by Paul Gilster | Jul 9, 2021 | Advanced Rocketry, Uncategorized |
Building a Bussard ramjet isn’t easy, but the idea has a life of its own and continues to be discussed in the technical literature, in addition to its long history in science fiction. Peter Schattschneider, who explored the concept in Crafting the Bussard Ramjet last February, has just published an SF novel of his own called The EXODUS Incident (Springer, 2021), where the Bussard concept plays a key role. But given the huge technical problems of such a craft, can one ever be engineered? In this second part of his analysis, Dr. Schattschneider digs into the question of hydrogen harvesting and the magnetic fields the ramjet would demand. The little known work of John Ford Fishback offers a unique approach, one that the author has recently explored with Centauri Dreams regular A. A. Jackson in a paper for Acta Astronautica. The essay below explains Fishback’s ideas and the options they offer in the analysis of this extraordinary propulsion concept. The author is professor emeritus in solid state physics at Technische Universität Wien, but he has also worked for a private engineering company as well as the French CNRS, and has been director of the Vienna University Service Center for Electron Microscopy.
by Peter Schattschneider
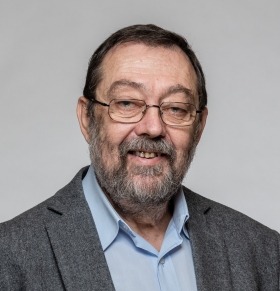
As I mentioned in a recent contribution to Centauri Dreams, the BLC1 signal that flooded the press in January motivated me to check the science of a novel that I was finishing at the time – an interstellar expedition to Proxima Centauri on board a Bussard ramjet. Robert W. Bussard’s ingenious interstellar ramjet concept [1], published in 1960, inspired a generation of science fiction authors; the most celebrated is probably Poul Anderson with the novel Tau Zero [2]. The plot is supposedly based on an article by Carl Sagan [3] who references an early publication of Eugen Sänger where it is stated that due to time dilation and constant acceleration at 1 g „[…] the human lifespan would be sufficient to circumnavigate an entire static universe“ [4].
Bussard suggested using magnetic fields to scoop interstellar hydrogen as a fuel for a fusion reactor, but he did not discuss a particular field configuration. He left the supposedly simple problem to others as Newton did with the 3-body problem, or Fermat with his celebrated theorem. Humankind had to wait 225 years for an analytic solution of Newton‘s problem, and 350 years for Fermat’s. It took only 9 years for John Ford Fishback to propose a physically sound solution for the magnetic ramjet [5].
The paper is elusive and demanding. This might explain why adepts of interstellar flight are still discussing ramjets with who-knows-how-working superconducting coils that generate magnetic scoop fields reaching hundreds or thousands of kilometres out into space. Alas, it is much more technically complicated.
Fishback’s solution is amazingly simple. He starts from the well known fact that charged particles spiral along magnetic field lines. So, the task is to design a field the lines of which come together at the entrance of the fusion reactor. A magnetic dipole field as on Earth where all field lines focus on the poles would do the job. Indeed, the fast protons from the solar wind are guided towards the poles along the field lines, creating auroras. But they are trapped, bouncing between north and south, never reaching the magnetic poles. The reason is rather technical: Dipole fields change too rapidly along the path of a proton in order to keep it on track.
Fishback simply assumed a sufficiently slow field variation along the flight direction, Bz=B0/(1+ ? z) with a „very small“ ?. Everything else derives from there, in particular the parabolic shape of the magnetic field lines. Interestingly, throughout the text one looks in vain for field strengths, let alone a blueprint of the apparatus. The only hint to the visual appearance of the device is a drawing of a long, narrow paraboloid that would suck the protons into the fusion chamber. As a shortcut to what the author called the region dominated by the ramjet field I use here the term „Fishback solenoid“.
Fig. 1 is adapted from the original [5]. I added the coils that would create the appropriate field. Their distance along the axis indicates the decreasing current as the funnel widens. Protons come in from the right. Particles outside the scooping area As are rejected by the field. The mechanical support of the coils is indicated in blue. It constitutes a considerable portion of the ship’s mass, as we shall see below.
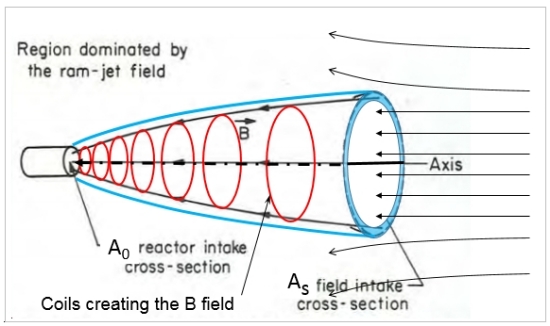
Fig. 1: Fishback solenoid with parabolic field lines. The current carrying coils are symbolized in red. The mechanical support is in blue. The strong fields exert hoop stress on the support that contributes considerably to the ship’s mass. Adapted from [5].
