by Paul Gilster | Jan 31, 2007 | Deep Sky Astronomy & Telescopes |
With its Wide Field Planetary Camera 2, Near Infrared Camera Multi-Object Spectrograph (NICMOS), and Fine Guidance Sensors still operational, the Hubble Space Telescope isn’t exactly blind. But the loss of the Advanced Camera for Surveys would be a serious one, and the bad news is that the next servicing mission, scheduled for mid-2008, probably won’t be able to fix the problem. ACS lived out its five year operational life but dazzling vistas like the Ultra Deep Field make us yearn for more.
Hubble’s other instruments are still doing good science. Recall that the UDF itself is actually made up of images from the ACS and NICMOS, leading Centauri Dreams to believe that many observational programs will go forward after adjusting to the change in instrumentation. As always, we make a virtue of necessity, a phrase first recorded by Chaucer that resonates even now in the realm of deep space exploration.
Meanwhile, we receive more positive news from the testing of the James Webb Space Telescope’s backplane (its ‘spine’), which supports the mirrors of the telescope and has been pronounced fit by project engineers. A recent freezing test took place at Marshall Space Flight Center in Huntsville, checking down to the nanometer level to ensure that the backplane will hold steady during space observations. Testing down to 30 degrees Kelvin (-405 degrees Fahrenheit) has already taken place, a step toward shaking out all the telescope’s key technologies.
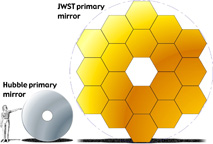
A few points of comparison between JWST and Hubble: The JWST will do most of its work at infrared wavelengths. Hubble can do some infrared work as well, but its primary mission is at optical and ultraviolet wavelengths. As to light gathering surface, JWST really is Hubble’s successor. Its 6.6 meter primary mirror significantly upgrades Hubble’s 2.4 meter one; in fact, JWST boasts about seven times the collecting area. And as compared to the Spitzer Space Telescope, which also works in the infrared, JWST offers much higher resolution. Finally, Hubble is in a near-Earth orbit, whereas JWST will operate 1.5 million miles out at the L2 point, using its huge solar shield to block sunlight and stay cool.
Image: Hubble and JWST’s primary mirrors compared. Credit: NASA/MSFC.
by Paul Gilster | Jan 30, 2007 | Deep Sky Astronomy & Telescopes |
It took six years to develop the design for the ‘microshutters’ that will fly aboard the James Webb Space Telescope. They will work with its near infrared spectrograph to screen out light from foreground objects. The advantages are enormous: The Webb telescope will be able to adjust its light mask with exquisite precision, something that previous technologies could not achieve to anywhere near this level of performance.
We’re talking thousands of tiny shutters — 62,000 to be exact — each measuring 100 by 200 microns, arranged in four identical grids. They’ll function in front of an eight million-pixel detector, allowing only the light from the specific areas under observation to reach the instrument.
Moreover, the new technology greatly widens the efficiency of the instrument in terms of observational time. Says Harvey Moseley (principal investigator for the microshutter at GSFC): “The microshutters provide a conduit for faint light to reach the telescope detectors with very little loss. The shutters allow us to perform spectroscopy on up to 100 targets simultaneously. We will be able to see deeper in less time.”
All this while functioning at a cool 40 Kelvin (-388 degrees Fahrenheit, or -233 degrees Celsius), the operational temperature for the Webb spectrograph. A nice NASA feature on how all this works is here. Webb doesn’t launch until 2013 at the earliest, and in addition to its deeper view into the early universe, it should have powerful capabilities at penetrating dust and debris to find planetary systems in formation.
