by Paul Gilster | Sep 30, 2010 | Exoplanetary Science |
What exactly does the word ‘habitable’ mean? The question comes to mind because of two things, the first being the media buzz over Gliese 581g, now widely described as the first potentially habitable planet we’ve found. The second is Paul Davies’ presentation yesterday at the International Astronautical Congress in Prague, where Davies was careful to differentiate between ‘habitable’ and ‘inhabited.’ More on the latter in a moment. Let’s look first at this outstanding find, two new planets in the Gliese 581 system discovered through the unflagging efforts of the Lick-Carnegie team.
A World in the Zone?
The beauty of Gl 581, of course, is not only that it has yielded a storehouse of planets (six known at present), but that these worlds are on nearly circular orbits, and several have caught our eye re habitability before. The current buzz seems a bit tamer than the one that greeted the announcement of Gl 581c, at the time thought to be capable of sustaining liquid water on its surface, although later research has shown that the planet is much more likely to be a Venus-like heat trap. Gl 581d, too, has its supporters, who argue that given the right atmospheric composition, temperatures there might be sufficient to sustain life.
But Gl 581g looks to be the most promising of the bunch, with a mass between three and four times that of Earth, a rocky world with enough gravity to hang onto an atmosphere and surface temperatures that average between -31 and -12 degrees Celsius. That’s cold, of course, but actual temperatures here are going to depend on where you happen to be, the planet being tidally locked to its star. I like what Stephen Vogt (UC-Santa Cruz) said at the press conference announcing the finding. Vogt talks about ‘eco-longitudes,’ meaning that on a tidally locked world like this, there will be temperature zones that follow the longitudes, from very hot at the center of the star-facing side to freezing on the dark side. Let me quote him briefly:
“If you are in the most comfortable place on this planet, which would be on the terminator, you would see the star sitting on the horizon. an eternal sunrise or sunset, depending on whether you are an optimist or a pessimist. Over billions of years, there would be stable zones where the ecosystem stays at the same temperature. You have ‘eco-longitudes,’ so that if you have evolved to like hot zones, you move a bit toward the star side, and if you like cold zones, you move toward the shadow side. And it stays like that more or less forever, so there are a lot of different niches for different kinds of life to evolve.”
The ‘goldilocks’ metaphor is being used all over the place to describe this planet’s orbital placement, a comparison Vogt was quick to exploit (this from a UC-Santa Cruz news release):
“We had planets on both sides of the habitable zone–one too hot and one too cold–and now we have one in the middle that’s just right… Any emerging life forms would have a wide range of stable climates to choose from and to evolve around, depending on their longitude.”
Tidal Lock and Open Questions
I’ll never get over the fascination of places like this, where the sun never moves in the sky and patterns of light and shadow are permanent, so that to move through what we consider a day-like cycle of light and dark would require physically moving along the surface of the planet. The terminator region between dark and light may be the most habitable region, though our modeling of atmospheres on such worlds is an ongoing thing, and it was only in the late 1990s that we began to realize, thanks to work by Manoj Joshi, Robert Haberle and team at NASA Ames, that a tidally locked planet might sustain regions stable enough to support life. Other questions, particularly that of solar flares on frequently active M-dwarfs, remain open.
Vogt talks about 238 observations with a precision of 1.6 meters per second being required for the radial velocity observations that found this world, and in the news conference, he pulled out a telling analogy to explain their precision. Use exquisitely sensitive calipers to measure a 6-inch ruler. Now stand the same ruler up on its end and measure it again. It will be infinitesimally shorter because it shrinks under its own weight, the effect of gravity. That tiniest of all changes is about on the order needed to find a Gl 581g amongst the data. It’s superb work, combining HIRES spectrometer (which Vogt designed) observations with published data from the Geneva group, and it is the result of fully eleven years of study of this interesting star.
The Nature of Habitability
But let’s get back to that question about habitability. First, Vogt is of the opinion that finding GL 581g this early in the planet hunt (with some 500 exoplanets now found) is a telling feat:
“If these [habitable worlds] are rare, we shouldn’t have found one so quickly and so nearby. The number of systems with potentially habitable planets is probably on the order of 10 or 20 percent, and when you multiply that by the hundreds of billions of stars in the Milky Way, that’s a large number. There could be tens of billions of these systems in our galaxy.”
