by Paul Gilster | Nov 30, 2006 | Antimatter, Culture and Society |
About to receive the Royal Society’s Copley Medal, Britain’s highest scientific award, Stephen Hawking told a BBC radio audience that if the human race were to survive, it would be necessary to go to another star. Here’s a quote from a story on this in the Daily Mail:
“The long-term survival of the human race is at risk as long as it is confined to a single planet… Sooner or later, disasters such as an asteroid collision or nuclear war could wipe us all out. But once we spread out into space and establish independent colonies, our future should be safe. There isn’t anywhere like the Earth in the solar system, so we would have to go to another star.”
Hawking acknowledges the immense problems, telling his interviewer that chemical rockets like the Saturn V used on Apollo would require tens of thousands of years to reach Alpha Centauri. And while he has an admiration for Star Trek‘s warp drive (and is quite a fan of the series, as Trekkies know), Hawking pins his hopes on antimatter, forseeing future technologies that might reach speeds equal to a high percentage of the speed of light.
Alpha Centauri in six years? Not bad, and if you can get to .86 c, you’ve created time dilation that reduces the travel time for those aboard to three years. That’s just the figure used by physicist and science fiction writer Gerald Nordley when I talked to him in 2004 about a viable manned star mission. Nordley’s view: The first humans will reach Alpha Centauri in about the same time it took Magellan’s crew to circle the Earth — three years. That’s three years shipboard time, six as seen by observers on Earth, and puts Nordley and Hawking very much on the same page.
That the odds on survival here on Earth may be diminishing doesn’t seem to be just Hawking’s view. Consider James Lovelock, also quoted in a Daily Mail story, who points to climate change as the culprit in the coming reduction of Earth’s population from 6.5 billion to 500 million. Lovelock, you may remember, first proposed the Gaia Hypothesis, the notion that the Earth is a balanced system whose parts, like a human body, all mesh to create conditions possible for life.
Speaking to the Institution of Chemical Engineers in London, Lovelock had this to say:
“There is very good evidence of what happened 55 million years ago when as much carbon dioxide was put into the atmosphere by geology as is being done by us now. Temperatures zoomed up by 8 degrees and stayed there for 200,000 years then came back to normal.”
Go back 55 million years and you’re in the tropical era known as the Eocene. If we are about to reproduce Eocene-like conditions, is there anything we can do about it? Lovelock thinks that attempts to remedy ‘global heating,’ as he calls it, are simply buying us time, but doubts the entire human race will be destroyed, with survivors in arctic refuges possibly undergoing new evolution. That’s an outcome quite similar to what happens in Ronald Wright’s unsettling novel A Scientific Romance. Whether it’s true or not, I’m with Hawking in hoping for the interstellar propulsion breakthrough that can offer us a refuge just in case.
by Paul Gilster | Nov 29, 2006 | Exoplanetary Science |
Tau Boötis, a billion year old star some 50 light years from Earth, would be a fascinating place to see up close. The star is orbited by a gas giant some 4.4 times Jupiter’s mass, one of those ‘hot Jupiters’ that close to improbably tight distances with the primary. In this case, the planet/star separation is a mere 0.049 AU, making it about 5 percent of the distance between the Earth and the Sun.
‘Hot Jupiters’ are bound to be dramatic objects anyway, but this one has other attributes. A team of French astronomers has been able to measure the magnetic field of Tau Boötis itself, finding it just slightly larger than the Sun’s. That’s the first direct measurement of the magnetic field of a star hosting an exoplanet, and it sets up interesting studies of the interactions between the two bodies as the planet moves so breathtakingly close to its star.
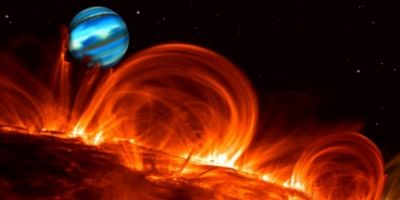
Image: An artist’s conception of the giant exoplanet orbiting Tau Boötis through the star’s magnetic archs. Credit David Aguilar, CfA.
In particular, the revolution of the planet shows it to be synchronized with a rotating band of material on the star’s surface, suggestive of all kinds of interactions between magnetosphere and planet. A key factor in stellar magnetic fields is the fact that parts of the star rotate at different rates. In fact, matter at the equator of Tau Boötis rotates 18 percent faster than matter located at the poles.
We can suspect from all this that a lot is going on here, and it should trigger a memory. Remember Voyager’s early images of tortured Io? One thing we learned about the pizza-like Jovian moon was that it orbited well within Jupiter’s magnetic field, contributing to the charged particles in that field and concentrating them in what we came to call the ‘Io torus,’ a doughnut-shaped band surrounding Io’s orbit. Huge electrical currents are created between Jupiter and Io, and we can imagine similar exotic effects occurring around the distant star.
