by Paul Gilster | Oct 29, 2010 | Outer Solar System |
Here’s an interesting bit of news from the New Horizons team. Remember that the spacecraft, having made its pass by the Pluto/Charon system in 2015, will be moving ever deeper into the Kuiper Belt. It’s been the hope of mission planners that a close study of one or more objects there might be possible. Now astronomer Scott Sheppard (Carnegie Institution of Washington) has announced that he has detected the first asteroid in Neptune’s trailing Trojan zone (the planet’s L5 point), an area New Horizons will fly through before arriving at the Pluto/Charon binary.
2008 LC18 is not itself in range for a New Horizons flyby, but mission principal investigator Alan Stern notes its significance in a recent report on the mission’s Web site: ” …its discovery shows that additional and potentially closer Neptune Trojans that New Horizons might be able to study could be discovered in the next three years.” And that gives us an interesting mission extension for New Horizons, to take advantage of the instrumentation we’ve put deep into the outer system. Closest approach to Pluto occurs in 1719 days.
Colors of a KBO
Thus far we’ve been able to image about 1000 objects in the Kuiper Belt, and it’s interesting to note that they come in a range of colors. Work at NASA GSFC (Greenbelt, MD) now offers up a computer model that tells us how the icy bodies acquire their red, blue or white tints. The model is based on incoming radiation and its effects on the different layers of a KBO.
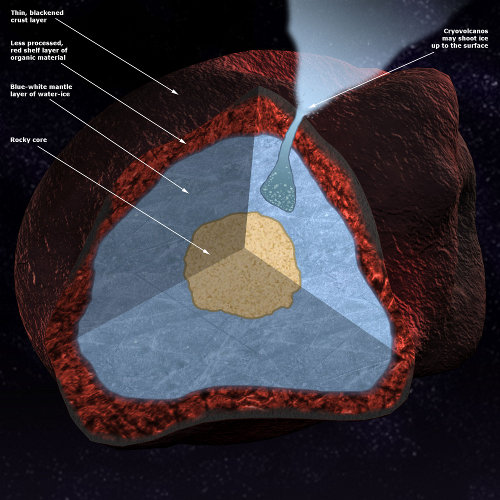
Image: This cutaway model shows a red “shelf” layer of a Kuiper Belt object peeking through the thin, darkened crust above so that the object appears red in telescopes. Credit: NASA/Conceptual Image Lab/Tyler Chase.
Active Processes Deep in the Outer System
I find Kuiper Belt objects utterly fascinating, and not least because of the possible astrobiological interest. Eris, for example, has a bright, icy surface. According to the GSFC model, deeper layers of relatively pure water ice could erupt upwards to form new outer layers on KBOs, accounting for Eris’ brightness. The potential for active processes deep within a KBO is intriguing, and I remember discussing astrobiological possibilities with Joel Poncy at last year’s Aosta conference. Poncy and team (Thales Alenia Space) were investigating a fast orbiter mission to Haumea, which is, like Eris, highly reflective and evidently covered with water ice.
Poncy’s point was that if you’re going to study KBOs in terms of astrobiology, Haumea isn’t the place, because its unusual shape (a flattened ellipsoid) probably derives from a major collision, which would have disrupted the interior processes we want to study. But we now have numerous objects with diameters over 500 kilometers beyond the orbit of Neptune, and we’ll doubtless find hundreds more in coming years. Some smaller KBOs, like 2002 TX300, are possibly the result of the same collision that produced Haumea. 2002 TX300 is highly reflective, its ice covering evidently fresh and thus somehow resurfaced periodically. The case for life inside a KBO is slim, but these intriguing objects may teach us something about life’s early chemistry.
Explaining How Colors Emerge
But back to the GSFC work. The colors of Kuiper Belt Objects seem related to the different sizes and orbits we’ve observed since 1992, when the first KBO, 1992 QB1, was discovered. John Cooper, a physicist at Goddard, takes note of KBO diversity:
“There’s a group called the Cold Classicals that move in relatively circular orbits, and are nearly aligned in the same plane as the orbits of the other planets. These are all consistently reddish. Other objects, which might range from red to blue to white, tend to move in more elliptical or inclined orbits, which suggest they came from a different location within the solar system early in its history. So, it’s possible that the uniformly red Cold Classicals represent a more pristine sample, showing the original composition of the Kuiper Belt with minimal disturbances.”