Searching for scientific publications that build upon Fishback’s proposal, Scopus renders 6 citations up to this date (April 2021). Some of them deal with the mechanical stress of the magnetic field, another aspect of Fishback’s paper that I discuss in the following, but as far as I could see the paraboloidal field was not studied in the 50 years since. This is surprising because normally authors continue research when they have a promising idea, and others jump on the subject, from which follow-up publications arise, but J. F. Fishback published only this one paper in his lifetime. [On Fishback and his tragic destiny, see John Ford Fishback and the Leonora Christine, by A. A. Jackson].
Solving the dynamic equation for protons in the Fishback field proves that the concept works. The particles are guided along the parabolic field lines toward the reactor as shown in the numerical simulation Fig. 2.
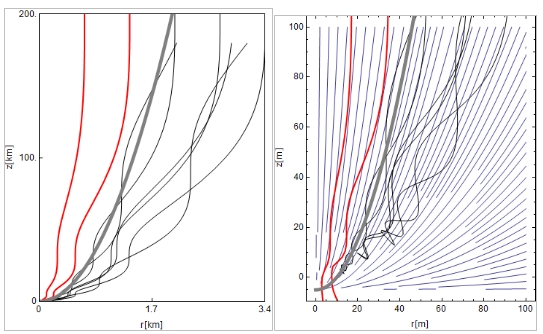
Fig.2: Proton paths in an (r,z)-diagram. r is the radial distance from the symmetry axis, z is the distance along this axis. The ship flies at 0.56 c (?=0.56) in positive z-direction. In the ship’s rest frame, protons arrive with a kinetic energy of 194 MeV from the top. Left: Protons entering the field at z=200 km are focussed to the reactor mouth at the coordinate origin, gyrating over the field lines. Particles following the red paths make it to the chamber; protons following the black lines spiral back. The thick grey parabola separates the two regimes. Right: Zoom into the first 100 m in front of the reactor mouth of radius 10 m. Magnetic field lines are drawn in blue.
The reactor intake is centered at (r,z)=(0,0). In the ship’s rest frame the protons arrive from top – here with 56 % of light speed, the maximum speed of the EXODUS in my novel [8]. Some example trajectories are drawn. Protons spiral down the magnetic field lines as is known from earth’s magnetic field and enter the fusion chamber (red lines). The scooping is well visible. The reactor mouth has an assumed radius of 10 m. A closer look into the first 100 m (right figure) reveals an interesting detail: Only the first two trajectories enter the reactor. Protons travelling beyond the bold grey line are reflected before they reach the entrance, just as charged particles are bouncing back in the earth’s field before they reach the poles. From the Figure it is evident that at an axial length of 200 km of the Fishback solenoid the scoop radius is disappointingly low – only 2 km. Nevertheless, the compression factor (focussing ions from this radius to 10 m) of 1:40.000 is quite remarkable.
The adiabatic condition mentioned above allows a simple expression for the area from which protons can be collected. The outer rim of this area is indicated by the thick grey line in Fig. 2. The supraconducting coils of the solenoid should ideally be built following this paraboloid, as sketched in Fig. 1. Tuning the ring current density to

yields a result that approximates Fishback‘s field closely.
What does it mean in technical terms? Let me discuss an idealized example, having in mind Poul Anderson’s novel. The starship Leonora Christina accelerates at 1 g, imposing artificial earth gravity on the crew. Let us assume that the ship‘s mass is a moderate 1100 tons (slightly less than 3 International Space Stations). For 1 g acceleration on board, we need a peak thrust of ~11 million Newton, about 1/3 of the first stage of the Saturn V rocket. The ship must be launched with fuel on stock because the ramjet operates only beyond a given speed, often taken as 42 km/s, the escape velocity from the solar system. In the beginning, the thrust is low. It increases with the ship’s speed because the proton throughput increases, asymptotically approaching the peak thrust.
Assuming complete conversion of fusion energy into thrust, total ionisation of hydrogen atoms, and neglecting drag from deviation of protons in the magnetic field, at an interstellar density of 106 protons/m3, the „fuel“ collected over one square kilometer yields a peak thrust of 1,05 Newton, a good number for order-of-magnitude estimates. That makes a scooping area of ~10 million square km, which corresponds to an entrance radius of about 1800 km of the Fishback solenoid. From Fig. 2, it is straightforward to extrapolate the bold grey parabola to the necessary length of the funnel – one ends up with fantastic 160 million km, more than the distance earth – sun. (At this point it is perhaps worth mentioning that this contribution is a physicist’s treatise and not that of an engineer.)