Encouraging indeed. But I’m also reminded of Paul Davies’ comment yesterday in Prague. Davies was addressing the change in opinion in the scientific community, which has in the past fifty years moved to support the idea of extraterrestrial life. In the paper on which his presentation was based, Davies writes “…to profess belief in extraterrestrial life of any sort, let alone intelligent life, in the 1960s and 1970s, was tantamount to scientific suicide. One might as well have expressed a belief in fairies.” But all that has changed with new discoveries, leaving many scientists convinced of life’s ubiquity. Davies takes a more cautious view.
We might, for example, call a planet ‘habitable’ because it can support liquid water at the surface. But is this enough? Again from the Davies paper (and all the italics are his):
Water does in fact seem to be abundant in the solar system and beyond, so (it is reasoned) life should also be abundant. Unfortunately this simplistic reasoning confuses a necessary with a sufficient condition. To be sure, liquid water is necessary for life (at least as we know it), but it is far from sufficient. The reason life on Earth inhabits almost all aqueous niches is because Earth has a contiguous biosphere, and life has invaded those niches; it has not arisen there de novo.
Davies is concerned less about life’s ability to adapt to extreme conditions than about the likelihood of its formation in the first place. Indeed, this is the question that so confounds our pulling meaningful information from the Drake Equation, in his view. He goes on:
Another reason given for the current optimism about life beyond Earth is the dawning recognition that life can survive in a much wider range of physical conditions than was recognized hitherto, opening up the prospect for life on Mars, for example, and generally extending the definition of what constitutes an “earthlike” planet. But this at most amounts to a factor of two or three in favor of the odds for life. Set against that is the exponentially small probability that any given complex molecule will form by random assembly from a soup of building blocks. In short, habitability does not mean inhabited. It is natural that we should concentrate on the habitable planets in our search for life – by the “keys under the lamppost” principle -but at this stage we cannot put any level of confidence – none at all – on whether such a search will prove successful.
Cause to Celebrate Nonetheless
So there you are, a cautionary note in an otherwise celebratory morning (and Davies, of course, wasn’t aware of the Gl 581g news when he wrote this). But even as I understand Davies’ position, I have to say that my own mood is much more upbeat. We have much to learn, but what an achievement this discovery is, showing our capability of finding planets of near-Earth mass, planets likely to have atmospheres, and surface conditions that could sustain water in liquid form. Whatever really exists on Gl 581g, it’s a fact that this discovery points toward near-term discoveries of planets much more like our own, around G and K stars at distances where tidal lock is not an issue. We still have no ‘second Earth’ but it’s only a matter of years (perhaps even months) before we find one.
And let’s not forget the second planet in the discovery announcement. No question of habitability here, but we should note the existence of Gl 581f, at a minimum-mass seven times that of the Earth, and orbiting at 0.758 AU with a period of 433 days. Gl 581g is going to get the lion’s share of attention, but we’re moving into the era of characterizing entire planetary systems and that in itself is worthy of celebration. It will ultimately teach us much about planetary formation and help us refine the strategies we need to bag that first unquestionably ‘Earth-like’ planet.
The paper is Vogt, Butler et al., “The Lick-Carnegie Exoplanet Survey: A 3.1 M_Earth Planet in the Habitable Zone of the Nearby M3V Star Gliese 581,” accepted by The Astrophysical Journal and available as a preprint.
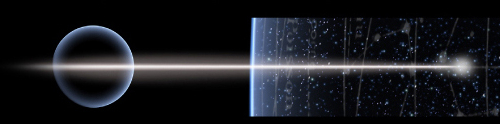
by Paul Gilster | Sep 29, 2010 | Astrobiology and SETI |
The ‘slow boat’ to Centauri concept we’ve discussed before in these pages envisions generation ships, vessels that take thousands of years to cross to their destination. And based on current thinking, that’s about the best we could manage with the propulsion systems currently in our inventory. Specifically, a solar sail making a close solar pass (a ‘sundiver’ maneuver) could get us up to 500 or 600 kilometers per second (0.002c), making a 2000-year journey to the nearest star possible. It’s hard to imagine under what circumstances such a mission might be launched.