Cooper’s work tells us that radiation should affect different objects in different ways, depending on their location. The so-called Cold Classicals would have formed in an area where plasma ions from the Sun aren’t intense enough to darken the KBO’s outermost surface. Instead, the plasma ions ‘sandblast’ the topmost layer to expose the layer immediately below, with further erosion being produced by dust grains from collisions in the belt. Given enough time, simple chemical reactions producing organic molecules, a kind of radiation ‘cooking’ process mediated by radiation from interstellar space, can then produce the red tint we see on many KBOs.
Ice and Complex Molecules
White Kuiper Belt objects also fit within Cooper’s layer model, which assumes water ices in a deep mantle layer that can erupt onto the surface to leave bright icy patches. “So these may not be dead icy objects,” says Cooper, “they may be volcanically active over billions of years.” Usefully, New Horizons’ pass through this region may yield better surface observations not just of Pluto and Charon but objects beyond that will help us confirm what materials are present. And its readings of the energy distribution and particle count may confirm the factors needed to make Cooper’s model work.
And about astrobiology in the Kuiper Belt?
“When you take the right mix of materials and radiate them, you can produce the most complex species of molecules,” says Cooper. “In some cases you may be able to produce the components of life — not just organic materials, but biological molecules such as amino acids. We’re not saying that life is produced in the Kuiper Belt, but the basic chemistry may start there, as could also happen in similar Kuiper Belt environments elsewhere in the universe and that is a natural path which could lead toward the chemical evolution of life.”
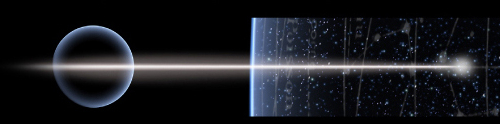
by Paul Gilster | Oct 28, 2010 | Exoplanetary Science |
Finding Earth-size planets around other stars is a long-cherished goal, and new results from Geoffrey Marcy and Andrew Howard (UC Berkeley) give us reason to think they’re out there in some abundance. As reported in Science, the astronomers have used the 10-meter Keck telescopes in Hawaii to make radial velocity measurements of 166 G and K-class stars within 80 light years of Earth. The resulting five years of data suggest that about one in every four stars like the Sun could have Earth-size planets, although none has thus far been detected.
“Of about 100 typical Sun-like stars, one or two have planets the size of Jupiter, roughly six have a planet the size of Neptune, and about 12 have super-Earths between three and 10 Earth masses,” said Howard, a research astronomer in UC Berkeley’s Department of Astronomy and at the Space Sciences Laboratory. “If we extrapolate down to Earth-size planets — between one-half and two times the mass of Earth — we predict that you’d find about 23 for every 100 stars.”
Howard and Marcy were homing in on close-in planets, but their findings support the possibility of finding more Earth-sized planets at greater distances, and that includes worlds in the habitable zone. But the findings seem at variance with some models of planet migration, which suggest that interactions in the gas disk around the star would cause many planets to spiral inward. That would create what the researchers call a ‘planet desert’ in the inner region of solar systems, one that these two researchers do not see in their findings. Says Marcy:
“Just where we see the most planets, models predict we would find no cacti at all. These results will transform astronomers’ views of how planets form.”
And let me quote the abstract on this:
Theoretical models of planet formation predict a deficit of planets in the domain from 5 to 30 Earth masses and with orbital periods less than 50 days. This region of parameter space is in fact well populated, implying that such models need substantial revision.
