by Paul Gilster | Aug 20, 2020 | Outer Solar System |
Have a look at what two of our older spacecraft saw on Jupiter’s giant moon Ganymede, a world that will snap into much greater focus once the JUICE mission arrives in 2029. The JUpiter ICy moons Explorer is slated for launch in 2022, with the intention of studying Ganymede, Callisto and Europa. But it’s Ganymede that will get the lion’s share of the attention from this European Space Agency effort as JUICE slides into orbit around the moon in 2032, marking the first time a spacecraft has orbited a moon circling a planet other than our own.
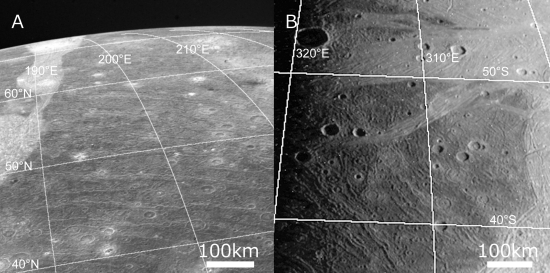
Image: Images of Ganymede’s surface taken by Voyager 2 (left) and Galileo (right). The Dark Terrain and Bright Terrain areas can be recognized, with concurrent furrows present in the Dark Terrains. Credit: NASA.
We looked at more recent Juno views of Ganymede just last month (see Glimpses of Ganymede), along with interesting results from its most recent flyby on disruptions in the crystalline structure of the moon’s north polar ice due to incoming plasma. Now we return to Jupiter space because of a new paper from Naoyuki Hirata (Kobe University) and colleagues that examines the orientation and distribution of tectonic troughs on Ganymede’s surface. Their concentric arrangement implies what may be the largest impact structure identified in the Solar System. The data used in the work come from both Voyagers and the Galileo spacecraft.
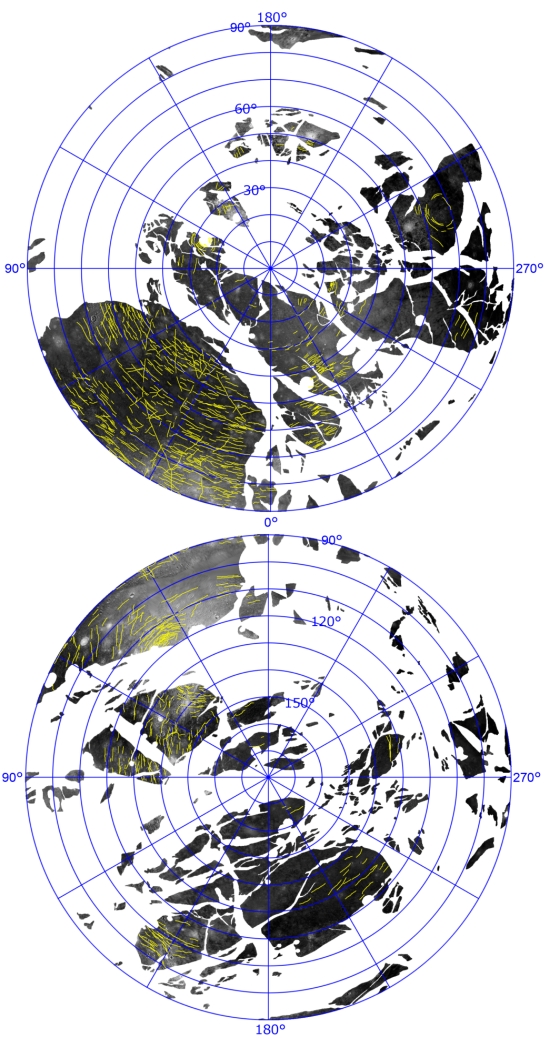
Image: Azimuthal equidistant map centered at 20° south 180° west showing Ganymede’s Dark Terrain and furrows (indicated by yellow lines). (Bottom) Azimuthal equidistant map of Ganymede’s surface centered at 20° north and 0° west. This shows the opposite hemisphere of Ganymede from the top image. The white areas indicate Bright Terrain. Credit: NASA.
Hirata’s team used computer simulations at the National Astronomical Observatory of Japan (NAOJ), speculating that what we are looking at is the result of an asteroid impact, a mighty blow struck by an object significantly greater than 50 kilometers in radius, and perhaps as much as 150 kilometers in radius, moving at 20 kilometers per second.
Almost all the furrows found in the spacecraft imagery are concentric rings aligned around a single point centered at 20°S 180°W, with the radial extent of the structures measuring 7800 kilometers. Bear in mind that the mean circumference of Ganymede is only 16,530 kilometers. If we really are seeing a crater here, it dwarfs Callisto’s 1900 kilometer crater, previously thought the largest in our system.