But let’s think long-term, as Greg Matloff (New York City College of Technology) did in a session that just concluded at the International Astronautical Congress in Prague. Matloff, a solar sail expert and well known figure in the interstellar community, notes that when the Sun leaves the main sequence and becomes a red giant, its luminosity may have increased by a factor of a thousand. Imagine using that kind of star as the source of the photons for your solar sail and you’ve dropped the time for an interstellar transit down to centuries or even decades.
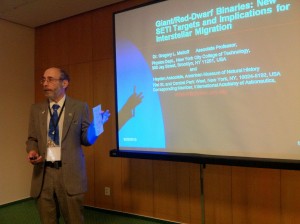
So let’s think about a civilization leaving the vicinity of a giant star, looking for a new home as their system is destroyed. They’ve got fast propulsion options by virtue of their star. The catch is, how do they slow down when they arrive? Traveling at the kind of velocities that would allow an interstellar crossing in decades, their photon sail would be all but useless for deceleration upon arrival at a main sequence star, meaning they’d have to supplement it with something like a magnetic sail. Or perhaps there’s another way, and one that has SETI implications.
Image: Greg Matloff begins his presentation this morning in Prague. Credit: Pat Galea.
After all, most stars are members of binary or multiple systems. Now we have an improved scenario: An advanced civilization targets a binary system composed of a giant and a widely separated red dwarf star. It uses the red giant’s intense luminosity for deceleration, then crosses to the planetary system of the red dwarf. We know that red dwarfs remain stable on the main sequence for as long as a trillion years, making building a new home there an attractive and long-term proposition.
We may find such thinking remote because we’re not all that concerned about our own Sun’s far future, with five billion years to go before it enters its giant phase. But if intelligent life is widespread in the universe, then a species evolving on planets circling stars older than our own or far more massive than our Sun may have already taken such a path. Matloff thus suggests that SETI take a look at red giant/red dwarf binaries within 100 light years from the Sun.
He then proceeds to go through a list of candidates, finding four possibilities: Alschain (Beta Aquilae), Gienah (Epsilon Cygni), Aldebaran (Alpha Tauri) and Theta Ursae Majoris [see note in the comments section]. Alschain’s red dwarf companion is roughly 150 AU from the star, while Gienah’s is at some 1700 AU. Aldebaran is just over 600 AU from its red dwarf companion, and Theta Ursae Majoris has an M6 red dwarf within 94 AU. All of these stars are northern hemisphere objects, so that for SETI purposes, the Allen Telescope Array should be able to conduct observations on them.
And our own future? Matloff’s figures show that at intervals of about 100,000 years, random motions cause a star to approach the Sun within two light years. In the paper his presentation is based on, he writes:
If the Sun?s luminosity will be at least twice its current level for 2 billion years between its late-main-sequence and planetary-nebulae phases, at least two stellar systems of the type considered here should closely approach the Sun. If we consider trips to destinations as far as 4 light years, we expect close approaches by 16 of these systems.
And actually, the number is higher, for Matloff goes on to show that most stars in the Sun’s vicinity are of roughly the same age. Five billion years from now, when and if a civilization remains in our system, many nearby stars will also have entered their red giant phase. Our remote posterity will, then, have plenty of red giant/red dwarf pairs to choose from, and thus planets to terraform and colonize in their habitable zones. “Terrestrial-derived life,” Matloff adds, “could have one or more planetary homes that will remain habitable for perhaps a trillion years.”
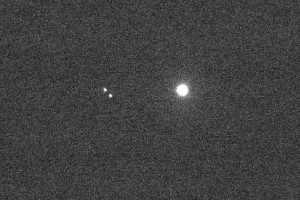
But back to SETI. Just as we have four red giant/red dwarf binaries to look at for SETI purposes, we might also consider widely separated white dwarf/red dwarf binaries. After all, a star’s red giant phase is finite, after which it becomes a white dwarf. Any civilization that used these methods to enter a planetary system around a red dwarf would survive to find itself in close proximity to the white dwarf remnant. And in fact, we do have one system like this close to the Sun. It’s Omicron-2 Eridani, 16.4 light years away, a K1 orange dwarf with a white dwarf that has an M4 dwarf companion of its own at 35 AU. The system is also called 40 Eridani.
