how it impacts their ability to host life. Because magnetic phenomena of cold brown dwarfs like Elegast are so similar to what is seen on solar system planets, we expect our work to provide a vital datapoint to test theoretical models that predict the magnetic fields of extrasolar bodies.”
The paper is Vedantham et al., “Direct radio discovery of a cold brown dwarf,” Astrophysical Journal Letters Vol. 903, No. 2 (9 November 2020). Abstract / Preprint.
Europa: Night-time Glow a New Tool for Analysis
When it comes to Europa, it’s the surface that counts as we try to learn more about the ocean beneath. Maybe one day we’ll be able to get some kind of probe through the ice, but for now we have to think about things like upwellings of water that percolate up through cracks in the frozen landscape, and unusual areas like Europa’s ‘chaos’ terrain. Here, fractures and evident ‘rafts’ of ice show disruptions where the icy surface of the moon experiences Jupiter’s tidal effects.
Image: The surface of Jupiter’s moon Europa features a widely varied landscape, including ridges, bands, small rounded domes and disrupted spaces that geologists called “chaos terrain.” This newly reprocessed image, along with two others along the same longitude, was taken by NASA’s Galileo spacecraft on Sept. 26, 1998, and reveals details of diverse surface features on Europa. Credit: NASA/JPL-Caltech/SETI Institute.
What kind of materials might we find frozen into the cracks and grooves of such terrain? Europa Clipper will doubtless tell us more as it updates Galileo’s two-decade old data. Meanwhile, we recently learned about another way to study the moon’s surface composition. At the Jet Propulsion Laboratory, Murthy Gudipati and team have been doing laboratory work that informs our understanding of how Europa reacts to the inflow of high-energy radiation from Jupiter. The high flux of charged particles occurs because of interactions with Jupiter’s magnetic field.
The key to the work is the fact that different salty compounds produce their own unique signature as they respond to this radiation bath. A glow emerges — sometimes green to the eye, sometimes edging into blue or white — depending on the materials in question. Gudipati’s team used a spectrometer, as we would expect, to study the surface, but here the observations are taking place not with reflected sunlight on the dayside but Europa’s own glow at night. The paper on this work, which appeared in Nature Astronomy, shows us how much data could be gathered by a method that seems to have surprised the scientists involved.
JPL co-author Bryana Henderson explains:
“[W]e never imagined that we would see what we ended up seeing. When we tried new ice compositions, the glow looked different. And we all just stared at it for a while and then said, ‘This is new, right? This is definitely a different glow?’ So we pointed a spectrometer at it, and each type of ice had a different spectrum.”
Image: This illustration of Jupiter’s moon Europa shows how the icy surface may glow on its nightside, the side facing away from the Sun. New lab experiments re-created the environment of Europa and find that the icy moon shines, even on its nightside, due to an ice glow. As Jupiter bombards Europa with radiation, the electrons penetrate the surface, energizing the molecules underneath. When those molecules relax, they release energy as visible light. Variations in the glow and the color of the glow itself could reveal information about the composition of ice on Europa’s surface. Different salty compounds react differently to the radiation and emit their own unique glimmer. Color will vary based on the real composition of Europa’s surface. Credit: NASA/JPL-Caltech.
The process seems straightforward, but sometimes it takes replicating known conditions as closely as possible in a lab to discover the consequences. Start with ice mixed with salts like magnesium sulfate and sodium chloride. Bathe the mix in radiation. The glow is a natural result, its variations linked to different compositions of the ice. Those variations are what gives this work implications for missions like Europa Clipper.
Sodium chloride brine, for example, turned out to produce a lower level of glow in the team’s setup, which used an instrument built at JPL known delightfully as ICE-HEART (Ice Chamber for Europa’s High-Energy Electron and Radiation Environment Testing). The experiments were run at a high-energy electron beam facility in Gaithersburg, Maryland once ICE-HEART had been taken there, the original plan being to study organic material under the Europan ice. The continuous glow on Europa’s night side now emerges as a source of future data, one that can be compared with these laboratory results to identify salty components on the surface.
“It’s not often that you’re in a lab and say, ‘We might find this when we get there,'” Gudipati said. “Usually it’s the other way around – you go there and find something and try to explain it in the lab. But our prediction goes back to a simple observation, and that’s what science is about.”
So Europa Clipper can use night-time flybys to delve into the chemical composition below, a method that may turn out to be helpful on other Galilean moons, or wherever high doses of ionizing radiation sleet down upon a frozen surface. Specific to Europa Clipper’s instruments, we learn the following:
Observation of night-time, high-energy, radiation-induced ice glow on the trailing hemisphere could thus provide more constraints on the chemical composition of non-ice material, revealing whether sulfates or chlorides are present, what their counterions could be, and whether pure water-ice patches are detectable. Dark regions could imply sodium- and chloride-dominant surfaces, whereas brighter regions could imply magnesium- and sulfate-dominant surfaces in the absence of water ice. The presence or absence of water ice could unambiguously be determined by the MISE [Mapping Imaging Spectrometer for Europa] instrument, designed to span a wavelength range of 0.8 μm to 5 μm, covering ice absorption features in the daytime.
In addition, the glow of night-time ice can be measured against daylight observations from Europa Clipper’s Wide Angle Camera and the MISE spectrometer to dig deeper into the chemical composition below. Some ultraviolet features of the night-time spectra will allow mission scientists to flag temperature anomalies on the surface for future observations.
The paper is Gudipati et al., “Laboratory predictions for the night-side surface ice glow of Europa,” Nature Astronomy 9 November 2020 (abstract).