Plugging the scooping area into the relativistic rocket equation tells us which peak acceleration is possible. The results are summarised in Table 1. For convenience, speed is given in units of the light speed, ß=v/c. Additionally, the specific momentum ß? is given where
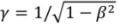
is the famous relativistic factor. (Note: The linear momentum of 1 kg of matter would be ß? c.) Acceleration is in units of the earth gravity acceleration, g=9.81 m/s2.
Under continuous acceleration such a starship would pass Proxima Centauri after 2.3 years, arrive at the galactic center after 11 years, and at the Andromeda galaxy after less than 16 years. Obviously, this is not earth time but the time elapsed for the crew who profit from time dilation. There is one problem: the absurdly long Fishback solenoid. Even going down to a scooping radius of 18 km, the supraconducting coils would reach out 16,000 km into flight direction. In this case the flight to our neighbour star would last almost 300 years.
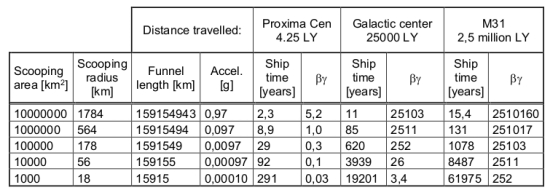
Table 1: Acceleration and travel time to Proxima Centauri, the galactic center, and the Andromeda galaxy M31, as a function of scooping area. ß? is the specific momentum at the given ship time. A ship mass of 1100 tons, reactor entrance radius 10 m, and constant acceleration from the start was assumed. During the starting phase the thrust is low, which increases the flight time by one to several years depending on the acceleration.
Fishback pointed out another problem of Bussard ramjets [5]. The magnetic field exerts strong outward Lorentz forces on the supraconducting coils. They must be balanced by some rigid support, otherwise the coils would break apart. When the ship gains speed, the magnetic field must be increased in order to keep the protons on track. Consequently, for any given mechanical support there is a cut-off speed beyond which the coils would break. For the Leonora Christina a coil support made of a high-strength „patented“ steel must have a mass of 1100 tons in order to sustain the magnetic forces that occur at ?=0,74.
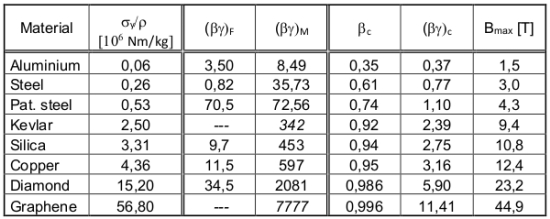
Table 2: Cut-off speeds ?c and cut-off specific momenta (ß?)c (upper bounds) for several support materials. (ß?)F from [5], (ß?)M from [7]. ?y/? is the ratio of the mechanical yield stress to the mass density of the support material. Bmax is the maximum magnetic field at the reactor entrance at cut-off speed. A scooping area of 10 million km2 was assumed, allowing a maximum acceleration of ~1 g for a ship of 1100 tons. Values in italics for Kevlar and graphene, unknown in the 1960s, were calculated based on equations given in [7].
But we assumed above that this is the ship‘s entire mass. That said, the acceleration must drop long before speeding at 0,74 c. The cut-off speed ?c=0,74 is an upper bound (for mathematicians: not necessarily the supremum) for the speed at which 1 g acceleration can be maintained. Lighter materials for the coil support would save mass. Fishback [5] calculated upper bounds for the speed at which an acceleration of 1 g is still possible for several materials such as aluminium or diamond (at that time the strongest lightweight material known). Values are shown in Table 2 together with (ß?)c.
Martin [7] found some numerical errors in [5]. Apart from that, Fishback used an optimistically biased (ß?)c. Closer scrutiny, in particular the use of a more realistic rocket equation [6], results in more realistic upper bounds. Using graphene, the strongest material known, the specific cut-off momentum is 11,41. This value would be achieved after a flight of three years at a distance of 10 light years. After that point, the acceleration would rapidly drop to values making it hopeless to reach the galatic center in a lifetime.
In conclusion, the interstellar magnetic ramjet has severe construction problems. Some future civilization may have the knowhow to construct fantastically long Fishback solenoids and to overcome the minimum mass condition. We should send a query to the guys who flashed the BLC1 signal from Proxima Centauri. The response is expected in 8.5 years at the earliest. In the meantime the educated reader may consult a tongue-in-cheek solution that can be found in my recent scientific novel [8].
Acknowledgements
Many thanks to Al Jackson for useful comments and for pointing out the source from which Poul Anderson got the idea for Tau Zero, and to Paul Gilster for referring me to the seminal paper of John Ford Fishback.