Image: The triple star system 40 Eridani. The fainter stars are B and C. 40 Eri B is a white dwarf star, 40 Eri C is a red dwarf. Credit: RDP, Tulane University.
Ring any bells? 40 Eridani is considered by many fans to be the location of the planet Vulcan in Star Trek. Now wouldn’t it be a kick if a place like that yielded our first confirmed signal from an extraterrestrial civilization?
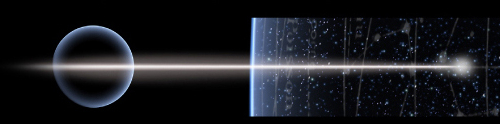
by Paul Gilster | Sep 28, 2010 | Missions |
Project Icarus, introduced to the IAA at last year’s Aosta conference, made quite a splash yesterday at the International Astronautical Congress in Prague, with four presentations by Icarus team members and related work on the FOCAL mission by Claudio Maccone. Icarus is the attempt to re-examine the Project Daedalus starship study of the 1970s in light of technological developments in the intervening years. A joint project between the British Interplanetary Society and the Tau Zero Foundation, it’s now in fully operational mode.
Fueling Up a Starship
There is much in these papers worth comment, but today I’ll home in on the issue of helium-3 and where to find it, presented yesterday and drawing on the work of Andreas Hein, Andreas Tziolas and Adam Crowl. Daedalus was envisioned as a fusion mission using deuterium and helium-3 as fuel, a reaction that has advantages over deuterium/tritium but one that has yet to be demonstrated in a working reactor. Assuming we do figure out how to light this reaction, we still have problems in that helium-3 has a very low abundance on Earth, which is why Project Daedalus’ planners came up with the idea of entering Jupiter’s atmosphere to collect it.
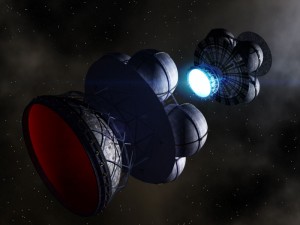
The idea is audacious, involving huge factories moving through Jupiter’s clouds and orbital ferries that would carry the precious cargo to Callisto, outside the worst of the planet’s radiation belts. Moreover, 128 such factories deployed over 20 years were needed to mine the needed 30,000 tons of helium-3. The Icarus designers note both the scale and the attendant problems of the idea, although uses of helium-3 fusion in Earth-based power generation and planetary defense could come into play to offer additional incentives to acquire helium-3 resources.
Image: The Daedalus starship at second stage firing. Credit: Adrian Mann.
But what about Jupiter itself? The strong radiation belts here rule it out for the Icarus team, which also eliminated Saturn because of its own radiation problems and high escape velocity. Uranus emerges as the planet of choice, with returning the processed helium-3 to orbit presenting perhaps the biggest technical hurdle. Double-walled hot-air balloons are chosen to keep the processing unit stable in the atmosphere, with a single-stage nuclear-thermal rocket emerging as the best solution for return to orbit. But the Icarus study is young, and the team is also considering tether concepts for atmospheric mining that do not involve braking and descent into the atmosphere at all.
The Outgoing Nuclear Option
Safety and political concerns would make a nuclear-thermal rocket unlikely for the outbound leg from Earth to Uranus, which the team thinks better handled in any case by nuclear electric propulsion, with aerodynamic braking or aerocapture used for orbital injection upon arrival. And we can’t rule out interesting solar sail possibilities. However, NEP remains the most attractive option to date. The paper on which Hein’s presentation was based lays out the benefits analysis and notes the high cost of gathering helium-3. What to do? Let me quote from the paper:
…we have to consider the consequences of the results for space exploration and an interstellar probe using D-He3 propulsion. For the annual rate of 1500 tonnes of He3 required for the Daedalus probe, it is reasonable to assume a civilization which has a much higher need for He3 for energy generation or interplanetary transportation. Taking current energy growth estimates, this will take many centuries. A possible solution to this problem would be a drastic reduction of the payload mass of the probe. A reduction by two orders of magnitudes would require an annual mining rate of 15 tonnes. Nevertheless, the course of the Icarus study will show, whether this kind of reduction of the probe size is feasible.
