by Paul Gilster | Sep 29, 2007 | Culture and Society |
Franklin Chang-Diaz, astronaut and CEO of Ad Astra Rocket Company, intends to test the VX-200 VASIMIR prototype in January. VASIMIR (Variable Specific Impulse Magnetoplasma Rocket) offers much greater fuel efficiency than conventional chemical rockets, working with hot plasma heated by radio waves and controlled by a magnetic field. Technology Review talks to Chang-Diaz about the prototype and the flight version to follow in this interview.
And here’s where Chang-Diaz see us going in the long-term:
I think lots of people are going to be moving into space. I think we will be populating the moon, building enclaves of research and even money-making ventures there. Just last month, Ad Astra signed an agreement with Excalibur Exploration Ltd., a British company, to mine asteroids [when the time is right]. I believe there will be a huge demand for resources, particularly water, from asteroids and comets, because taking water from the earth is going to be very expensive. We’re probably going to supply the moon and the habitat on the moon with water from comets.
Thus Earth as a place humans come back to; think of it as a kind of national park. Chang-Diaz rightly worries about redundancy in case something happens to our planet. Coupling the commercial space sector with next generation propulsion technologies may expand our options.
——-
And speaking of potential catastrophes, consider the work of Stephen Petranek, who argues that there are plausible ways to surmount almost any disastrous scenario you can mention. Is this the first time in history that humans have had a shot at controlling many of the greatest threats to their survival? View his absorbing presentation on ten ways the Earth could end at the annual TED (Technology, Entertainment and Design) Conference here. A former editor-in-chief at Discover, Petranek is now working on a book on the dangers of climate change.
——-
What’s the best way to explore an asteroid? If the object’s diameter is something less than eight kilometers, a bad step could put an exploring astronaut into orbit or worse. Even much larger objects present the problem of trying to maneuver on a surface without bouncing yourself into completely new trajectories. A possible solution is a tether out of MIT that would be unwound from a spool (deployed by remote-controlled rocket) to completely encircle the asteroid, establishing a roped path along which crews could work. A homely idea, to be sure, but a first step toward making on-site human exploration of Earth-crossing objects possible.
by Paul Gilster | Sep 28, 2007 | Missions |
The launch of the Dawn mission to the asteroids makes me think about solar sails. I realize that Dawn uses ion propulsion, about which more in a moment, but watching ion methods as they mature makes an emphatic point: We need to bring solar sail technologies up to the same readiness level that ion propulsion currently enjoys. And we need to be shaking out sail ideas in space. The Russian Znamya attempts at a ‘space mirror’ were attached to a Progress supply ship, and interesting mostly in terms of their deployment problems, leaving the 2004 Japanese test of reflective sails in space as the only free-flying experiments I know about.
Which is not to say I’m a skeptic about ion propulsion. It will be fascinating to follow the performance of Dawn’s engines as the mission progresses. 54 feet of solar array produce the needed power to ionize their onboard xenon gas, which is four times heavier than air. The ions are then electrically acccelerated and emitted as exhaust from the spacecraft. The result is an engine of great utility over time, as JPL’s Keyur Patel notes:
“Each of our three ion engines weighs in at 20 pounds and is about the size of a basketball. From such a little engine you can get this blue beam of rocket exhaust that shoots out at 89,000 miles per hour. The fuel efficiency of an ion engine is an order of a magnitude higher than chemical rockets and can reduce the mass of fuel onboard a spacecraft up to 90 percent. It is a remarkable system.”
Remarkable indeed. Dawn’s engines will accumulate 2,000 days of operation during the course of its eight-year investigations, pushing the vehicle with about the same amount of thrust as the weight of a piece of paper in your hand. Days and months of thrusting add up, giving the vehicle an effective change in speed of about 37,000 kph by the end of its mission.