References
[1] Robert W. Bussard: Galactic Matter and Interstellar Flight. Astronautica Acta 6 (1960), 1-14.
[2] Poul Anderson: Tau Zero. Doubleday 1970.
[3] Carl Sagan: Direct contact among galactic civilizations by relativistic inter-stellar space flight, Planetary and Space Science 11 (1963) 485-498.
[4] Eugen Sänger: Zur Mechanik der Photonen-Strahlantriebe. Oldenbourg 1956.
[5] John F. Fishback: Relativistic Interstellar Space Flight. Astronautica Acta 15 (1969), 25-35.
[6] Claude Semay, Bernard Silvestre-Brac: The equation of motion of an interstellar Bussard ramjet. European Journal of Physics 26 (1) (2005) 75-83.
[7] Anthony R. Martin: Structural limitations on interstellar space flight. Astronautica Acta 16 (6) (1971) 353-357.
[8] Peter Schattschneider: The EXODUS Incident. Springer 2021,
ISBN: 978-3-030-70018-8. https://www.springer.com/de/book/9783030700188#aboutBook
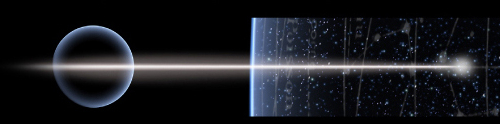
by Paul Gilster | Apr 12, 2021 | Uncategorized |
There is a public YouTube channel for watching the Breakthrough Discuss meetings, which began today and extend through tomorrow. Click here to go to sessions on “The Alpha Centauri System: A Beckoning Neighbor.” I’ll have thoughts on some of these presentations in coming weeks.
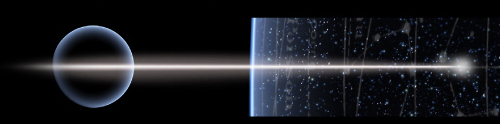
by Paul Gilster | Jan 11, 2021 | Uncategorized |
The news that NASA will extend the InSight mission on Mars for two years, taking it through December of 2022, is not surprising, given the data trove the mission team has collected through operation of the mission seismometer. A live asset on Mars also deepens our knowledge of the planet’s atmosphere and magnetic field, all reasons enough for pushing for another two years. But the extension of the Juno mission to Jupiter deserves more attention than it’s getting, given that Juno’s remit will be expanded deep into the Jovian system.
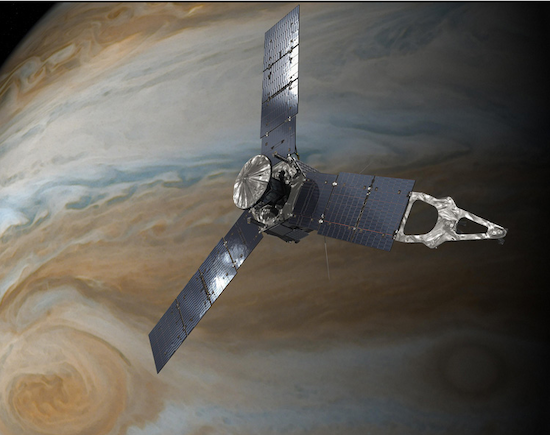
Image: NASA has extended both the Juno mission at Jupiter through September 2025 and the InSight mission at Mars through December 2022. Credit: NASA/JPL-Caltech.
For those of us fascinated with the outer system, this is good news indeed. I’m looking over two documents, the first being a presentation based on a report submitted to NASA’ Outer Planets Assessment Group (thanks to Ashley Baldwin for passing this along). The OPAG document was produced by Scott Bolton (Southwest Research Institute); it gives the overview of what a mission extension could look like. Also on my desk this morning is the text of the 2020 Planetary Missions Senior Review (PMSR), outlining a set of three mission scenarios. The context of both analyses is the success of the mission in studying Jupiter’s interior structure, magnetic field and magnetosphere, not to mention the examination of its atmospheric dynamics, seen in such roiling imagery as that depicted with stunning complexity in many of the JunoCam images.
Launched in 2011 and operational at Jupiter since 2016, Juno’s prime missions were to have ended in July of this year, with the spacecraft having completed 34 polar orbits, each of 53 day duration. The OPAG report refers to the subsequent extended mission as “a full Jovian system explorer with close flybys of satellites and rings.” The extended mission is to last through September, 2025, with observations of the planet’s ring system, its large moons, and a series of targeted observations and close flybys of Ganymede, Europa and Io.