Thus we run into energy growth estimates again (see the discussion of Marc Millis’ paper on same in relation to the first launch of an interstellar probe). Reducing the payload mass helps, but we’re also looking to a civilization that has established a clear need for helium-3. The Daedalus design, of course, ran into constraints like this, but it falls to Icarus to take them to their logical conclusion, even as we note again that deuterium/helium-3 fusion has yet to be demonstrated. Must we wait for other fusion concepts and, possibly, a Uranus space elevator to supply the technologies that would fuel Icarus, pushing back its launch by centuries?
First Estimates and Their Uses
A search of the literature shows that deuterium/helium-3 fusion has been analyzed in terms of inertial confinement, electrostatic confinement and magnetic confinement, but the higher energy and temperature requirements are obstacles that seem to favor deuterium/tritium fusion in the short run. Hein examined whether deuterium/helium-3 could be competitive with the more researched deuterium/tritium alternative, coming to this conclusion:
A rough estimate shows that the maximum order of magnitude cost of Helium 3 must be between 1 – 10 Billion$ per ton, in order to be competitive. This is a very important result, because it will limit the allowed cost per ton of He3 obtained in our mining operation, if fusion reactor economics is the dominant factor.
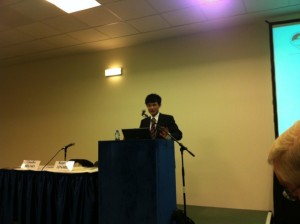
The technical and commercial feasibility of deuterium/helium-3 remains in question, because Hein’s numbers exceed the maximum cost. But if a workable fusion propulsion technology using helium-3 becomes available, then its uses within the Solar System would be clear, especially in terms of dealing with dangerous objects like Earth-crossing asteroids. A planetary defense grid could well take advantage of this kind of fusion, allowing the delivery of fast payloads to these objects in response to perceived threats. This coupled with possible Earth-based reactors could impel society in the helium-3 direction and would represent the best scenario for lowering costs.
Image: Andreas Hein giving his presentation at IAC 2010. Credit: Pat Galea.
If that scenario plays out, helium-3 mining could become a long-term strategic investment in technology and security. A system delivering helium-3 to the L2 Earth-Sun Lagrange point could thus generate political and financial support. All of this could reduce the cost of helium-3 to within acceptable limits, making deep space missions like Icarus more likely. How likely is all this to occur? Reports like these deliver first estimates and are not meant to be definitive, but they do sketch out the extraordinary challenges implicit in the idea of putting together a near-term interstellar mission.
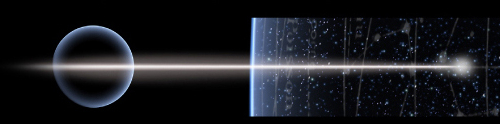
by Paul Gilster | Sep 27, 2010 | Missions |
The International Astronautical Congress is in full swing in Prague today, with regular updates flowing over #IAC2010 on Twitter and the first session of interstellar import now in progress as I write this. It’s a session on interstellar precursor missions that includes, in addition to Ralph McNutt (JHU/APL) on the impact of the Voyager and IBEX missions, a series of papers from the Project Icarus team ranging from helium-3 mining to communications via the gravitational lens of both the Sun and the target star (no specific target has yet been chosen for Icarus).
Claudio Maccone will be summarizing where we stand with the FOCAL mission, envisioned as the first attempt to exploit gravitational lensing for astronomical observations. But I’ll turn today to Marc Millis, who will wrap up the precursor session with a discussion of the first interstellar missions and their dependence on things we can measure, such as energy. The notion here is to look at the energy required for an interstellar mission and to weigh this against predictions of when those energy levels will be accessible and available to be used for space purposes.