A 150 kilometer impactor would produce a melt pool with a radius of 1000 kilometers, a possible explanation for the central region of Ganymede’s Marius Regio, which is free of furrows. Usefully, the Hirata simulations lead to a prediction that we will soon be able to test. From the paper:
Our numerical impact simulation for the case of Rimp = 150 km (Rimp
=50 km) demonstrates the generation of a large melt region with a radius of ~1000 km (~500 km). This large melt region would allow the dense material to gravitationally segregate, which generate positive gravity anomaly such as mass concentrations seen on the lunar impact basins. Therefore, we predict that, if our view is correct, positive gravity anomaly with a radius of ~1000 km when Rimp = 150 km (or a radius of ~500 km when Rimp = 50 km) should exist around 20°S 180°W. This can be tested by future geodetic observations of Ganymede such as the JUICE (Jupiter Icy moon Explorer) or Europa Clipper mission.
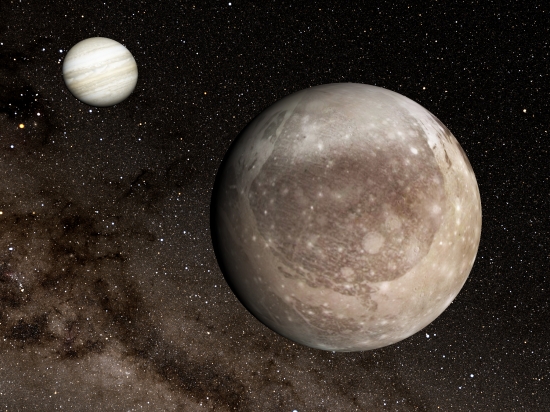
Image: Jupiter (background, left) and its moon Ganymede (foreground, right) visualized using the Four-Dimensional Digital Universe viewer “Mitaka.” The furrows found on the older, Dark Terrain, areas of Ganymede’s surface could actually be parts of a single, giant, multiring impact crater. Credit: Tsunehiko Kato, 4D2U Project, NAOJ.
The paper is Hirata et al.,”A global system of furrows on Ganymede indicative of their creation in a single impact event,” Icarus Vol. 352 (July 15, 2020), 113941 (abstract).
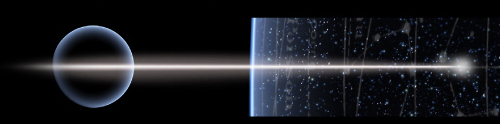
by Paul Gilster | Aug 19, 2020 | Exoplanetary Science |
The boundary between planet and star is hard enough to pin down without thinking of some recently discovered brown dwarfs that are cool enough to approach Earth temperatures. Yet worlds/stars like these are among the haul assembled by volunteers working data for Backyard Worlds: Planet 9, a citizen science project whose latest findings include 95 cool brown dwarfs in the Sun’s neighborhood, as reported in the Astrophysical Journal.
Despite a determined search, we’ve yet to find such an object closer than the nearest stars at Alpha Centauri. But 23 light years out — the distance of the closest of these brown dwarfs — is definitely close in galactic terms, and most of the brown dwarfs tracked in the new work are between 30 and 60 light years from Earth. That makes sense, for objects like these are faint enough that identifying them at greater range is all but impossible.
The data used in the brown dwarf collection come from a range of observatories including W. M. Keck, Mont Mégantic, Las Campanas, Kitt Peak and Cerro Tololo. Space-based data from WISE (Wide-field Infrared Survey Explorer) were also valuable, as were follow-up observations from the Spitzer Space Telescope providing photometric confirmation. Low temperature brown dwarfs like these build our catalog while also clarifying gaps in the low-temperature population.
Jackie Faherty (American Museum of Natural History) is a co-author of the paper, whose lead author is Aaron Meisner (NSF NOIRLab). Faherty places the work in context, while giving a nod to the Backyard Worlds participants:
“This paper is evidence that the solar neighborhood is still uncharted territory and citizen scientists are excellent astronomical cartographers. Mapping the coldest brown dwarfs down to the lowest masses gives us key insights into the low-mass star formation process while providing a target list for detailed studies of the atmospheres of Jupiter analogs.”