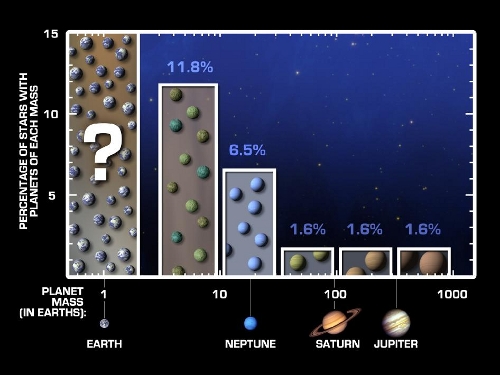
Image: The data, depicted here in this illustrated bar chart, show a clear trend. Small planets outnumber larger ones. Astronomers extrapolated from these data to estimate the frequency of the Earth-size planets — nearly one in four sun-like stars, or 23 percent, are thought to host Earth-size planets orbiting close in. Each bar on this chart represents a different group of planets, divided according to their masses. In each of the three highest-mass groups, with masses comparable to Saturn and Jupiter, the frequency of planets around sun-like stars was found to be 1.6 percent. For intermediate-mass planets, with 10 to 30 times the mass of Earth, or roughly the size of Neptune and Uranus, the frequency is 6.5 percent. And the super-Earths, weighing in at only three to 10 times the mass of Earth, had a frequency of 11.8 percent. NASA/JPL-Caltech/UC Berkeley
Out of the 166 stars surveyed, 22 had detectable planets, with 33 planets being found in all (twelve planet candidates are still in the process of confirmation, which could raise the total as high as 45 planets around 32 stars). We’ll get another read on this from Kepler, for plugging these conclusions into its survey of 156,000 stars should yield 120 to 260 ‘plausibly terrestrial worlds’ with orbital periods of less than 50 days around G and K stars. Adds Howard: “One of astronomy’s goals is to find eta-Earth, the fraction of Sun-like stars that have an Earth. This is a first estimate, and the real number could be one in eight instead of one in four. But it’s not one in 100, which is glorious news.”
Needless to say, Earth-sized planets orbiting a Sun-like star at roughly one quarter of an AU do not make for habitable places, but these are statistical calculations that give us a rough read on what to expect. If we follow up with the assumption that thus far undetected Earth-sized planets should also form in the habitable zone, then G and K stars should yield numerous targets for future terrestrial planet hunter missions. We’re still looking for a ‘second Earth’ in the habitable zone of a Sun-like star, but these calculations suggest the prospects are promising.
The paper is Marcy and Howard, “The Occurrence and Mass Distribution of Close-in Super-Earths, Neptunes, and Jupiters,” Science Vol. 330, No. 6004 (29 October, 2010), pp. 653 – 655 (abstract).
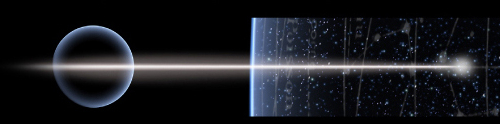
by Paul Gilster | Oct 27, 2010 | Asteroid and Comet Deflection |
It’s good to see asteroid deflection occasionally popping up in the news, thanks to the efforts of people like former astronaut Rusty Schweickart, whose efforts as co-chairman of the Task Force on Planetary Defense of the NASA Advisory Council are complemented by his work for non-profits like the B612 Foundation. Schweickart is worried about the potential consequences of even a small asteroid impact, pointing to the Tunguska event of 1908, in which 800 square miles of Siberian forest were flattened in the kind of strike that occurs every 200 to 300 years.
Bigger asteroids are, obviously, a far greater danger, and while they’re much rarer, they do have the capability of wiping out entire species, as may well have occurred some 65 million years ago in the destruction of the dinosaurs. In his recent New York Times article, Schweickart notes what we need to do:
With a readily achievable detection and deflection system we can avoid their same fate. Professional (and a few amateur) telescopes and radar already function as a nascent early warning system, working every night to discover and track those planet-killers. Happily, none of the 903 we’ve found so far seriously threaten an impact in the next 100 years.
Nonetheless, asteroids demand a constant vigilance. Schweickart continues:
Although catastrophic hits are rare, enough of these objects appear to be or are heading our way to require us to make deflection decisions every decade of so.
A deflection capacity is something NASA needs to be looking at, and the report of the Task Force on Planetary Defense urges that financing for it be added to the NASA budget. Schweickart believes that $250 to $300 million, added annually over the next ten years, would allow our inventory of near-Earth asteroids to be completed and a deflection capability to be developed, after which a maintenance budget ($50 to $75 million per year) would keep us tuned up for potential deployment.
Underscoring the need for a deflection capability is the work of Elisabetta Pierazzo (Planetary Science Institute), whose forthcoming paper in Earth and Planetary Science Letters focuses on two impact scenarios, 500-meter and 1-kilometer asteroids hitting a 4-kilometer deep ocean. What Pierazzo finds is that an ocean strike could deplete the Earth’s protective ozone layer for several years, resulting in a spike in ultraviolet radiation levels that would, among other things, make it more difficult to grow crops (not to mention its effects on other life forms).