Energy Needs Drive Spaceflight
How to get a handle on energy growth trends? Millis uses annual data on the world’s energy production from 1980 to 2007, calculating the ratio of each year’s energy production to the preceding year, then finding the average and standard deviation of all 27 of these years. How soon until Earth becomes a Kardashev Type I civilization — one capable of mastering all the energy reaching the Earth from the Sun? Acknowledging the wide span of uncertainty in the result, Millis pegs the earliest year this could occur as 2209, with a nominal date of 2390 and a latest date of 6498. A constant growth rate is assumed, which balances depletion of natural resources against unforeseen advances in new energy sources, leaving growth rates relatively stable.
Fascinating as they are in their own right, I won’t go through all the numbers (I’ll link to the paper when it becomes available online). But note the key factors here, which are the total amount of energy produced by our species and the proportion of that energy devoted to spaceflight. For the latter, Millis compares the annual Space Shuttle launch rate against the total annual energy consumed by the United States, finding that the maximum ratio of Shuttle propulsion energy to total US energy consumed occurred in the year 1985, equaling 1.3 x 10-6. The average ratio over the years 1981 to 2007 is 5.5 x 10-7. Millis then takes the maximum ratio (over an order of magnitude greater than the average ratio) to calculate the earliest opportunity for future missions. What he calls the Space Devotion Ratio is thus 1.3 x 10-6.
The Alpha Centauri Calculation
When could we launch a 104 kilogram interstellar probe to Alpha Centauri based on these calculations? Assume 75 years as the maximum travel time that might be acceptable to mission scientists and assume a rendezvous rather than a flyby mission, acknowledging the need to acquire substantial amounts of data at the destination. Millis extrapolates from existing deep space probes to arrive at a putative mass, adding the needed margins to ensure survival over a 75-year transit and the substantial communications overheard to relay information to Earth.
As to propulsion options, Millis works with two possibilities, the first being an ideal case that assumes 100% conversion of stored energy into kinetic energy of the vehicle (think ‘idealized beam propulsion’ or even some kind of space drive), the second being an advanced rocket with an exhaust velocity of 0.03c. We thus wind up with two sets of figures, again based on energy availability. Millis then converts the propulsion energy figures into equivalent world energy values, using the Space Devotion Ratio he first calculated earlier for US space involvement.
The result: The earliest launch for a 75-year probe is 2247, with a nominal date of 2463. This assumes idealized propulsion; i.e., a breakthrough technology like a space drive. Fall back on advanced rocket concepts and the energy requirements are much higher, with the nominal launch date of the probe now becoming 2566, the earliest possible date being 2301.
Strategies for Interstellar Research
The play in the numbers is huge, the uncertainty in the results caused by the wide span in possible energy production growth rates. Interestingly, Millis’ finding that the earliest interstellar mission will not be possible for two centuries coincides with earlier estimates from Bryce Cassenti and Freeman Dyson based on economic and technological projections. We can, obviously, adjust the numbers based on our projections of technological growth, and as with any projection, sudden changes to world economic patterns would be a substantial wild card.
But Millis argues that in the absence of a single technological solution, it would be premature to focus on specific propulsion options to the exclusion of other, more theoretical alternatives. For that matter, it would be foolish to be inhibited by the ‘incessant obsolescence’ postulate (a term that Millis himself coined), noting that earlier missions may well be overtaken by faster ones launched at a later date. Instead, what he calls ‘cycles of short-term, affordable investigations’ targeting key questions whose answers we can hope to find today are the best way to proceed. And that means continuing our investigations of everything from the already operational solar sails to technologies that today seem impossible, such as travel faster than the speed of light.
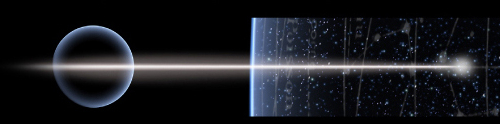
by Paul Gilster | Sep 24, 2010 | Astrobiology and SETI |
The European Planetary Science Congress ends today in Rome even as scientists and engineers on the astronautical side of things head for Prague, where the International Astronautical Congress convenes on Monday. I’ll be keeping an eye on events in Prague and wishing I could join the gathering of Tau Zero practitioners that will be taking place there — Marc Millis will be presenting four papers, and many of the Project Icarus team members are also making the journey, so we should be getting regular updates on matters interstellar.