The comparison between the Webb instrument and the Spitzer Space Telescope is striking. Consider this: Spitzer’s aperture diameter is roughly three feet. The Webb’s aperture will be closer to 20. More on its design in this interview MSNBC’s Alan Boyle conducted with John Mather (senior project scientist for the JWST). Having discussed ways of determining the chemical composition of the atmosphere of planets around other stars, Mather goes on to consider one system that merits special attention:
We also are pretty sure there’s a planet that could be detected directly, around a star called Fomalhaut. It’s got a big cloud of dust around it which is organized in a ring. This ring is probably due to the gravitational force of a planet that’s also orbiting that same star. So we think we know more or less where to look for that planet, and that it should be big enough to see directly. But it’s more like Jupiter than like Earth. So we’ll be getting some clues about the possibilities for life elsewhere from all of this.
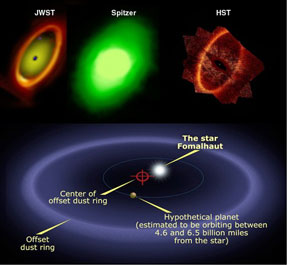
When you’re flying an instrument that can probe to the earliest era of the universe, working on exoplanets seems like staying close to home. But notwithstanding the science we’ll pull from the Webb telescope about the beginnings of the cosmos, the instrument’s effectiveness in studying planetary disks could help us with many puzzles, including hot issues like planetary migrations as gas giants move toward their parent star, and the effect of their passage on planets in the inner system. But with launch six years away, we’re bound to accumulate still more mysteries for the JWST to investigate around interesting places like Fomalhaut.
Image: JWST, Spitzer and HST views of the area around the star Fomalhaut, with diagram showing a suspected planet. Credit: NASA, GSFC.
by Paul Gilster | Jan 29, 2007 | Exoplanetary Science |
A globular cluster is a glorious thing. Consider Omega Centauri, a vast city of stars about 15,000 light years from Earth. Clusters like this one are composed of millions of Population II stars, meaning they’re among the oldest observed stars and may date back as far as twelve billion years. A result of their early formation is that they remain deficient in metals (in astronomical terms, the elements above hydrogen and helium), making them ideal laboratories for a particular branch of exoplanet studies.
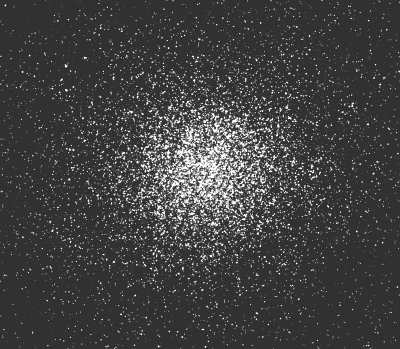
Image: This image of Omega Centauri, the brightest and largest globular cluster in the sky, was obtained with the Danish 1.5 m telescope at the ESO La Silla observatory. It shows the central part only; the cluster is actually much larger than the field reproduced here. Credit: European Southern Observatory.
A growing assumption about the massive ‘hot Jupiters’ we’ve found in our early planet hunting is that their existence depends upon a relatively high metallicity in their host star. The planets we’ve found certainly fit that bill, but is this just an observational bias, given that we’re looking at nearby stars that are all metal-rich to begin with? To test the idea, a team led by David T.F. Weldrake (Max-Planck Institut für Astronomie, Heidelberg) has been looking at Omega Centauri and 47 Tucanae, the two brightest clusters in the sky, to see if metal-poor stars produce such planets.
Working with Australian National University’s 1-meter telescope and Wide Field Imager, the team compiled 56 nights of data and gathered photometry on 53,000 main sequence stars, sampling the outer halos of each cluster. Analyzing the occurrence frequencies expected for gas giants in close orbits and factoring in the transit probabilities, the team expected to find 7 planets in 47 Tucanae and 5.3 in Omega Centauri. The actual result: not a single transit around any of the sampled cluster stars.
Note the method here. The work proceeded under the assumption that stellar metallicity does not affect the occurrence of these planets in globular clusters. If that were the case, the numbers expected should have been found. The result is statistically significant. From the paper:
…our null result suggests that stellar metallicity, not dynamics, is the dominant effect limiting the frequency of short period massive planets in globular clusters, and places an observational constraint on planetary frequency at such a low metallicity. Perhaps the low metallicity does not affect planet formation, but does affect planetary migration. If true, then long period planets should still exist in these clusters, undetectable in our work.