That last clause really got my attention, as I hadn’t seen it coming. Juno is in an elliptical orbit with a 53-day period whose perijove migrates northward. This bit from the Senior Review reveals in depth the interactions between the various mission scenarios and satellite flybys. The three scenarios mentioned offer alternatives given varying science and budget considerations:
The proposed Juno extended mission (EM) would take advantage of the natural northward progression of the periapsis of the spacecraft’s orbit and the consequent lowering of spacecraft altitudes over Jupiter’s high northern latitudes. The EM would run until the end of the mission, with an expected duration of approximately four years. Under the High and Medium Scenarios, propulsive maneuvers would be utilized not only to target Jupiter-crossing longitude and perijove altitude, as during the prime mission, but also to target close flybys of Ganymede, Europa, and Io. The flyby maneuvers would act to shorten the spacecraft orbital period, yielding more close passes of Jupiter within a given time interval, and increase the rate of northward movement of spacecraft perijove. Under the Low scenario for EM operation, the satellite gravity assists and close satellite flybys would not be attempted.
So mission scientists have a number of options to work with. The extended mission investigates the northern hemisphere and probes the region above Jupiter’s polar cap aurora. The northern adjustment in Juno’s orbit is what makes the satellite flybys possible and enables as well close analysis of its ring structures. The Juno team can look forward to 3D mapping of Jupiter’s polar cyclones and studies of the planet’s unusual dilute core, the latter an earlier Juno discovery revealing a core consisting of both rocky material and ice as well as hydrogen and helium.
Both Europa Clipper and the European Space Agency’s JUICE mission (Jupiter Icy Moons Explorer) should benefit from Juno data on the radiation environment they will operate within. At Europa, Juno will continue the search for possible plume activity while examining the ice shell and mapping surface features, while studies of Io’s magma, polar volcanoes and interactions with Jupiter’s magnetosphere will be enabled by its encounters there. At Ganymede, magnetospheric interactions and surface composition data should be produced in abundance.
In the OPAG presentation, most of the Juno flybys will be at Io, with 11 possible between mid-2022 and 2025. Two encounters are planned for Ganymede (and recall that JUICE is scheduled to orbit the huge moon), and three encounters are feasible for Europa. The actual number of flybys will, according to the Senior Review, depend upon budget choices. In that document, I find this overview of Juno’s satellite flybys:
The orbit of Juno in the EM [extended mission] would take the spacecraft through the Io and Europa plasma tori and in close proximity to Io, Europa and Ganymede. Maps of Ganymede’s surface composition would allow studies to understand the importance of radiolytic processes in surface weathering, identify changes since Voyager and Galileo, and search for new craters. Juno’s Microwave Radiometer (MWR) is particularly sensitive to the upper 10 km of Europa’s ice shell. Studies at wavelengths complementing expected results from Europa Clipper’s radar would identify regions of thick and thin ice and search for regions where shallow subsurface liquid may exist. Juno’s visible and low-light cameras would search Europa for active plumes and changes in color/albedo that may reveal eruption regions since Galileo. The fields and particles experiments would look for evidence of recent activity. Finally, the Juno EM would include a flyby of Io and search for evidence of a magma ocean.
What an interesting development Juno’s extended mission turns out to be! Continuing science operations with existing equipment far undercuts the cost of new missions while extending long-duration datasets and, in the case of Juno, enabling a set of exciting new targets. We have the option here of a series of Galilean moon flybys that were never in Juno’s original mission, observations that could inform later choices made for Europa Clipper and JUICE. All told, Juno’s unanticipated extended mission is a heartening contribution to outer system science.
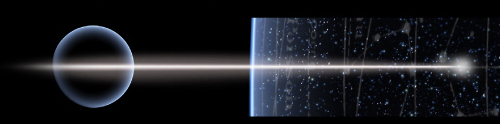
by Paul Gilster | Jan 7, 2021 | Uncategorized |
Henry Cordova, whose recent critique of traditional SETI kicked off a lengthy discussion in these pages, has been mulling over issues of habitability in the galaxy’s vast population of red dwarf stars. While we’ve focused on the questions raised by stellar flare activity and the climate challenges of tidal lock, the narrow band of habitability among the fainter M-dwarfs poses its own problems. How big a factor is a narrow circumstellar habitable zone? Henry comes by his interest in these matters by way of US Navy training in both astronomy and mathematics. A retired geographer and map maker now living in southeastern Florida, he’s keeping up with exoplanetary issues as an active amateur astronomer and collector of star atlases.
by Henry Cordova
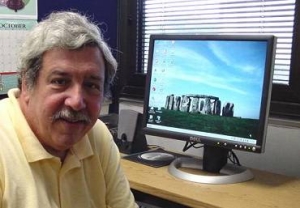
I am curious as to how the width of a star’s habitable zone varies with respect to its luminosity.