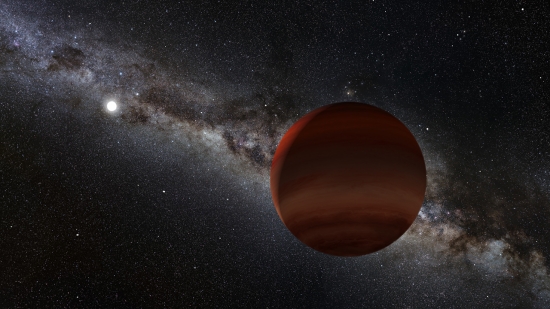
Image: Artist’s impression of one of this study’s superlative discoveries, the oldest known wide-separation white dwarf plus cold brown dwarf pair. The small white orb represents the white dwarf (the remnant of a long-dead Sun-like star), while the brown/orange foreground object is the newly discovered brown dwarf companion. This faint brown dwarf was previously overlooked until it was spotted by citizen scientists, because it lies right within the plane of the Milky Way. Credit: NOIRLab/NSF/AURA/P. Marenfeld. Acknowledgement: William Pendrill.
We can also thank NOIRlab’s Community Science and Data Center (CSDC), which made the large survey sets available through its Astro Data Lab science platform. The web portal makes matching data sets from a variety of observatories available to citizen scientists. Meisner adds:
“The technical burden of downloading billion-object astronomical catalogs is typically insurmountable for individual investigators—including most professional astronomers. Thankfully, the Astro Data Lab’s open and accessible web portal allowed Backyard Worlds citizen scientists to easily query massive catalogs for brown dwarf candidates.”
The authors make the point that some of the objects in this study will be potential targets for follow-up spectroscopy by the James Webb Space Telescope:
Among our most exciting discoveries are new candidate members of the 10 pc sample, two objects moving faster than 200″/yr, three T type subdwarf candidates, five Y dwarf candidates, and a new T8 plus white dwarf co-moving system. Our Y dwarf candidates begin bridging the gap between the bulk of the Y dwarf population and the coldest known brown dwarf, making them potential targets for JWST spectroscopy. Backyard Worlds is actively pursuing additional ground-based follow-up of the discoveries presented in this study, especially spectroscopy where feasible. While this work’s new brown dwarf candidates already demonstrate the power of citizen science for mapping the solar neighborhood, these objects make up only a small fraction of Backyard Worlds moving object discoveries to date. As NEOWISE continues scanning the sky, Backyard Worlds will endeavor to search all of its newly delivered data for yet more cold and close neighbors to the Sun.
Backyard Worlds volunteers have discovered more than 1,500 stars and brown dwarfs near the Sun. The current batch represents some of the coldest brown dwarfs in that collection. More than 100,000 citizen scientists are involved in the project, a network that examines telescope images to identify the movements of nearby dwarfs and planets. Putting human eyes on large data sets is integral to the search for rare objects, an approach astronomers will exploit with data gathered by the Vera C. Rubin Observatory (VRO). Now under construction in the Chilean Andes, the instrument will survey the southern hemisphere sky every three nights over a ten year period.
The paper is Meisner et al., “Spitzer Follow-up of Extremely Cold Brown Dwarfs Discovered by the Backyard Worlds: Planet 9 Citizen Science Project,” accepted at the Astrophysical Journal (preprint).
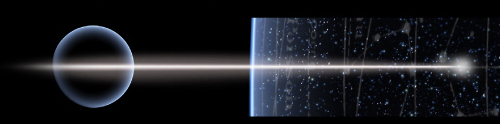
by Paul Gilster | Aug 18, 2020 | Interstellar Medium |
Studies of interstellar interloper ‘Oumuamua move at lightning pace, to judge from a recent exchange on hydrogen ice. A study by Greg Laughlin and Darryl Seligman (both at Yale) just published in June, has now met a response from Thiem Hoang (Korea University of Science and Technology, Daejeon) and Harvard’s Avi Loeb. The issue is significant because if, as Laughlin and Seligman argued, ‘Oumuamua were made of hydrogen ice, then the outgassing that drove its slight acceleration would not have been detectable. At least one mystery solved.
Or was it? One reason the 0.2km radius object didn’t fit the description of a comet was that there was no explanation for its tiny change in velocity. Hoang and Loeb have examined the hydrogen ice concept and found it wanting. Says Hoang:
“The proposal by Seligman and Laughlin appeared promising because it might explain the extreme elongated shape of ‘Oumuamua as well as the non-gravitational acceleration. However, their theory is based on an assumption that H2 ice could form in dense molecular clouds. If this is true, H2 ice objects could be abundant in the universe, and thus would have far-reaching implications. H2 ice was also proposed to explain dark matter, a mystery of modern astrophysics.”
