by Paul Gilster | Dec 19, 2007 | Asteroid and Comet Deflection |
Fifteen megatons of TNT would set off a blast a thousand times more powerful than the weapon used on Hiroshima. 2000 square kilometers of flattened pine forest near the Podkamennaya Tunguska River in central Siberia bear witness to what such a blast can do. That explosion occurred on June 30, 1908 [original typo said ‘2008,’ an obvious mistake!], and we’re learning more about it. The Tunguska event seems to have been an air blast occurring at an altitude between five and ten kilometers. The presumed cause: A small asteroid slamming into our atmosphere at speeds in the range of 15-30 kilometers per second.
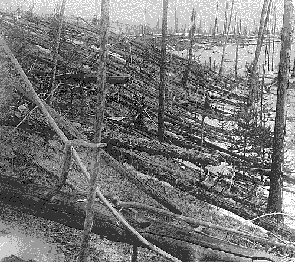
But just how big was the asteroid? I’ve seen estimates in the range of 50-100 meters in diameter, but we know surprisingly little about the object. No fragments exist. The effects of the fireball and blast wave are apparent (and there are eyewitness accounts of hot winds and shaking buildings), but there is no crater at the epicenter of the blast. We’re left to calculate the parameters of the object through its effect and its presumed composition and speed as it entered the atmosphere.
New work at Sandia National Laboratories suggests something that is worrisome indeed. I’m going to let Sandia’s Mark Boslough deliver the bad news:
“The asteroid that caused the extensive damage was much smaller than we had thought. That such a small object can do this kind of destruction suggests that smaller asteroids are something to consider. Their smaller size indicates such collisions are not as improbable as we had believed… We should be making more efforts at detecting the smaller ones than we have till now.”
The Sandia work uses a supercomputer to match the known facts with their probable cause. The devastation delivered by the Tunguska object slammed into the ground in the form of a high-temperature jet of expanding gas. The fireball turns out to be more efficient than we realized, creating a blast wave and thermal radiation at the surface stronger than would have been predicted simply by modeling an explosion at the assumed altitude. New models of the fireball show that the energy it transported to the surface is consistent with only a three to five megaton blast, much smaller than originally thought.
So we’re looking at a smaller asteroid to account for Tunguska, which should set off a few alarm bells. For planetary security, think small. Smaller asteroids approach the Earth more frequently than the larger variety. Are we doing enough to track down not just the large potential impactors but those capable of Tunguska-like events? The question is obviously rhetorical at a time when we’re still wrangling over keeping planetary radars like Arecibo’s alive so that they can play their role in such detections. To avoid another Tunguska, we’d better get smart about not just maintaining but ramping up the asteroid search.
by Paul Gilster | Dec 18, 2007 | Astrobiology and SETI |
Lately I’ve been thinking about cosmic killers, the kind of extinction events that could destroy an entire ecosphere and any civilization living within it. It’s a natural enough thought given our speculations about life elsewhere in the universe. Just how hostile a place is the Milky Way? We’re beginning to learn that planets are abundant around stars in our region of the disk, with the encouraging expectation that habitats for evolving lifeforms must be widespread. But maybe there are natural caps other than technological suicide that could end a civilization’s dreams.
You can’t help pondering this when you run into the recent news about a long duration gamma-ray burst (GRB) that took astronomers by surprise. GRBs are normally thought to flag the death of a massive star, but in this case the burst seems to come out of nowhere. What caused the event in a region of space where the nearest galaxy is 88,000 light years away? And no question about GRB 070125’s credentials. It was detected and corroborated last January and its location readily pinpointed in Gemini.
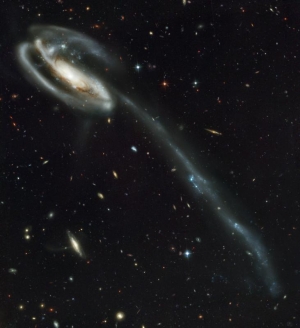
So did a massive star find its way out into the depths of intergalactic space? If so, it was an unusual one, for the kind of stars we’re talking about lead short lives and by necessity remain close to the clouds of gas and dust inside galaxies that gave them birth. Research now turns to the question of whether the star in question could have formed in the ‘tidal tail’ between two interacting galaxies. Such tails can be extraordinarily faint. Hubble may be able to tell us whether or not this is true, in which case the burst will have shown us a star-forming region we didn’t know was there.
Image: A recent galaxy collision produced the long tail in the Tadpole Galaxy. If GRB 070125 exploded in a similar tail, only Hubble could detect the tail. Credit: NASA, H. Ford, et al.