It would not be unreasonable to assume that the surface temperature of a planet is directly related to the radiant flux of its star. Furthermore, it seems reasonable that the range of surface temperatures in which water can be a liquid on at least part of a planet’s surface is directly related to the stellar flux at its orbital distance. There may be many other factors involved, such as the properties of the planet’s atmosphere, its rotational characteristics, orbital elements and the variability and spectrum of its parent star; but let us ignore them for the moment and simply consider the geometrical parameters involved.
All else being equal, the radiant flux received by the planet must then be directly proportional to the luminosity of the star, and inversely proportional to the square of the planet’s distance from it. In other words, if one star is a hundred times more luminous than another, a planet orbiting the fainter star must be ten times closer to its primary in order to receive the same flux. The same reasoning can be applied to both the inner and outer edges of the star’s habitable zone. Regardless of how we define the HZ, it will become much narrower as the luminosity of the primary decreases. And the narrower an HZ is, the less likely there is a planet there.
Consider our own Sun’s habitable zone. Although there is some controversy about its dimensions, let us for the purposes of this argument say that it is limited by the orbits of Mars and Venus.The two planets have semi-major orbital axes of roughly 1.5 and 0.7 AU, respectively. These two figures mark the limits of Sol’s HZ, and their difference gives an HZ width of 0.8 AU, plenty of room to squeeze Earth in.
If our Sun were a hundred times less luminous, the HZ boundaries would be at 0.15 and 0.07 AU, which translates to an HZ width of only 0.08 AU! Clearly, the HZs of faint stars can be very narrow. The chances of a planet forming there, or migrating in, are substantially reduced.
Astronomers have detected planets in the HZs of some red dwarfs, but I feel this is due primarily to selection effects. Many of our planet detection techniques are very sensitive to big planets orbiting small stars in close, highly elliptical orbits, circumstances which are not conducive to life and yielding statistics that may give us a distorted idea of how solar systems form. Because of these considerations, red dwarfs may not be good candidates for life, even if we disregard other problems such as flares and tidal locking. It is true that these stars are often old, stable for long periods of time and by far the most common type of star, but I think we’re better off looking at brighter main sequence stars, such as spectral classes K, G or even F.
Several recent papers have pointed out that albedo effects on the planets of red dwarf stars may significantly expand the sizes of their habitable zones. See Joshi and Haberle, “Suppression of the water ice and snow albedo feedback on planets orbiting red dwarf stars and the subsequent widening of the habitable zone,” Astrobiology Vol. 12, No. 1 (23 Jan 2012); here’s the abstract. For Centauri Dreams‘ discussion on this, see M-Dwarfs: A New and Wider Habitable Zone.
These monographs suggest that on worlds with significant snow and ice cover, the effective albedo is much lower than snow and ice on Earth because frozen water absorbs more radiant heat at the red and infrared wavelengths emitted by red dwarfs than it does in the visual part of the spectrum as in Sol’s case. This effect would certainly extend the size of the habitable zone, but that would be dwarfed (no pun intended) by the much greater inverse square law effect (several orders of magnitude) of the much lower M-dwarf stellar luminosity.
Of the 53 known systems (66 stars) within 5 parsecs (16.3 ly) of the Sun, there are 48 red dwarfs (spectral class M) ranging from absolute magnitude 8.09 to 16.20 (an enormous range of luminosities!), and only 2 of them brighter than absolute magnitude 10.0. Absolute magnitude is the intrinsic brightness, how bright the star would appear if it were exactly 10 pc distant.
Keep in mind that magnitudes are exponential: A 5th magnitude star is 100 times brighter than one of 10th magnitude. Or alternatively, each magnitude is 2.512 times brighter than the next. The Sun’s absolute magnitude is 4.84, Barnard’s star, 13.23. Proxima Centauri is absolute magnitude 15.56. Although our own Sun is considered to be in the mid-range of stellar luminosities, it is still much brighter than most other stars. Red dwarfs may be very numerous, but they are very, very faint. All together, they don’t provide much habitable space.
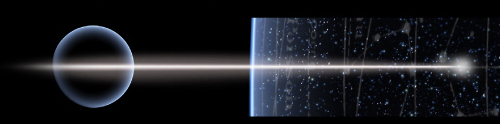
by Paul Gilster | Jan 1, 2021 | Uncategorized |
Alex Tolley’s essay on using beaming technology to reach the solar gravity focus (SGF) caught the eye of Jim Benford, who has been exploring the prospects for beamed sails for many years. Along with brother Greg, Jim did laboratory work at the Jet Propulsion Laboratory some 20 years ago to demonstrate the method, and in the years since has written extensively on the uses of beaming within the Solar System as well as on interstellar trajectories. But what kind of beam are we talking about? Benford, a plasma physicist and CEO of Microwave Sciences, has done recent work on a gravitational focus mission in connection with Breakthrough Starshot. He points to the maturity of microwave technology and the cost savings involved in using microwaves for a mission far faster than anything that has yet flown.
by James Benford
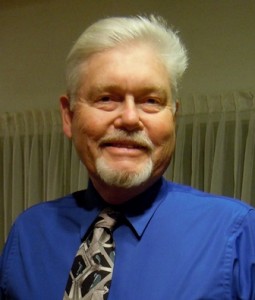
An intermediate destination for beamed energy interstellar probes, such as Starshot, is the Sun’s Inner Gravitational Focus (SGF). Alex Tolley suggests using Beamer technology for this mission. Gregory Matloff and I studied this approach in 2018 in work on the Starshot Project and published it [1]. This is a summary and update of that work.