Which sounds interesting in itself because anything bearing on dark matter is worth a look, given our frustration at understanding the matter behind the hypothesis. Laughlin and Seligman had suggested a giant molecular cloud [GMC] as the origin for ‘Oumuamua, but Hoang and Loeb argue that the earlier paper, while considering the destruction of H2 ice in the interstellar medium through evaporation, did not take into account the improbability of such ices forming within a GMC, or the effects of that environment upon their later growth. From the paper:
Assuming that H2 objects could be formed in GMCs by some mechanisms (Füglistaler & Pfenniger 2016; Füglistaler & Pfenniger 2018; Seligman & Laughlin 2020), we quantify their destruction and determine the minimum size of an H2 object that can reach the solar system. We assume that the H2 objects are ejected from GMCs into the ISM by some dynamical mechanism such as tidal disruption of bigger objects or collisions (see Raymond et al. 2018; Rice & Laughlin 2019).
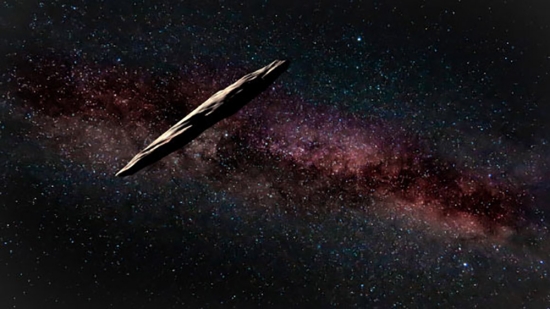
Image: An artist’s rendering of ‘Oumuamua, a visitor from outside the Solar System. Credit: The international Gemini Observatory/NOIRLab/NSF/AURA artwork by J. Pollard.
Hoang and Loeb’s calculations show that H2 icebergs are unlikely to grow to large size because of collisional heating not just from dust but from gas within the birth cloud, meaning that ‘Oumuamua likely wasn’t a hydrogen iceberg (and, along the way, taking out the ancillary proposition that dark matter may be accounted for by H2 snowballs). Micron sized grains in regions where the density of gas is high will cause the hydrogen on the grains to sublimate.
Assuming that H2 objects could somehow form in the densest regions of GMCs, we found that sublimation by collisional heating inside the GMC would destroy the objects before their escape into the ISM [interstellar medium]. We also studied various destruction mechanisms of H2 ice in the ISM. In particular, we found that H2 objects are heated by the average interstellar radiation, so that they cannot survive beyond a sublimation time of tsub ~ 10 Myr for R = 300 m (see Figure 1). Only H2 objects larger than 5 km could survive.
While giant molecular clouds like (GMC) W51, one of the closest to Earth at roughly 17,000 light years, could be a point of origin for the object, the authors argue that even this close GMC is simply too far away. Moreover, it might be hard for a hydrogen iceberg even to exit the giant molecular cloud in the first place. Collisional heating within a GMC would destroy objects like this through thermal sublimation long before they reached a distant stellar system. The paper finds that objects below 200 meters in radius would be destroyed within the parent GMC.
We also have to find a way to get ‘Oumuamua all the way from its birthplace to our Solar System. What Hoang and Loeb point out is that an iceberg made of hydrogen would be unlikely to survive an interstellar journey that would probably take hundreds of millions of years. An object like this is going to begin to evaporate. Their paper goes to work out the survivability of H2 ice from interstellar radiation given thermal sublimation and photodesorption along the way.
Many other factors come into play that cause problems for a hydrogen iceberg. It has to stand up to cosmic rays as well as impacts with matter in the interstellar medium. And we have to throw in what can happen when an object like ‘Oumuamua enters the Solar System, where solar radiation becomes an issue. The calculations presented here show the significance of thermal sublimation due to starlight, while going beyond this to reveal the effects of cosmic rays and impacts with interstellar matter, which turn out to be less significant.
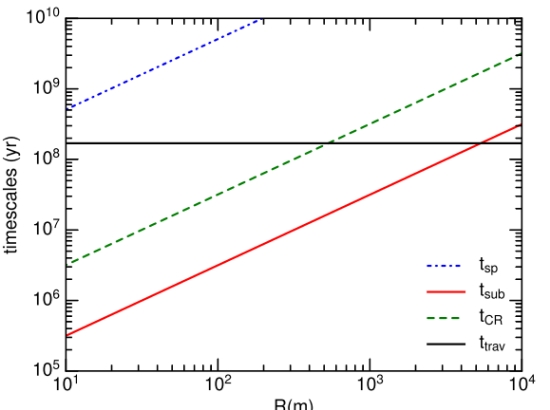
Image: This is Figure 1 from the paper. Caption: Comparison of various destruction timescales (slanted colored lines) as a function of the object radius (in meters) to the travel time from a GMC at a distance of 5.2 kpc, assuming a characteristic speed of 30 km s?1 (horizontal black line). Credit: Hoang & Loeb.
The authors calculate a minimum radius of H2 objects in the range of 5 kilometers for survival in a journey that would have to take in formation in giant molecular clouds and movement through the interstellar medium. 10 million years would wreak havoc on an object the size of ‘Oumuamua.