Pierazzo and team’s atmospheric simulations show a global perturbation of upper atmosphere chemistry, as water vapor and compounds like chlorine and bromide alter the ozone layer to create a new ozone hole. Adds Pierazzo:
“The removal of a significant amount of ozone in the upper atmosphere for an extended period of time can have important biological repercussions at the Earth’s surface as a consequence of increase in surface UV-B irradiance. These include increased incidence of erythema (skin reddening), cortical cataracts, changes in plant growth and changes in molecular DNA.”
Ultraviolet radiation intensity can be expressed by the ultraviolet index (UVI), which indicates the intensity of UV radiation at the surface, with the higher numbers tending toward damage to skin and eyes. While a UVI of 10 is considered dangerous, resulting in burns to fair-skinned people after short exposure, values up to 18 are occasionally recorded at the equator. The highest recorded UVI is 20, recorded at a high-altitude desert in Puna de Atacama, Argentina.
Modeling a strike by an asteroid that hit at latitude 30 degrees north in the Pacific Ocean in January, Pierazzo’s simulations show that a 500-meter asteroid impact would result in a major ozone hole, boosting UVI values to over 20 for several months in the northern subtropics. A 1-kilometer asteroid would drive the UVI in certain areas to a sizzling 56, while boosting UVI values over 20 within a 50-degree latitude band north and south of the equator for about two years. The affected band’s northern end would include Seattle and Paris, while its southern end reached New Zealand and Argentina.
“A level of 56 has never been recorded before, so we are not sure what it is going to do,” adds Pierazzo. “It would produce major sunburn. We could stay inside to protect ourselves, but if you go outside during daylight hours you would burn. You would have to go outside at night, after sunset, to avoid major damage.”
We always tend to depict asteroid impacts in terms of their direst consequences as a way of illustrating the magnitude of the threat. But it’s chastening to learn that even a survivable impact like those Pierazzo and team have modeled would create serious environmental damage even if loss of life could be prevented. All this assumes, too, an asteroid that strikes in the ocean (the most likely scenario). There’s no question that building up our planetary defense against such impacts is the best insurance we could create, stopping potential impactors before they near our planet.
The paper is Pierazzo et al., “Ozone perturbation from medium-size asteroid impacts in the ocean,” in press at Earth and Planetary Science Letters (abstract). Jeremy Hsu’s article on this work in LiveScience is excellent.
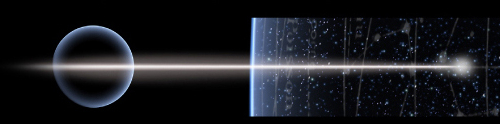
by Paul Gilster | Oct 26, 2010 | Exoplanetary Science |
With three groups now looking hard at Alpha Centauri for planets, let’s hope our nearest stars don’t do for us what Gliese 581 has. First we had a habitable planet in Gl 581c, then we didn’t. Then Gl 581d looked a bit promising, and may skirt the outer edges of the habitable zone, although the jury is still out. Gl 581g looked to be the winner, the fabled ‘Goldilocks’ planet, but now the evidence for it seems weak and its existence is called very much into doubt. Gl 581 keeps dealing out winners and then calling them back, a frustrating period for all concerned.
What we’d like to find at Alpha Centauri, then, is something unambiguous. But while we wait for answers, the issue of how planets form in close binary systems like Alpha Centauri is under the microscope. Centauri A and B have a mean separation of 23 AU, closing to within 11.2 AU (think of another star as close to ours as Saturn) and receding up to 35.6 AU (roughly Pluto’s distance). Proxima is much further out at 13,000 AU and is the nearest individual star to the Sun. We know there are feasible planetary orbits around Centauri A and B. The question is how small, rocky worlds might form in this environment in the first place.
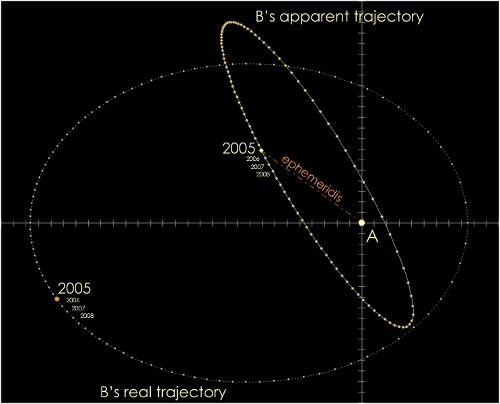
Image: Apparent and true orbits of Alpha Centauri. Motion is shown from the A component against the relative orbital motion of the B component. The apparent orbit (thin ellipse) is the shape of the orbit as seen by the observer on Earth. The true orbit is the shape of the orbit viewed perpendicular to the plane of the orbital motion. Credit: Wikimedia Commons.