Centauri Dreams‘ take: The upshot is that we have to be careful in stretching our conclusions too far. What we can say is that the existence of metals in stellar atmospheres is implicated as the leading factor in preventing hot Jupiters from forming in these clusters. But the other great imponderable, how such planets migrate to the inner system, is still in play. Its possible role in planetary formation needs further study.
And because we’ve speculated in these pages about the view from a terrestrial world in a globular cluster, let’s add one more thing. While the planet/metallicity connection seems to be firming up rather well for gas giants, we know little about its effect on smaller, rocky worlds. It seems a natural assumption that these glorious cities of stars would be bereft of such planets, but the present work does not address that question.
The paper is Weldrake et al., “Searching for Planetary Transits in Globular Clusters – 47 Tucanae and ω Centauri,” slated to appear in the PASP proceedings of “Transiting Extrasolar Planets Workshop” (Heidelberg), and available as a preprint online.
by Paul Gilster | Jan 27, 2007 | Outer Solar System |
Centauri Dreams‘ view is that the more we can learn about asteroids, the better. And the interest isn’t purely scientific. One day we may have to set about nudging an approaching asteroid so as to prevent a collision with Earth, and if that day comes, we’ll need to have a plan in place that depends upon a thorough understanding of these objects and their composition. In the long run, asteroid studies are anything but optional.
The European Space Agency’s Rosetta mission is filling in some of the gaps enroute to comet 67P Churyumov-Gerasimenko. In its sights are two asteroids — 2867-Steins and 21-Lutetia — orbiting between Mars and Jupiter. We’ll learn a lot more about both between now and July of 2010, by which time Rosetta will have viewed each at close range.
Meanwhile, the spacecraft has taken a first look at 21-Lutetia using the onboard OSIRIS (Optical, Spectroscopic, and Infrared Remote Imaging System) instruments. At the time of its observations, the vehicle was roughly 245 million kilometers from the asteroid, which shows up as little more than a bright spot in the early imagery.
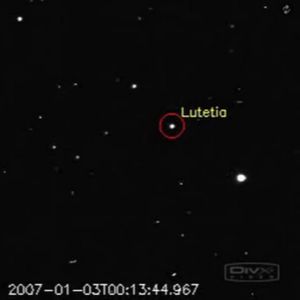
So what is Rosetta up to here? The early observations are intended to study the rotation of the 100-kilometer object, analyzing the changes in the intensity of light reaching the instrument. Up next for Rosetta: a swing past Mars that will slingshot the spacecraft toward Earth for yet another gravity assist this coming November. The ultimate objective when the comet is reached is to land a probe on the surface of 67P/Churyumov-Gerasimenko, followed by a two year study.
Image: Asteroid 21-Lutetia, as seen by the spacecraft from a distance of about 245 million kilometres, during a remote-sensing observation campaign of this object that lasted 36 hours. Click on the image to see ESA’s animation of the observing session (and note the occasional flashes, which are the result of cosmic rays hitting the OSIRIS detectors). Credit: ESA/MPS/UPD/LAM/IAA/RSSD/INTA/UPM/DASP/IDA.
by Paul Gilster | Jan 26, 2007 | Culture and Society, Exotic Physics |
Sometimes what we don’t know is more interesting than what we do. I’m always confounded when I hear people lay out confident scenarios for the human future, each different from the next, when we’re still at a stage where we don’t even know what the universe is made of. While we’re figuring out dark matter and (even worse) dark energy, we can answer some of the other big questions looked at in this article in Wired. What happens to information in a black hole? What causes gravity? How do entangled particles communicate? Some significant names tackle these questions — not all cosmological by any means — in entertaining form.