This first direct measurement should contribute to our understanding of how magnetic fields affect planetary development, perhaps giving us clues to why planets like this one make migrations into the extreme inner systems around their star. The paper is Catala et al., “The magnetic field of the planet-hosting star tau Boötis,” soon to appear in Monthly Notices of the Royal Astronomical Society and available in preprint form.
by Paul Gilster | Nov 28, 2006 | Culture and Society, Missions |
We seem to have accepted in our time the notion that technology always moves forward. But a key factor in the Drake Equation, that long and interesting conjecture that parses the possibilities for extraterrestrial life, is the question of whether technological societies have an average lifetime. Do they invariably survive to reach the stars, or do they destroy themselves before this is possible?
Listen to something Fred Hoyle said back in 1964:
It has often been said, if the human species fails to make a go of it here on Earth, some other species will take over the running. In a sense of developing high intelligence, this is not correct. We have, or will have, exhausted the necessary physical prerequisites so far as this planet is concerned. With coal gone, oil gone, high-grade metallic ores gone, no species however competent can make the long climb from primitive conditions to high-level technology. This is a one-shot affair. If we fail, this planetary system fails so far as intelligence is concerned. The same is true of other planetary systems. On each of them, there will be one chance, and one chance only.
The reference is from Hoyle’s Of Men and Galaxies (Seattle: University of Washington Press), and I ran into it in Andrew Kennedy’s paper on ‘the wait calculation,’ which we’ve been discussing here recently. Hoyle’s take is controversial, to say the least, but it underlines the long-range issues we often focus on in these pages. Do we have the capacity, for example, to solve intractable problems over the course of centuries when this would involve sacrifice on the individual level during our comparatively short lifetimes?
Think long-term about interstellar missions and you see the limitation. In a recent presentation at Princeton, Marc Millis laid out the support levels that might be expected for various human ventures into the cosmos. A mission time of 3-5 years could attract the interest of power brokers in government and industry. A 20-year mission could still capture a significant portion of the populace. But by the time you hit 50 years — and these are the time frames being discussed for Kuiper Belt missions or missions to the gravity focus — your potential audience is reduced largely to the professionals involved.
Millis ponders these things because he is former head of NASA’s Breakthrough Propulsion Physics program and founding architect of the Tau Zero Foundation. And he knows that it has become all but axiomatic that a mission cannot be longer than the lifetime of the scientists who sent it. A 100-year long mission gains virtually no public interest (though still piquing the attention of the scientists most closely involved with the target in question). Mission times over 100 years become problematic in every way, requiring a societal commitment that we haven’t seen since the cathedrals of the Middle Ages or the pyramids of the Egyptian pharaohs. In both those eras, multi-generational projects were undertaken and brought to fruition because of a broader conception of man’s place in history than seems possible today.
On a visit to Marshall Space Flight Center, I once asked NASA’s Les Johnson whether society would ever send a 1000-year mission to another star. I was really asking about the social will to do such a thing, but Johnson took the question more practically. “I’d love a thousand years,” Johnson replied. He meant that we’re nowhere near having the capability of launching even a mission of that length, much less getting those numbers down to a few centuries.
So what are reasonable mission goals? Millis laid out some key numbers at Princeton that serve us well. Get to 6 percent of light speed and Centauri becomes feasible as a 76-year mission, while within 15 light years of Earth (and 265 years of flight time) are five systems that look intriguing from the perspective of habitable planets. Jump to 25 percent of light speed and Centauri is reachable in 22 years (no worse than the Voyagers’ continuing mission), with fully 20 other systems in range in a 100-year time frame.
What throws a wrench into the machinery? Three possibilities, one being the discovery of a nearer target (i.e., a brown dwarf closer than Centauri or something of that nature). Another is a physics breakthrough that dramatically changes mission times (Millis calls this ‘incessant obsolesence’). But the third is the one we seldom discuss: perhaps the pace of technology actually slows, and becomes longer than the actual mission time. At that point, there is no thought of waiting to launch, because no faster mission will get to the target first. In fact, the longer we wait, the worse the chances of ever launching.
If Hoyle is right, a major variable in all this is that technological growth may falter entirely, with terminal exhausion of a planet’s resources. Circle back to Fermi and you get a solution to the question ‘where are they’ that technology optimists like myself don’t like to consider. We don’t see extraterrestrials because technological societies are rare. And they’re rare because most such societies destroy themselves before star missions ever become viable. It’s a bleak assessment but something to ponder as we consider our ability to plan and carry out projects over the lifetimes not just of researchers but civilizations.
by Paul Gilster | Nov 27, 2006 | Exoplanetary Science |
We’ve identified over 200 planets around other stars, but in many ways we know little about other solar systems. The problem is in extrapolating from our knowledge of one or two planets to an entire planetary system, much of which we cannot detect. Can we expect to find gas giants mixed with small terrestrial worlds around most Sun-like stars? And what about the smaller and far fainter red dwarfs? Clearly, the job of characterizing not just planets but entire systems is going to occupy astronomers for many a decade.
A new paper from the California & Carnegie team takes helpful steps in that direction. New planet finds are always fascinating, and the team does have four of them, the highlight being the pair orbiting the Sun-like star HIP 14810. Greg Laughlin (UC-SC) writes about that system on the systemic site, noting this:
The fact that the orbit is clearly non-circular would be strong evidence for the presence of planet c, even if there weren’t enough data to detect c directly. If planet b was the only significant planet in the system, its orbit would have circularized via tidal dissipation on a timescale that is less than the age of the star.