Nor do I want to neglect the Royal Society meeting on extraterrestrial life, coming up early in October in Buckinghamshire in the UK. Emails from James Benford (Microwave Sciences) and Richard Carrigan (Fermilab) tell me both will be speaking at the session, which reminds me that it was way back in April that I promised more on Carrigan’s notions of interstellar ‘archaeology,’ a form of SETI that makes no assumptions about the originating civilization. It’s time to honor that pledge by looking at the kind of artifacts an advanced civilization might create that could be detectable. Today I want to focus on an extreme possibility, a civilization that spans its galaxy.
Kardashev Type III and Its Traces
What would happen if a true galaxy-spanning civilization went to work on the kind of astroengineering it would be capable of? We call this a Kardashev Type III civilization, one that could exploit the power resources of an entire galaxy, and my assumption has always been that such a culture would be blindingly obvious, its projects so vast that our astronomers would be able to detect them by noting anomalies that could hardly be natural occurences. Imagine, for example, a galactic culture that encloses each individual star in a Dyson sphere.
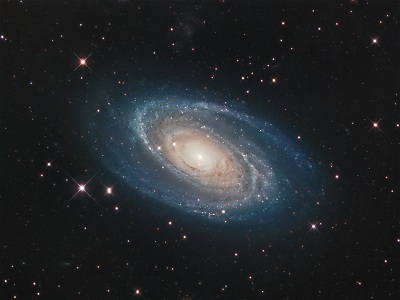
A Dyson sphere or ‘shell’ would absorb all of the visible light from a star, re-radiating stellar energy at infrared wavelengths. A Dyson ‘ring’ would use planetary materials that would mask only part of the star’s light. Dick Carrigan used a list of interesting infrared sources from the Infrared Astronomy Satellite (IRAS) in his own search, coming up with no strong Dyson sphere candidates. But Dyson spheres remain interesting, vastly increasing the habitable area around a star. What would a Type III civilization do with technologies that could create Dyson spheres not only in one place but across the galaxy?
Image: M81, a spiral galaxy in Ursa Major. A ‘wavefront’ Dyson sphere culture might spread across such a galaxy, causing stars to drop out of visible light entirely, one by one, to be detected in the infrared. Credit and copyright: Giovanni Benintende.
Whatever the answer, you would think it would be clearly noticeable. Freeman Dyson himself has said that “…a type III (Kardashev civilization) in our own galaxy would change the appearance of the sky so drastically that it could hardly have escaped our attention.” In a recent paper, Carrigan also quotes James Annis, who has studied anomalous galaxies in quest of signs of a Type III civilization. Annis’ view: “It is quite clear that the Galaxy itself has not transformed into a type III civilization based on starlight, nor have M31 or M33, our two large neighbors.” But Carrigan wonders whether we should take these statements as definitive:
…what would happen for a civilization on its way to becoming a type III civilization, a type II.5 civilization so to say? If it was busily turning stars into Dyson spheres the civilization could create a “Fermi bubble” or void in the visible light from a patch of the galaxy with a corresponding upturn in the emission of infrared light. This bubble would grow following the lines of a suggestion attributed to Fermi… that patient space travelers moving at 1/1000 to 1/100 of the speed of light could span a galaxy in one to ten million years. Here “Fermi bubble” is used rather than “Fermi void”, in part because the latter is also a term in solid state physics and also because such a region would only be a visible light void, not a matter void.
A Wavefront of Dyson Spheres
This is long-term thinking in the richest sense. We currently have spacecraft moving out of our Solar System at a speed of roughly 0.0001 of the speed of light. If we stay well within physics as currently understood, we can expend 100 times the energy to raise that velocity from 42 kilometers per second to 400 kilometers per second, in the neighborhood of 10-3 c. Now we’re talking galactic travel times on the order of a hundred million years and less, half the time the Sun takes to circle the center of the galaxy. A patient, long-lived civilization could, as Carrigan notes, envelop a galaxy on a time-scale comparable to or shorter than the rotation period of the galaxy.