The on-going Starshot technology development program will build a modular Beamer system that will incrementally achieve steadily higher launch speeds. As the Starshot technology develops, velocity regimes beyond anything available now will be attained. This will include flyby probes of the outer solar system planets and moons, exploration of the Kuiper belt objects and interstellar precursors to investigate beyond the heliopause. All these missions have the advantage of not requiring any deceleration as the objective is reached. Thus consideration of earlier missions and destinations nearer than the Centauri system is in order.
Here we consider a specific application of the basic Starshot concept, to fly a mission at 100 km/sec. We take sailcraft parameters from Parkin’s Starshot System Model, a thin-film circular photon sail with a mass of 4 grams, a payload of 1.5 grams, a diameter of 5 meters and a thickness of about 0.1 micron (0.2 g/m2, in the range of graphene) [2]. In order not to choose the system parameters arbitrarily, we use the Beamer cost optimization method developed by Benford [3], which minimizes the total system cost.
Why Cost Matters
The approach in our paper is to stipulate the key parameters; mass and velocity, then minimize the cost of the system. All other parameters, such as the sail diameter and, most importantly, the frequency of the Beamer are varied in order to minimize costs. Why does cost matter? These are very expensive systems: note that Starshot is designed/optimized to have a system cost below $10B. We showed SGF Beamer Systems can be in order of magnitude lower.
Economies of Scale
The costs include the decrease in unit cost of hardware with increasing production, economies of scale [3]. The components we’re modeling here, sources of microwave, mm-wave and laser beams, antennas and optics, must be produced in large quantities for the large scales of directed energy-driven sails. High-volume automated manufacturing would drive costs down.
Cost-Optimized Systems
Microwave Beamer cost is 580 M$. (Parameters are wavelength 0.03 m, frequency 10 GHz) parameters for 100 km/sec, 3 gram, 5-meter diameter sail, perfect reflectivity, 0.3m wavelength.) Microwave costs have reached true economies of scale and are now available in quantity at about 0.01 $/W and about 100 $/m2. Consequently, there is no need to extrapolate future microwave cost because present costs are low enough to use.
Millimeter-Wave Beamer cost is 2 B$. Thus far, millimeter-wave (wavelength 3mm, 100 GHz) devices at ~ 1 MW are available at $6/W and 10,000 $/m2. No large market has developed for millimeter-wave devices, so economies of scale have not been firmly established. We assume the learning curve of millimeter-wave tubes will be approximately that of similar tube devices, such as klystron, for which the learning curve is well established. At present the largest application for a megawatt-level millimeter-wave sources is the ITER fusion project, which requires hundreds of devices. An emerging near-term application for millimeter-wave technologies is for 5G Wi-Fi. Although the power levels will be low because of the short-range requirement, mass manufacture of millimeter-wave transmitters and apertures may enable substantial cost reductions to be realized in the next few decades.
Laser Beamer cost is >5.3 B$. Parkin estimates contemporary costs as at least $150/W and 1M $/m2. There are several options for the technology of the laser Beamer: from small mm-scale wafers at ~ 1 W power to larger ~500 W lasers with long coherence length (a key constraint in operating an array). Cost elements include emitters, optics and amplifiers. Lasers are being used for LIDAR in autonomous vehicles and at powers of 10-100 W, cost 100-$1000 $/W. At the higher figure, the Beamer would cost 23 B$!
The large number of sails needed to provide a useful image of an exoplanet means that we must take into consideration the cost of sails. Each sail will cost far less than the Beamer. We estimated the cost of such sails at ~1M$ each [1].
Technology Readiness and Feasibility
- State-of-the Art. Several practical factors favor microwave and millimeter waves over lasers, because they have practical advantages: Microwave equipment such as sources, anechoic rooms, antennas and diagnostics are commonly available than the emerging technology of high power lasers. That’s because microwave and millimeter wave sources, waveguide and supporting equipment, such as power supplies, are a developed industry. That means it is cheaper and faster to build systems. Lasers are developing fast, but at present are still expensive, and are produced in small numbers at slow rates.