I mention Laughlin’s comment because it bears on where we are in the planet hunting process. The radial-velocity measurements that have found most known exoplanets depend on collecting data about how a star wobbles as it is gravitationally influenced by the planet(s) around it. Clearly, larger planets close in to their primary star are going to be the first seen with this method because they affect the star more clearly in short time frames.
But as we collect data over longer and longer periods, we begin to see more subtle signatures in the data that can point to other planets. Gas giants in outer system orbits eventually get teased out of the noise, and most intriguingly of all, we start to look for smaller worlds that may be terrestrial in nature. The California & Carnegie team points out that the mass distribution of planets is thought to increase steeply as we move into the range of lower masses. And that means that there are probably a large number of planets in systems already identified that are accompanied by other worlds.
So here are some conclusions from the study: The number of known multiple-planet systems is 22, and the number of exoplanet-bearing stars with trends in the radial velocity data that point to additional planets is also 22. The overall number of nearby stars bearing planets is 152. From the paper:
This means that 30% of known exoplanet systems show significant evidence of multiplicity. Considering that the mass distribution of planets increases steeply toward lower masses…, our incompleteness must be considerable between 1.0 and 0.1 Jupiter-masses. Thus, the actual occurrence of multiple planets among stars having one known planet must be considerably greater than 30%.
And backing out to a wider view:
From an anthropocentric perspective, this frequency of multiplicity suggests that in some respects, the Solar System is not such an aberration. Our Sun has 4 giant planets, and it appears that such multiplicity is not uncommon, although circular orbits are.
The news keeps getting more interesting the more time elapses, for as data accumulates, the planet hunt for a given star becomes that much more precise. The California & Carnegie team points out that its search is only now becoming sensitive to planets like Jupiter in 12-year orbits. Finding a true analog to Saturn would demand another 15 years of observation. It’s hard to be patient when the ultimate goal for some of us is a terrestrial-sized world in a star’s habitable zone, but every passing day yields that much more information that can tell us what other solar systems really look like.
The paper, which will run in The Astrophysical Journal in February, is Wright et al., “Four New Exoplanets, and Hints of Additional Substellar Companions to Exoplanet Host Stars,” now available online in preprint form.
by Paul Gilster | Nov 25, 2006 | Deep Sky Astronomy & Telescopes |
It’s a gorgeous day in the mid-Atlantic states following one of the most colorful autumns in memory. Most of the leaves are down now, which gives me plenty to do. I had intended to look at the new paper from the California & Carnegie planet finder team, but the great outdoors beckons. Instead, we’ll examine their latest next week, along with more on interstellar flight scheduling with reference to Marc Millis’ recent presentation at Princeton and an intriguing Fred Hoyle insight.
For today, here’s a quick post on how adaptive optics can sharpen a telescope’s view. In the image below, you’re seeing two views of the part of the Orion Nebula known as the Trapezium. The Subaru telescope on Mauna Kea (Hawaii), working without adaptive optics, obtained the image on the right back in 1999. The image on the left shows the effect of a new laser guide star system and enhanced AO, and as you can see, the difference in detail is remarkable. Be sure to click to enlarge both views.
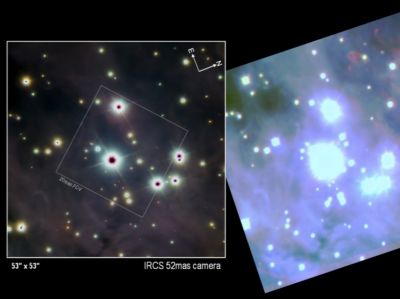
Image: The trapezium region in the Orion Nebula with and without adaptive optics. The image on the right has a resolution of 0.6 arcseconds and was obtained with Subaru’s CISCO camera in 1999 without the use of adaptive optics. The image on the left has a resolution of 0.06 arcseconds and was obtained in October 2006 using Subaru’s IRCS camera and the new adaptive optics system. Credit: Subaru Telescope/National Astronomical Observatory of Japan.
What we’re starting to see from Earth-based observatories would have astonished an earlier generation of astronomers. The new system greatly boosts Subaru’s capabilities over an earlier AO installation. Its laser guide creates an artificial star high in the atmosphere, the light from which can be measured to gauge atmospheric distortion. The adaptive optics system then adjusts the shape of its deformable mirror 1000 times a second to erase the extraneous effects — no more ‘twinkling’ star.
In essence, being able to produce an artificial ‘star’ for measurements anywhere in the atmosphere means that observing teams aren’t limited to regions near existing stars. They can observe any part of the sky they choose, and when they do lock onto a star or distant galaxy, the observatory’s new 188-element wavefront sensor improves Subaru’s view by a factor of ten. One thing’s for sure: if we ever do launch an interstellar probe, installations like these and upcoming space-based systems like Kepler and COROT will sharpen our view of its target.