We’re in a period of energetic debate, a time when the unresolved questions about ‘Oumuamua remain in play. It seems clear that we need a larger population of interstellar objects to place the current work in context, and Loeb has pointed out that we won’t have long to wait:
“If ‘Oumuamua is a member of a population of similar objects on random trajectories, then the Vera C. Rubin Observatory (VRO), which is scheduled to have its first light next year, should detect roughly one ‘Oumuamua-like object per month. We will all wait with anticipation to see what it will find.”
The paper is Hoang & Loeb, “Destruction of Molecular Hydrogen Ice and Implications for 1I/2017 U1 (‘Oumuamua),” Astrophysical Journal Letters Vol. 899, No. 2 (17 August 2020). (Abstract). The Seligman & Laughlin study that argued for “‘Oumuamua as a hydrogen iceberg is “Evidence that 1I/2017 U1 (‘Oumuamua) was Composed of Molecular Hydrogen Ice,” Astrophysical Journal Letters Vol. 896, No. 1 (9 June 2020). Abstract.
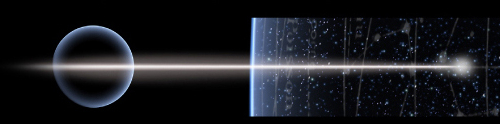
by Paul Gilster | Aug 14, 2020 | Sail Concepts |
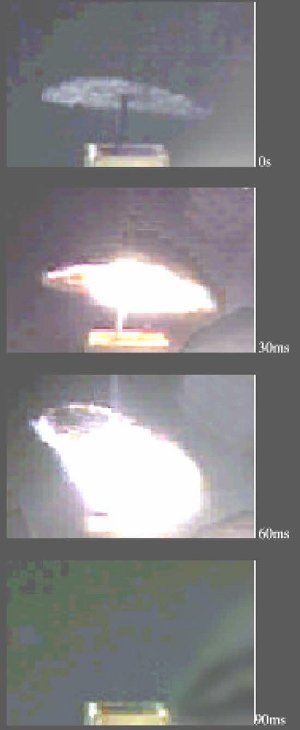
The first laboratory work on pushing a space sail with microwaves was performed by Jim and Greg Benford at the Jet Propulsion Laboratory back in 1999, with the results presented the following year at a European conference. Leik Myrabo (then at Rensselaer Polytechnic Institute) was, at about the same time, performing experiments with lasers at Wright-Patterson Air Force Base in Ohio. When you think about the problems of laboratory work on these matters, consider the fact of gravity, meaning that you are working in a 1 g gravity well with diaphanous materials whose acceleration depends on how hot you can allow them to become.
Advances in materials and in particular in lightweight carbon structures allowed the Benfords’ experiments to succeed, with the help of a 10-kilowatt microwave beam that produced significant acceleration on the test object. But I’m reminded by looking at a new paper on sail technologies using no beam at all that the Benfords also demonstrated something else. Molecules of CO2, hydrocarbons and hydrogen that had been incorporated in the lattice of their material at manufacture emerged under the high temperatures involved. Thus another form of acceleration came into play through the phenomenon called desorption.
Image: Carbon disk sail lifting off of truncated rectangular waveguide under 10 kW microwave power (four frames, 30 ms interval, first at top). Credit: James and Gregory Benford.
Just how useful an effect may desorption turn out to be? It seems to offer an acceleration dividend. Roman Kezerashvili (City University of New York), working with colleagues at Samara National Research University in Russia and the State University of New York at Buffalo, has now analyzed the effect of desorption on an inflated, torus-shaped sail. I had the pleasure of talking with Dr. Kezerashvili in Italy at the Aosta conference in 2009, where he discussed relativistic effects that have to be taken into account in the navigation of a space sail close to the Sun. The current work follows up an earlier paper on desorption he presented in 2016.
We have the prospect of incorporating compounds into a sail that become a kind of propulsive shell that is triggered either by microwave beam or, in the case of a close Solar pass, by the Sun itself. Think of desorption as involving a kind of propulsive ‘paint.’ In terms of beaming, it is necessary to understand that desorption can take place under either microwave or laser beam, but microwaves do not damage sail materials and so heat them far less destructively. The Benfords’ work, as Kezerashvili points out, shows that microwave beaming produces more efficient absorption in the sail’s coating materials, and high specific impulse can result from desorption.
In fact, in the Benford experiments on ultra-light carbon sails, photon pressure can account for no more than 30 percent of the observed acceleration. The rest comes from desorption.