And ponder this. A chemical rocket’s exhaust speed is limited by thermal issues — the rocket nozzle has to be able to bear up to the temperatures involved. The ion thruster’s top exhaust speed is limited instead by applied voltage. Ion propulsion’s future seems bright — the greater the exhaust speed, the more efficient the engine. Dawn needed a boost from a Delta II Heavy to get off the Earth’s surface, but once in space the long push for the asteroids is remarkably stingy on fuel, delivering ten times as much thrust per kilogram as chemical rockets. No wonder JPL has taken to calling Dawn the Prius of space. Ion engines are ingenious and space-tested devices (Deep Space 1 was an early proof of concept, and the SMART-1 lunar mission again validated the basic ion design).
But if something about an ion engine’s gentle push rings a bell, you’re circling, like me, back to the similar situation with solar sails. The momentum imparted by photons falling on a solar sail delivers a vanishingly small push. A sail one-third of a mile square in Earth orbit would be pushed to no more than ten miles per hour in its first hour of operation. The solar flux at that distance is nine orders of magnitude weaker than the force of the wind on the Earth’s surface, so you can see the reason why.
Like ion engines, the speed of solar sails can increase bit by bit, day by day, and unlike them, sails carry no propellant whatsoever. As long as sunlight is plentiful (out to about 5 AU), sails should make an efficient alternative to ion engines, particularly in explorations of the asteroids. And while the continued development of ion propulsion is heartening, it shouldn’t be forgotten that sail strategies continue to be carefully studied. We need to find budgetary help for the work that will lead to the deployment of demonstrator missions. A sail in space will teach us much more about this potentially paradigm-changing form of propulsion.
by Paul Gilster | Sep 27, 2007 | Deep Sky Astronomy & Telescopes |
An odd radio burst that seems to signal a previously undiscovered astrophysical phenomenon is now on the scene. Culled out of archival data gathered from the Parkes radio telescope in Australia, the burst may signal something exotic indeed, the last stages of the evaporation of a black hole. Another candidate: A collision between two neutron stars.
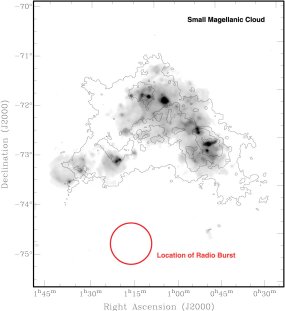
And while the data in question come from a survey that included 480 hours of observation of the Magellanic Clouds, some 200,000 light years from Earth, the phenomenon they’ve uncovered is far more distant. Drawing the attention of astronomers was the fact that no radio burst yet found shows the same characteristics. Despite its strength, the signal lasted less than five milliseconds. Dispersion effects caused by its passage through ionized gas in deep space caused higher frequencies to arrive at the telescope before lower frequencies.
Image: Visible-light (negative greyscale) and radio (contours) image of Small Magellanic Cloud and area where burst originated. CREDIT: Lorimer et al., NRAO/AUI/NSF.
Dispersion, it turns out, is a useful indicator, in this case helping to determine the distance of the event from Earth, about three billion light years. Maura McLaughlin (West Virginia University and NRAO) notes another potential benefit of unlocking the signal’s secret:
“We’re actively looking for more of these powerful, short bursts, in other archival pulsar surveys, and hope to resolve the mystery of their origin. In addition, if we can associate these events with galaxies of known distance, the radio dispersion we measure can be used as a powerful new way to determine the amount of material in intergalactic space.”
It’s an intriguing find, enough so that Matthew Bailes (Swinburne University, Australia) could say, “This burst represents an entirely new astronomical phenomenon.” Needless to say, follow-up studies are called for. They’ll involve a search for short, powerful bursts like this one in other archival survey data. Beyond that, the next generation of radio telescopes, more sensitive than today’s equipment, should be able to detect similar phenomena.