Growing Planetesimals Out of the Dust
We saw yesterday that the initial phases of planet formation are at issue. In the core accretion model, planetesimals form from a dust disk and, through a series of collisions, eventually form into planetary embryos. Much remains to be learned about the transition from dust to planetesimal, as well as the planetesimal collision stage. A new paper by Ji-Wei Xie (Nanjing University) et al. looks at the transition between planetesimal formation and the beginning of the collisions between them, a period the paper refers to as the ‘snowball phase.’
Where this departs from older work is that many earlier scenarios for growing planetesimals start with the planetesimals in place and go from there. The new paper questions this approach, positing the snowball phase as a transition period when isolated planetesimals exist in a disk where most of the mass is still in the form of dust grains:
We outline a new growth mode, which dominates during this early phase, during which planetesimals experience negligible gravitational or collisional interactions with one another, and grow mainly (or solely) via the accretion of dust or ice that they sweep up?in the manner of a rolling snowball. In this paper, we refer to this dust-fueled planetesimal growth phase as the “snowball” growth phase.
What the paper finds is that in close binaries, snowball growth overcomes a key problem, that high impact velocities prevent kilometer-sized planetesimals from growing into planetary embryos:
Indeed, this dynamically excited environment, which is hostile to mutual planetesimal accretion, is on the contrary favorable to growth by dust sweeping. If efficient enough, snowball growth allows planetesimals to grow large enough, 50-100 km, to be protected from mutually destructive impacts.
Viable Planetary Growth Around Close Binaries
Going from planetesimals to planetary cores, then, becomes a viable process once the objects are large enough (the 50 to 100 kilometers mentioned above) to survive high speed collisions. The ‘snowball’ model allows the planetesimals time to grow through the accretion of ice and dust that is swept up in the manner of a rolling snowball. Snowball growth as defined here begins with the formation of the first planetesimal and ends when planetesimal collisions become the dominant growth mode. The paper derives the snowball growth rate, shows how long it will last, and considers how the planetesimals can grow around single stars and binaries.
All of this winds up looking promising for environments like Alpha Centauri, and again, its scenario is possible because the authors are defining a growth phase where the planetesimals are emerging from the dust disk but are still sparse — not yet in an era of mutual planetesimal annihilation — growing by sweeping up dust in the protoplanetary disk. Snowball growth is a mechanism that, the authors point out, needs more detailed studies to improve its modeling. I suspect that before we get too far along in that process, we’ll have news from our planet hunting teams about the presence (or lack of same) of planets around Centauri A and B.
The paper is Ji-Wei Xie et al., “From Dust To Planetesimal: The Snowball Phase?” accepted by The Astrophysical Journal and available as a preprint.
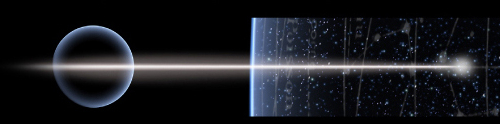
by Paul Gilster | Oct 25, 2010 | Exoplanetary Science |
Planets around binary stars fascinate me, doubtless because of Alpha Centauri’s proximity and the question of whether there are planets there. About ten percent of the planets we’ve found around main sequence stars are found in binary systems, and most of these binaries have wide separations, in the range of 100 to 300 AU. But, like Alpha Centauri, close binaries remain promising targets. I’m looking at a new paper by Andras Zsom, Zsolt Sándor and Kees Dullemond (Max-Planck-Institute für Astronomie) dealing with early stage planet formation in binaries, and they’re quick to note that planets in close binary systems put constraints on planet formation theories.
After all, if we find planets in these systems, our planet forming theories have to produce satisfactory explanations for their existence. Does core accretion, then, work in these environments? We can look to close binaries with planets, systems like Gamma Cephei (separation 18.5 AU), GL 86 (18.4 AU), HD 41004 (23 AU) and HD 196885 (17 AU). What Zsom et al. are interested in is the question of the growth and fragmentation of dust in binary systems, the gas and dust disk around the primary star being perturbed by the secondary, and their paper compares their model — with and without eccentricity — to the dust population in disks around single stars.