Various materials can undergo desorption at different temperatures — the sail analyzed by Kezerashvili in 2016 uses carbon, whose properties of acceleration are there analyzed. If low mass atoms or molecules can be blown out of the sail lattice at known temperatures, the mission concept Kezerashvili suggested in that 2016 paper can emerge, one in which the sail reaches desorption temperature at a particular point in a close pass around the Sun. In this scenario, no microwave or laser beam is needed:
It is of particular interest to consider an inflatable torus-shaped solar sail as both propellant-less and propellant-based system. It is a propellant-based and a propellant-less system which create thrust by the sun-driven ejection of a flux of particles of non-zero rest mass due to the desorption of coating and solar radiation pressure, while it performs as a propellant-less conventional solar sail after the thermal desorption ends.
As far as I know, this new paper is the first analysis of desorption on an inflatable sail, but inflatable structures have a long history due to their flexibility and low volume at launch. Among the earliest inflatables to be used in space were the Echo balloons launched in the 1960s, but inflatable structures have been developed extensively in the decades since. I’ve written in the past on a hydrogen-inflated sail using a molybdenum reflector that became the basis for an early orbiting radio telescope design, a concept Greg Matloff extended and investigated along with Kezerashvili and Italian physicist Giancarlo Genta for interstellar flight purposes.
This is also the first paper that considers thermal desorption in the context of solar heating alone, which leads to an interesting class of ‘sundiver’ missions with close solar passes. Thus a conventional solar sail coated with desorptive paint is heated by the space environment.
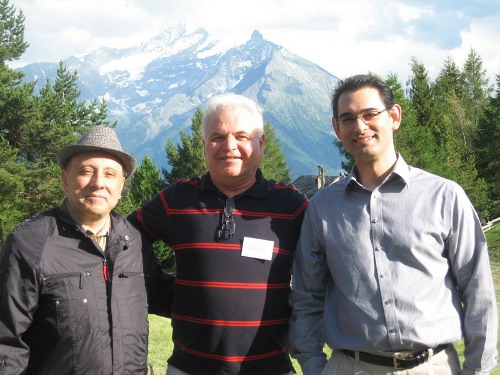
Image: From the Aosta conference of 2009, a fine memory: A snapshot taken in the Italian Alps. Left to right: Giovanni Vulpetti, Roman Kezerashvili, and Justin Vazquez-Poritz.
On deployment matters: Most solar and beamed sails discussed in the literature assume systems of electromagnetic actuation devices, often involving guide rollers and booms. All of this, of course, adds to the mass of the resulting structure as well as its complexity. Deployment through a series of inflatable booms, as assumed in the inflatable sail concept, simplifies an otherwise intricate procedure. The paper also mentions the advances in flexible polymers and high-strength fibers that allow more mass packed into the launch vehicle at lower cost.
So inflatable sails make sense. The new Kezerashvili paper looks at the dynamics of an inflatable sail, conceived as “a thin reflective membrane attached to an inflatable torus-shaped rim.” The round, flat membrane is coated with heat sensitive materials that make the transition from solid state to gas. Pressure introduced into the rim allows sail deployment from the initial stowed configuration, with deployment occurring at the chosen heliocentric distance.
At this point the sail membrane is extended into its final flat shape, with thermal desorption occurring at a specific temperature. For the purposes of these calculations, the authors cite an acceleration time for the torus-shaped solar sail due to thermal desorption of about 1500 seconds, with the mass of the coating material pegged at 1.5 kg and a desorption rate of 1 g/s.
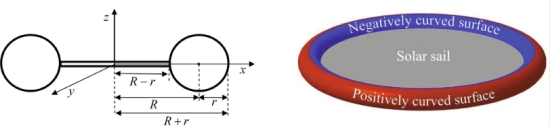
Image: This is Figure 1 from the paper. Credit: Kezerashvili et al.
And here is a figure from one of Dr. Kezerashvili’s presentations on the inflated sail.
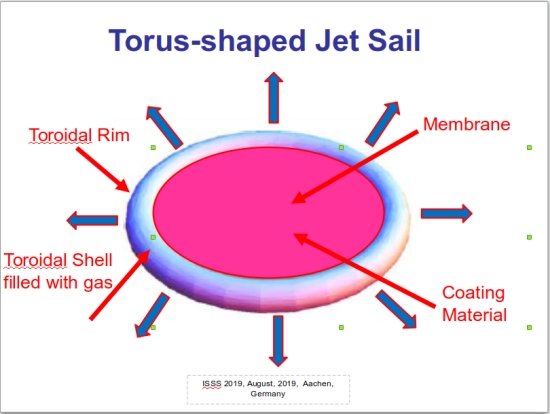
Thus we wind up with two types of acceleration at perihelion, the first being the expected solar radiation pressure, the second being that caused by thermal desorption from the sail itself. The authors assume a beryllium-coated solar sail and analyze the membrane mass of the sail as well as the toroidal rim and coating material, along with the desorption rate, under varying molecular hydrogen gas fills and a temperature range varying with the perihelion approach considered. The different gas fills change the tensile stress on the sail membrane. The paper analyzes the structural strength required in the inflatable torus to support the circular membrane of the sail and considers the deflection of the membrane due to acceleration.