Of course, where gamma-ray bursts are concerned, the farther away, the better. But there are other interesting sources of trouble besides GRBs. Consider 3C321. The two galaxies orbiting each other that make up this celestial object made enough of a splash that my local newspaper picked up the story, an unusual addition for a normally astronomy-free venue. Both these galaxies, according to Chandra X-ray Observatory data, contain supermassive black holes, but the larger galaxy shows a jet that seems to be pointed right at the smaller. That could make things interesting indeed at the receiving end, as Dan Evans (Harvard-Smithsonian Center for Astrophysics) is quick to point out:
“We’ve seen many jets produced by black holes, but this is the first time we’ve seen one punch into another galaxy like we’re seeing here. This jet could be causing all sorts of problems for the smaller galaxy it is pummeling.”
Indeed. High-energy X-rays and gamma rays aren’t conducive to long lifetimes, with potentially lethal effects for the atmospheres of planets that happen to be in the way. The two galaxies are separated by a scant 20,000 light years, so the effect is about the same as if a jet from the Milky Way’s core illuminated our position in the Orion Arm. Which brings to mind disaster scenarios like that found in Richard Cowper’s wonderful Twilight of Briareus, where a future Earth is transformed by a supernova (not GRB) explosion, with results that awaken in humans a strange, latent potential (regulars here know of my admiration for Cowper).
That’s quite a benign result compared to what might happen with a full-bore hit from a nearby GRB or a major jet. Fortunately for life’s chances, alignments like that seen in 3C321 seem vanishingly rare. As for that unusual GRB in Gemini, the evidence says GRB 070125 took place some 9.4 billion years ago. We know all too little else. “Many Swift discoveries have left astronomers scratching their heads in befuddlement,” says Swift lead scientist Neil Gehrels (NASA GSFC). “But this discovery of a long GRB with no host galaxy is one of the most perplexing of all.”
More surprises are likely. Last December Nature reported on GRBs in a series of papers, focusing particularly on the interesting GRB 060614. This was an extremely long (102 seconds) burst from 1.6 billion light years away in the constellation Indus. No trace of a supernova could be found that would explain it. Another burst, GRB 060505, likewise left no supernova remnant. All of which leaves this observer thinking GRBs have much to teach us, particularly in terms of understanding the celestial events that give them birth. As for extinction events, GRBs do, at least, seem to be tightly beamed, good news for all but the unlucky.
by Paul Gilster | Dec 17, 2007 | Exoplanetary Science |
How long did it take for the planets in our Solar System to form? Much depends upon the surface density of the solar nebula protoplanetary disk, the gas and dust from which the planets emerged. And the problem with surface density — mass per area — in these settings is that it’s hard to observe with our current instrumentation. Looking at distant systems in the process of formation, we see mostly dust and miss larger objects.
Thus an estimate based on known factors is called into play. It produces the so-called minimum mass solar nebula. Using it, scientists can estimate solar nebula mass by starting with the rocky components of each planet, adding hydrogen and helium until the composition resembles that of the Sun. Spread that mass over the area of each planet’s orbit and you get disk masses that look like what we see in systems around other stars.
But there’s a problem. The low surface densities this model produces aren’t sufficient to allow the planets to form in a reasonable period of time. This is where Steve Desch (Arizona State University) goes to work. Here’s the problem he sees:
“I was thinking about planet formation and noticing that all the current models failed to predict how Jupiter could grow to its current size in the life time of the solar nebula. Given Jupiter’s composition and size, models predicted it would take many millions of years for it to form, and billions of years for Uranus and Neptune – but our solar system isn’t that old.”
Desch turned to a different model for considering the solar nebula. The ‘Nice model’ (named after the French city whose open markets — fabulous olives! — still have my head spinning) operates according to fundamentally different assumptions. For one thing, the model assumes the giant planets formed much closer together than their present position suggests, with Neptune forming at around 15 AU. Using this model to assume a much more tightly packed solar nebula, Desch was able to simulate a nebula with smooth variations in surface density with distance from the Sun, although that density falls off sharply at the outer edge.
In fact, using this model, all the planets could be accounted for, assuming you switched Uranus and Neptune in their places. Desch now thinks that for the first 650 million years of the Solar System, Neptune was closer to the Sun than Uranus. Planet formation, meanwhile, now seems to fit the kind of time restrictions imposed by the early nebula. Desch again:
“The surface density of the solar nebula isn’t what we originally thought – it is actually much higher – and this has implications for where we formed and for how fast planets grow. A higher surface density of the solar nebula means that Uranus and Neptune formed closer and faster, in only 10 million years instead of billions.”