Core Accretion and Its Problems
Think about how planets form under the core accretion model. Tiny dust grains eventually form planetesimals, which then form protoplanetary cores that over time gain a gaseous envelope (or not, depending on the presence of gas), and undergo a chaotic impact phase until their orbits are finally stable. It’s a complicated picture that binary stars make even more treacherous, and in several ways. For one thing, contemplate what a stellar companion does to the dust disk:
The tidal torques of the companion generate strong spiral arms in the disk around the primary. Angular momentum is transferred to the binary orbit which will truncate and restructure the disk… This dynamical effect of the secondary has several consequences which might influence planet formation: it decreases the lifetime of the disk, increases the temperature of the disk and modifies the stable orbits around the primary.
But it’s not just the early disk that’s affected. Here the authors go into what happens to the evolving planetesimals, and how their fate may shape planet formation:
The evolution of planetesimals is also influenced in a binary system. The perturbation of the secondary increases the relative velocity of the planetesimals and/or creates unstable regions where the planetary building blocks cannot maintain a stable orbit as shown by e.g., Heppenheimer (1978), Whitmire et al. (1998), Thébault et al. (2004). The increased relative velocities can then lead to the disruption of the planetesimals. However, Marzari & Scholl (2000) showed that the combined effects of the gravitational perturbation and the gas drag may increase the efficiency of their accretion by reducing their relative velocity and produce, later on, terrestrial planets. Marzari et al. (2009) calculated the relative velocity of planetesimals in highly inclined systems and concluded that planet formation appears possible for inclinations as high as 10? , if the separation between the stars is larger than 70 AU. The region where planetesimals can accumulate into protoplanets shrinks consistently for lower binary separations.
Of course, it’s the closer binaries that we’re interested in today. This paper homes in on the earliest stages of core accretion, the key issue being: can planetesimals form in the first place, or is the disk too thoroughly disrupted to allow the process? Some theories suggest that a ‘pressure bump’ forming around the snow-line could concentrate particles so that planetesimals could form in the inner system, but the authors’ simulations show that this effect quickly disappears in infant systems, so quickly that the needed planetesimals never emerge.
Various other models are out there that could concentrate particles as needed for the early stages of planet formation — the authors run through the possibilities — but they’re still under investigation and may be rendered unworkable by the presence of the secondary star. As the authors note, “Particle concentration mechanisms in general need to cope with the continuous gravitational stirring and perturbations of the secondary.” And we’re by no means sure that core accretion can function within these models when a secondary star is present.
A Different Formation Model?
If that’s the case, what about the planets we’ve already found around close binaries? Are they the result not of core accretion but gravitational instability, the alternate model of planet formation? In the latter, unstable regions in the protoplanetary disk form clumps of gas and dust which eventually coagulate into a single core. In this model, knots of matter collapse rapidly, to form planets in a much shorter time frame.
The gas giants we’ve found around close binaries thus far could be the result of gravitational instability. But here we have to fall back on the limits of our observations, for as some readers have already been discussing in the comments to a previous post, close binaries are tricky targets. We have yet to find low-mass planets around close binaries, but this may simply be because of the limits of our current techniques, and because of an observational bias that has kept such systems out of many early surveys. But if further work rules out core accretion in these environments, then the alternate model — gravitational instability — becomes the focus of more targeted study.
How dust turns into planetesimals, and thence to planets, is the subject of intense ongoing work. And if the Zsom paper has you discouraged about the prospects around Alpha Centauri, we’ll look tomorrow at another new paper that tackles early disk issues and comes to a different conclusion about what may be possible there. For today, though, the paper is Zsom et al., “The First Stages of Planet Formation in Binary Systems: How Far Can Dust Coagulation Proceed?” accepted at Astronomy & Astrophysics and available as a preprint. You may want to check the comments to my recent article on the SIM mission, where this paper first came up (and thanks to Centauri Dreams regulars andy and spaceman for the discussion).
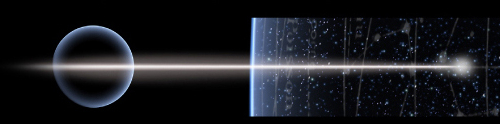