A ‘sundiver’ maneuver is a mission scenario we’ve discussed often in these pages — only this one occurs with an inflatable sail, and it is a mission in which the sail is deployed just as the appropriate temperature is reached. The additional kick provided by desorption produces a substantial boost in performance over a sail driven by solar radiation alone:
The present study reveals that the inflation deployed torus-shaped solar sail accelerated via thermal desorption of coating results in high post-perihelion heliocentric solar sail velocities. With the speed 20-40 AU/year, post-perihelion travel times to the vicinity of Kuiper Belt Objects (KBO) will be less than 1-3 years, while the Sun’s gravity focus at 547 AU can be reached in 13-25 years.
What interested me in particular was the idea of extending the concept into small sails and opting for a ‘fleet’ concept:
The suggested configuration of the torus-shaped solar sail fits the cube-scale size configurations. Recent research reveals that much smaller sails could be incorporated with highly miniaturized chip-scale spacecraft. It is quite possible that a single dedicated interplanetary ”bus” could deploy many cube-scale sails at perihelion. Sequential deployment of a fleet of solar sails could be timed to allow exploration of many small KBOs from a single launch. The natural continuation of this work can be extended in the following directions: i. Detailed research on materials for thermal desorption at temperature suitable for solar sailing; ii. consideration of the Sun as an extended source of radiation; iii. study the influence of solar sail surface oscillations on the motion of a spacecraft performing an interplanetary flight.
Interesting issues arise with a sail like this. The coating on the surface of the sail must be uniform, and in fact one would expect that slight variations will occur that can lead to trajectory deviations during the desorption process. Remember that the time of desorption acceleration is small, around 1500 seconds, in the configuration discussed here. The authors assume there would be a need for periodic corrections in the sail’s tilting angle as these deviations occur. You can also see how neat a fit this work makes with Kezerashvili’s earlier studies on navigation issues deep in a gravity well. An error in relativistic calculations close to the Sun could lead to substantial variations in the final trajectory.
The paper is Kezerashvili et al., “A torus-shaped solar sail accelerated via thermal desorption of coating,” in press at Advances in Space Research (abstract). The original 2016 paper is Ancona and Kezerashvili, “Orbital dynamics of a solar sail accelerated by thermal desorption of coatings,” Proceedings of 67th International Astronautical Congress (IAC 2016), Guadalajara, Mexico, 26-30 September 2016. Paper IAC-16-C1.6.7.32480 (preprint). The comprehensive paper on extrasolar exploration by a solar sail accelerated via thermal desorption of a coating that grew out of this is published in Advances in Space Research 63, pp. 2021-2034 (2019).
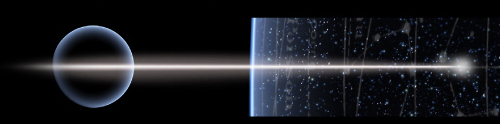
by Paul Gilster | Aug 13, 2020 | Outer Solar System |
A great part of the excitement of scientific discovery is not knowing what will emerge when you take data. Our space missions have proven that time and again, and I have no doubt that as we tighten the resolution on future telescopes, we’ll find things that defy many an accepted theory. NASA’s Stardust mission reflects the phenomenon. Designed as a comet sample return, Stardust is now providing information about the migration of materials in the primordial Solar System, which may point toward a phenomenon more widespread than earlier believed.
Thus the work of Devin Schrader and Jemma Davidson (University of Arizona Center for Meteorite Studies). Working with colleagues at the Smithsonian Institution’s National Museum of Natural History, the University of Hawai?i at M?noa, Washington University in St. Louis, and Harvard University, the duo have produced evidence that at least fragmentary materials in the inner Solar System crossed what is often called the ‘Jupiter Gap’ and moved much further from the Sun. The Stardust samples produced evidence for the kind of migration that the authors then followed up by analyzing numerous samples of early chondrite meteorites.
Says Schrader:
“This research provides new information about the dynamics of the early Solar System. Our research shows that these two reservoirs were not completely isolated from one another.”