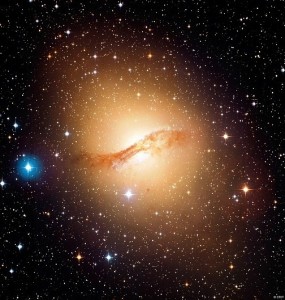
Turning stars into Dyson spheres as it went, such a culture should leave vast Fermi ‘bubbles’ whose infrared signature would flag their existence. But detection might still elude us. Carrigan points out that we see M51, the Whirlpool galaxy, face-on at a distance of 30 million light years. We can say with some confidence that we see no unexplained voids larger than about five percent of M51’s area, but any void features below this level would be hard to identify because of spiral galaxy structure. Elliptical galaxies might be better places, says James Annis, to look for Fermi bubbles, because they display little structure, and potential voids should be far more pronounced.
Image: The elliptical galaxy Centaurus A. Are ellipticals the best place to search for Type III civilizations? Credit: NASA/CFHT.
Even so, separating artificial structure from natural phenomena is not easy. I was fascinated to learn from Carrigan’s paper that we have identified several relatively dark dwarf galaxies, including Virgo HI21, the Ursa Major dwarf spheroidal UMa dSph and others. Anyone making the case that these objects show evidence of Dyson sphere activity runs into more plausible natural explanations. Virgo HI21, for example, may be showing the effects of tidal shredding. These so-called ‘dark galaxies’ demand a thorough understanding of star populations and types and exquisitely precise radio astronomy measurements to help fix the rotation rate. What might have seemed an easy search for a Dyson infrared signature becomes exceedingly complicated.
When Annis ran his search for anomalous galaxies, he took advantage of the fact that galactic optical brightness can be measured against the maximum rotation velocity and radius of the galaxy. The relationship between these factors is relatively consistent, allowing the scientist to look for sources below the normal trend line in a sample of 137 galaxies. Annis found no candidate galaxies in this admittedly small sample, but the work allowed him to set a limit on dark galaxies below one percent of the population. Larger-scale searches will doubtless be attempted one day.
The Uses of Interstellar Archaeology
Even though we have only begun to tune up our methods for detecting an extraterrestrial civilization, there is much we can learn. Our existing SETI methods and near-term observations of exoplanets may be the best chance at finding a genuine life signature elsewhere, but we should keep the possibility of a truly advanced civilization in mind. Says Carrigan:
Breaking up a planet to build a Dyson sphere completely transcends the will, ability and long term dedication of contemporary civilization. There is not even a mildly plausible technique for stellar engineering. Surprisingly the step from a Dyson sphere to a galaxy filled with Dyson spheres only involves a strengthened capability for space travel. On the time scale of Dyson Sphere construction this type of relatively slow space travel can be discussed even now. To recapitulate, SETI and the search for planetary signatures of life and intelligence currently appear to be the most accessible signatures. For Dyson spheres and stellar engineering work should mostly be directed toward investigating engineering techniques and limitations.
I come back around to the premise behind interstellar archaeology, that unlike conventional SETI it does not require a civilization to have any intention of contacting us. There are numerous ways to proceed, involving the kind of Dyson sphere search Carrigan has himself conducted within our own galaxy, or looking at planetary atmospheres in hopes of finding not only biosignatures but the markers of an advanced industrial or post-industrial culture. As we continue the SETI hunt, keeping in mind how planetary change or deliberate decisions to expand into the galaxy could leave visible traces allows us to hunt for things advanced intelligence might do.
How many civilizations in our galaxy, for example, have already faced the end of their main sequence star’s lifetime? If the number is high, it may be that we can find evidence of their response in the form of planetary or stellar engineering, making stars of this description interesting targets for future searches. In any case, our model of SETI is changing as not only our technologies but our assumptions become more sophisticated, leaving us to ponder a universe in which the need for expansion or simple survival may have left its own detectable history.
The paper is Carrigan, “Starry Messages: Searching for Signatures of Interstellar Archaeology” (preprint). The Annis paper is “Placing a limit on star-fed Kardashev type III civilisations,” JBIS 52, pp.33-36 (1999).
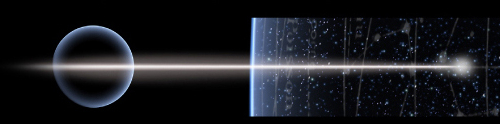