Just as significant, Desch thinks he can explain why the solar nebula falls off so sharply in density at large distances from the Sun. The process at work is photoevaporation, with material at the outer edge of the disk being constantly removed because of the effect of ultraviolet radiation from nearby massive stars. The paper examining all this is Desch, “Mass Distribution and Planet Formation in the Solar Nebula,” Astrophysical Journal 671 (December 10, 2007), pp. 878-893 (abstract). Dr. Desch has also made the full text available via his Web site.
by Paul Gilster | Dec 15, 2007 | Outer Solar System |
We wrap up our look at the American Geophysical Union meeting last week in San Francisco with an update on Europa. An interesting point that William McKinnon (Washington University, St. Louis) made in a news briefing there has that what had been a belief that there is an ocean on Europa is now hardening into a certainty. The intriguing next step is to learn more about that ocean, and here things get tricky. Just how do you study an ocean on a distant world upon which you have yet to land?
Fortunately, magnetic variations around Europa as well as observations of how the moon flexes and deforms as it orbits Jupiter can tell us much. Thus the need for a Europa orbiter, a mission that could measure gravity and magnetic fields as well as determining surface composition. Another area of interest for such a spacecraft: Are there recent eruptions from this geologically young world? The search for hot spots from such events could get interesting, to judge from what Cassini found on Enceladus.
Complementing such work will be the use of radar sounding, which should give us a solid idea of how thick the blanket of ice over the ocean is. Donald Blankenship (University of Texas at Austin), who joined McKinnon in the briefing, noted that ice-penetrating radar should be able to locate liquid water both within and beneath the shell. “Free water within the icy shell,” Blankenship adds, “and its relationship to the underlying ocean will be a critical factor in determining the habitability of Europa.”
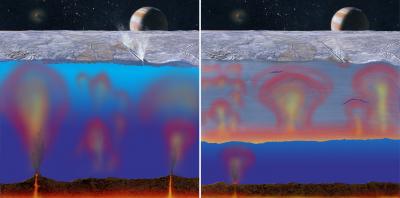
Image: An artist’s conception showing two possible cut-away views through Europa’s ice shell. In both heat escapes, possibly volcanically, from Europa’s rocky mantle and is carried upward by buoyant oceanic currents. If the heat from below is intense and the ice shell is thin enough (left), the ice shell can directly melt, causing what are called “chaos” on Europa, regions of what appear to be broken, rotated, and tilted ice blocks. On the other hand, if the ice shell is sufficiently thick (right), the less intense interior heat will be transferred to the warmer ice at the bottom of the shell, and additional heat is generated by tidal squeezing of the warmer ice. This warmer ice will slowly rise, flowing as glaciers do on Earth, and the slow but steady motion may also disrupt the extremely cold, brittle ice at the surface. Europa is no larger than Earth’s moon, and its internal heating stems from its eccentric orbit about Jupiter, seen in the distance. As tides raised by Jupiter in Europa’s ocean rise and fall, they may cause cracking, additional heating, and even venting of water vapor into the airless sky above Europa’s icy surface. Credit: Michael Carroll, courtesy NASA/JPL.
That issue of habitability is also more interesting given new models showing that radiation on Europa is perhaps up to two-thirds less powerful than previously thought, making the prospects for orbital spacecraft and landers a little less daunting. Moreover, reprocessing of Galileo data is telling us more about the surface of this frozen world, including the presence of carbon dioxide, which is most likely coming from the ocean below. Future orbital missions may well find compounds Galileo’s instrumentation was unable to catch.
This is an interesting point in itself. We’re collecting so much data in our recent missions that researchers can be overwhelmed with the inflow. Further analysis and reprocessing — in some cases years or decades after the fact — can tease out information that had hitherto been missed. Thus the infrastructure demand is clear: We can’t afford to lose even the most apparently insignificant datasets, a fact that would seem obvious but bears repeating given the challenge of examining old Pioneer data. In terms of both storage and format, future scientists have got to be able to recover anything a deep space probe can send.
So will we ever get some kind of vehicle able to penetrate the ice on Europa? The answer may depend on what a future Europa orbiter, when and if it is funded, finds. One project to keep an eye on as these things are debated is Stone Aerospace’s Endurance, an autonomous underwater robotic vehicle that will be deployed in Antarctica some time in 2008, testing approaches and procedures for studying a frozen ocean. The kind of biochemical maps Endurance makes may one day tell us whether anything lives on Jupiter’s most provocative moon.
by Paul Gilster | Dec 15, 2007 | Culture and Society |
Universe Today offers the latest Carnival of Space, which will have exoplanet watchers checking Steinn Sigurðsson’s Dynamics of Cats site for updates on CoRoT. Almost a year into its mission, the observatory has completed its first 150-day continuous examination of the same area of sky, and the results are, so rumor has it, quite interesting. I see that CNES (Centre National d’Études Spatiales) is now saying that ‘CoRoT will instigate a breakthrough in both of the fields of science that it applies to,’ making the upcoming publication of CoRoT papers eagerly to be awaited. And you have to love this statement: “”…CoRoT is discovering exo-planets at a rate only set by the available resources to follow up the detections.”