- Efficiency. Microwaves are more efficient than lasers, typically 50-90%. Millimeter wave generation technologies now make it possible to generate wavelengths as short as 0.1 cm with relatively high efficiency (>40%). Laser efficiencies are ~40% now and have been slowly rising.
- Phased Arrays. Microwave phased arrays of transmitters and apertures are relatively easily done and are widely used, while phased arrays of laser beams, although possible in principle, subject to the coherence length constraint related above, are thus far little developed in practice. Work to date on laser phased arrays has been limited to small numbers of sources and modest power levels.
Desorption-Assisted Sail Missions
A different method that the JPL group has apparently not noticed is to use the desorption of various materials from the sail, ‘paints’, as it passes perihelion near the sun. That multiplies the utility of the solar sail technique substantially.
Thermal desorption consists in atoms, embedded in a substrate, that are liberated by heating, thus providing an additional thrust. Desorption can attain high specific impulse if low mass molecules or atoms are blown out of a lattice of material at high temperature.
Desorption of materials from hot sails in flight was observed in 2000 in microwave beam-driven carbon sail experiments I was conducting [4]. We found out that photon pressure could account for 3-30% of the observed acceleration, while the remainder came from desorption of embedded molecules.
After we understood what we were observing, my brother Gregory suggested it be used as a means of propulsion for sails [5,6]. The extraordinary potential of this sort of propulsion mechanism: if properly used, desorption could enhance thrust by orders of magnitude, shorten mission times.
Roman Kezerashvili and his fellow researchers have conducted detailed studies using desorption for solar sail missions to obtain high velocities [7]. Kezerashvili recently published a review article about this [8].
Conclusion
Therefore if we are to send probes to the SGF in this era, my calculations show that the lowest cost Beamer will be a microwave system. This will enable a transportation system within the Solar System that could be realized far sooner than laser arrays.
A solar sail augmented by desorption propulsion may give better performance for solar sail missions to the Sun’s Gravitational Focus.
If exoplanet imaging from the SGF is to be done soon, microwave or millimeter-wave beam systems could be built with existing technology now. Developing the phased array laser Beamer and driving the cost down to where larger arrays can be afforded will take decades. Similarly, it will take decades to conduct the test demonstrations required to prove the solar sail approach in the inner solar system. Advocates of both approaches should acknowledge these necessary timescales.
References
1. James Benford & Gregory Matloff, “Intermediate Beamers for Starshot: Probes to the Sun’s Inner Gravity Focus”, JBIS 72, 51-55, 2019.
2. Kevin Parkin, “The Breakthrough Starshot System Model”, Acta Astronautica 152 370, 2018.
3. J. Benford, “Starship Sails Propelled by Cost-Optimized Directed Energy”, JBIS 66 85, 2013.
4. James Benford et al., Flight and Spin of Microwave-Driven Sails, Final Report, Contract Number NAS8-99135, 2000. See also short version: “Flight and Spin of Microwave-driven Sails: First Experiments”, James Benford, Proc. Pulsed Power Plasma Science 2001, IEEE 01CH37251, 548, 2001.
5. Gregory Benford & James Benford “Desorption Assisted SunDiver Missions”, AIP Conf. Proc. 608, 462-469, 2002.
6. Gregory Benford, & James Benford, “Acceleration of Sails by Thermal Desorption of Coatings”, Acta Astronautica 56, 593-599, 2005.
7. Elena Ancona, Roman Ya. Kezerashvili, & Gregory L. Matloff, “Exploring the Kuiper Belt with sun-diving solar sails”, Acta Astronautica 160, 601-605 2019.
8. Elena Ancona & Roman Ya. Kezerashvili, “Extrasolar Space Exploration by a Solar Sail Accelerated via Thermal Desorption Of Coating”, Advances in Space Research 63 2021-2034, 2019.
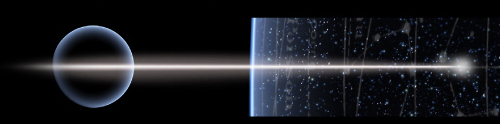
by Paul Gilster | Dec 25, 2020 | Uncategorized |
I want to send along best wishes for the season to all of you. Centauri Dreams started as a book and became a study guide for me as I tried to keep up with ongoing developments in deep space research. But turning the site into a community, which I did in 2005 by adding comments, has been what really made it go, as I’ve continued to learn from the discussions between readers, finding new resources and different insights I would never have achieved on my own. So thank all of you for this continuing gift, and may this holiday season be the prelude to great discoveries ahead.
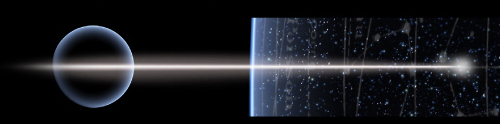