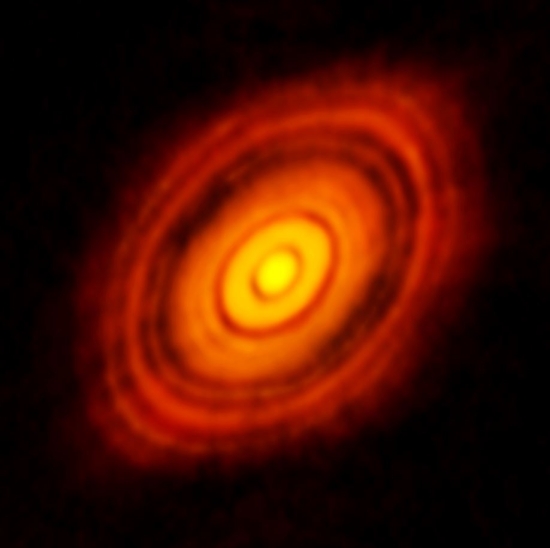
Image: Atacama Large Millimeter Array image of the protoplanetary disk around HL Tauri. The dark rings are gaps in the dust and gas-rich protoplanetary disk, likely due to the formation of planets. These gaps may be similar to the disk gap thought to be formed by the formation of Jupiter in our protoplanetary disk. Credit: ESO/ALMA.
The so-called Jupiter Gap should be examined within the context of current theories of gas giant formation. A gas giant core of between 5 and 10 Earth masses likely formed about 1 million years into the growth of the new system, soon drawing in a dense atmospheric envelope. The gravitational influence of this world would be sufficient to create a gap in the disk of gas and dust around the Sun out of which the planets we see today emerged. The idea that material might somehow cross this divide to move from one disk reservoir to another seemed unlikely.
But the matter demanded exploration because the degree to which such mixing occurs in protoplanetary disks is unresolved, and the nature of the chemical and physical structure of the resulting system depends on it. It’s a complicated analysis, one in which scientists must plug in the conservation of angular momentum as they study the migration of tiny particles, the role played by the pressure of the gases involved and the drag within them, and the effects of whatever planetesimals and larger objects begin to emerge from the mix.
The idea of a Jupiter Gap emerged out of modeling of these factors in various studies in the literature, and it combines with analyses of the calcium- and aluminum-rich inclusions in chondrites, with the prediction that while smaller particles (less than 300 ?m) could pass through the gap, larger particles would be blocked. And thus we would have differences in the isotopes found in meteorites formed in the inner system (non-carbonaceous) and those that formed in the outer system (carbonaceous chondrites) up to 4 million years after the system’s birth.
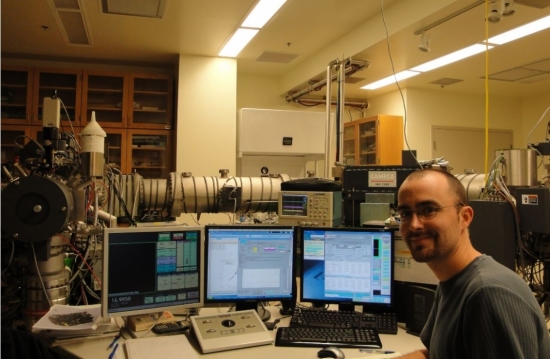
Image: Associate Research Professor Devin Schrader uses the ion mass spectrometer at the University of Hawai?i at M?noa to determine the isotopic composition of individual minerals in meteorites. Photo credit: Devin Schrader/ASU.
Samples of both are fortunately abundant at the various institutions involved in Schrader and Davidson’s work. The scientists deployed electron probe microanalyzers for imagery and analysis of individual minerals and a secondary ion mass spectrometer to analyze the isotopic composition of the samples. The round grains known as chondrules found in chondrites as part of their earliest formation, particularly those that were only partially melted during formation (‘relict grains’) provide evidence of chondrite formation and early transport within the disk.
The population of chondrites is fortunately varied enough to make fine distinctions among them by measuring the chemical and oxygen isotope compositions of relict grains in their chondrules, allowing the authors to differentiate between their origins and discuss disk transport within the first 4 million years of the Solar System’s formation. The mixing between inner and outer Solar System, occurring despite the Jupiter Gap, seems to have been complex and widespread. It will be interesting to see what analysis of asteroid samples from Hayabusa2 and OSIRIS-REx, both expected within the next several years, may yield in support or refutation of this theory.
The paper is Schrader et al., “Outward migration of chondrule fragments in the early Solar System: O-isotopic evidence for rocky material crossing the Jupiter Gap?” Geochimica et Cosmochimica Acta Volume 282 (1 August 2020), pp. 133-155 (abstract).
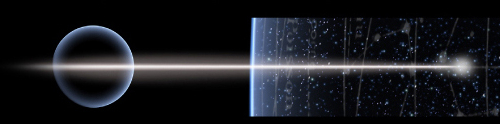