The paper is Lorimer, Bailes et al., “A Bright Millisecond Radio Burst of Extragalactic Origin,” ScienceXpress September 27, 2007 (abstract).
by Paul Gilster | Sep 27, 2007 | Missions |
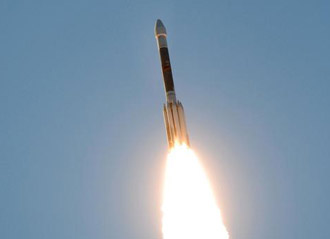
Great to see Dawn on its way. The spacecraft lifted off at 11:34 UTC, with signal acquisition just over one hour into the flight. The spacecraft will begin its exploration of Vesta in 2011 and Ceres in 2015, two asteroids that between them have much to tell us about the history of the Solar System. Measurements of shape, surface topography, tectonic history, elemental and mineral composition will be included in a full data acquisition package. Image credit: NASA.
by Paul Gilster | Sep 26, 2007 | Exoplanetary Science |
How large a planet is depends upon its composition and mass. Earth is largely made of silicates, with a diameter of 7,926 miles at the equator. Imagine an Earth mass planet made of iron and you’re looking at a diameter of a scant 3000 miles. Interestingly, the relationship between mass and diameter follows a similar pattern no matter what material makes up the planet. Running the numbers, an Earth mass planet made of pure water will be 9500 miles across.
Sara Seager (Massachusetts Institute of Technology) has been studying these things as part of a project to model the kind of Earth-size planets we’re likely to find around nearby stars. About the mass/diameter pattern, she says this:
“All materials compress in a similar way because of the structure of solids. If you squeeze a rock, nothing much happens until you reach some critical pressure, then it crushes. Planets behave the same way, but they react at different pressures depending on the composition. This is a big step forward in our fundamental understanding of planets.”
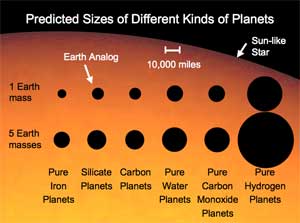
It’s a needed step, too, because we often speak of Earth-size planets as if they were likely to resemble the worlds we see in our own Solar System. The team, made up of scientists from MIT, NASA and the Carnegie Institution of Washington, wants to throw out that assumption, going back to the nature of the protoplanetary disks we’re seeing around young stars. Its speculations have produced fourteen different compositions, among them pure water ice, carbon, iron, silicates, carbon monoxide and silicon carbide. Corresponding sizes can be calculated for each planet.
“We have learned that extrasolar giant planets often differ tremendously from the worlds in our solar system, so we let our imaginations run wild and tried to cover all the bases with our models of smaller planets,” says Marc Kuchner (NASA GSFC). “We can make educated guesses about where these different kinds of planets might be found. For example, carbon planets and carbon-monoxide planets might favor evolved stars such as white dwarfs and pulsars, or they might form in carbon-rich disks like the one around the star Beta Pictoris. But ultimately, we need observations to give us the answers.”
Image: Astronomers have calculated the diameters of various types of planets given certain compositions and masses. This image shows the relative sizes of six different kinds of planets with different compositions, and depending on whether they have the same mass as Earth, or five times the mass of Earth. Note that the 5-Earth-mass planets are larger than their 1-Earth-mass counterparts, but they are not five times larger due to the gravitational compression that occurs when a planet’s mass is increased. The planets are shown silhouetted against the Sun, as if they are transiting planets seen from afar. Credit: Marc Kuchner/NASA GSFC.
Comparing a planet’s size and mass with the help of planetary transits is a first step toward determining its composition. The French COROT satellite should be capable of finding planets not much larger than Earth as they pass across the surface of their star as seen from the spacecraft. One tricky call will be a silicate planet vs. a carbon planet — the two model out to roughly the same size for a given mass. Maybe by the time we need to make such distinctions we’ll have the James Webb Space Telescope around for help.
And this comment in the paper’s conclusion on a definition for ‘super Earths’ is interesting:
Planets above the H2O [mass-radius] curve must have a significant H/He envelope. We can therefore easily distinguish between exoplanets with significant H/He envelopes and those without, as is the case for GJ 436b. We therefore define a “super Earth” to be a solid planet with no significant gas envelope, regardless of its mass.
The paper is Seager, Kuchner et al., “Mass-Radius Relationships for Solid Exoplanets,” now in press at The Astrophysical Journal, with publication due in late October (abstract).