by Paul Gilster | Jan 31, 2020 | Deep Sky Astronomy & Telescopes |
Farewell to Spitzer after more than 16 years of infrared observations of the universe. We’ve recently looked at the observatory’s accomplishments (see Looking Back at the Spitzer Space Telescope), but I want to run the photo below to celebrate the team that managed it.
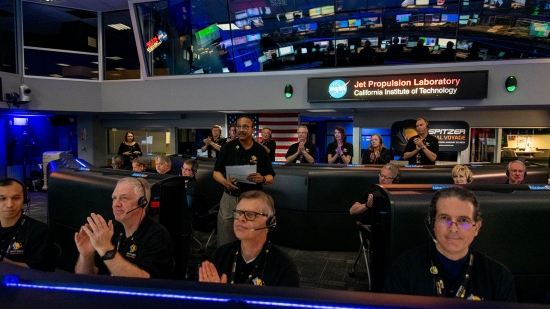
Image: Spitzer Project Manager Joseph Hunt stands in Mission Control at NASA’s Jet Propulsion Laboratory in Pasadena, California, on Jan. 30, 2020, declaring the spacecraft decommissioned and the Spitzer mission concluded. Credit: NASA/JPL-Caltech.
Meanwhile, we have the good news that the European Space Agency’s CHEOPS (CHaracterising ExOPlanet Satellite), which was launched from Kourou (French Guiana) on December 18, has completed its early orbit phase, involving instrument tests and calibration, and has now opened its telescope cover, exposing the focal plane to starlight.
The space observatory carries a 95-cm long baffle that shields its telescope from stray light and minimizes light contamination from sources like the nearby Earth (CHEOPS is in a 700 kilometer heliosynchronous orbit, passing over any given point on Earth’s surface at the same local time). What has been removed is the baffle cover — ESA likens it to the lens cap of a camera — which had protected the instrument from dust and bright light during the initial phases of in-orbit commissioning. The procedure was considered mission-critical, and its successful completion means the telescope cover is now permanently locked in the open position.
Francesco Ratti is a CHEOPS instrument engineer for ESA:
“The opening mechanism is known to be extremely reliable, as it was extensively tested on the ground and already flown on previous space missions, but it was still quite a nerve-wracking moment to witness, and we are all very excited now that the telescope has opened its eye to the Universe.”
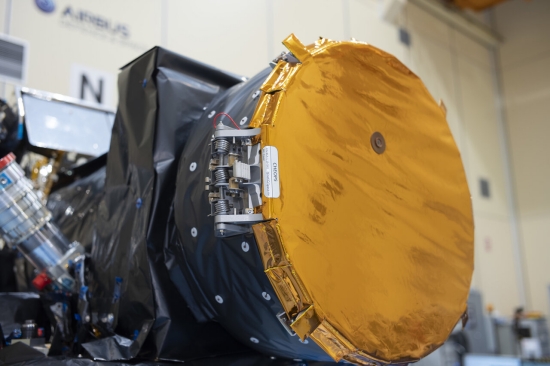
Image: The telescope baffle cover of the Cheops satellite, pictured here during spacecraft testings in the cleanroom at Airbus Defence and Space Spain, Madrid, protected the mission’s science instrument from dust and bright light during testing, launch and the early phases of in-orbit commissioning. The cover lid consists of an aluminium frame covered with copper-coloured, multi-layer insulation (MLI) sheeting. The spring-loaded hinge mechanism that connects the cover lid to the baffle is clearly visible in the foreground of the image. On the opposite side of the baffle (not visible in this photo), a locking mechanism held the cover closed. Credit: ESA.
Bear in mind that 80 percent of the observing time on CHEOPS is set aside for a program defined by the science team, but the remaining 20 percent is available to the astronomical community through ESA’s guest observer program, so proposals can be submitted for peer review in a competitive selection process. The observatory will study bright stars already known to host planets, using transit techniques to investigate their physical and chemical properties. For more, see CHEOPS Enters the Game, a Centauri Dreams post from late December.
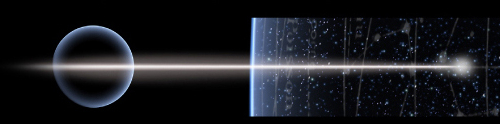
by Paul Gilster | Jan 30, 2020 | Missions |
When one of our Voyagers experiences a blip of any kind, it gets my attention. It’s not like we have any other options outside the heliosphere right now.
Both Voyagers have fault protection software that allows the spacecraft to protect themselves if problematic situations occur. And a problem did indeed surface aboard Voyager 2 on January 25, when there seems to have been a delay in the onboard execution of commands for a scheduled maneuver. The latter was a 360 degree rotation to be executed as a way of calibrating the craft’s magnetic field instrument, and the result of the delay was that two systems that consume power at relatively high levels were operating at the same time.
Not a good idea. Right now, with power dwindling inexorably, the Voyager missions are both dominated by power management. Hence the shutdown of Voyager 2’s science instruments to make up for the power deficit, as reported by the Voyager team on Twitter:
The Voyager team was able to turn off one of the high power systems on January 28, and has now turned the science instruments back on, although data acquisition has not resumed yet. What’s needed is a review of spacecraft status as a check to ensure that returning to normal operations is warranted. What I want to focus on here is the distance this diagnostic work is being conducted at, about 18.5 billion kilometers. Bear in mind that one way communications require 17 hours, with another 17 hours for the response.
Thus 34 hours are required between sending a command and finding out whether it has had the desired effect on the spacecraft. We’re dealing with 1970s technology here, a fact that emphasizes the role that spacecraft autonomy will increasingly play as we push into the immediate interstellar neighborhood, when such operations will need to be handled aboard the spacecraft from start to finish. It’s a tribute to the quality of the Voyager design that we are still able to make the craft function given communications delays that were never anticipated.
We’re also working with two spacecraft whose power supply is heading for exhaustion. The radioisotope thermoelectric generator (RTG) that powers up the Voyagers turns heat from radioactive decay into electricity, but such decay reduces Voyager 2’s power budget by about 4 watts per year. We’ve looked before in these pages at the balancing act this requires of controllers, who last year turned off the primary heater for the Voyager 2 cosmic ray subsystem to compensate for the decrease in power, although the instrument is still in operation.
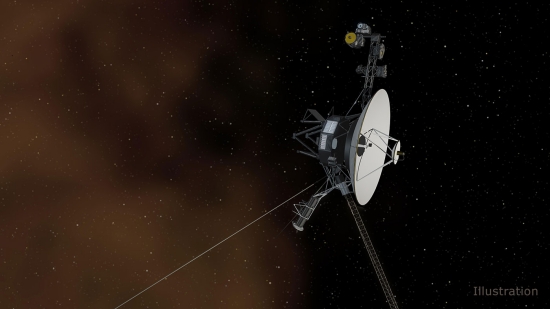
Image: This artist’s concept depicts NASA’s Voyager 1 spacecraft entering interstellar space, or the space between stars. Interstellar space is dominated by the plasma, or ionized gas, that was ejected by the death of nearby giant stars millions of years ago. The environment inside our solar bubble is dominated by the plasma exhausted by our Sun, known as the solar wind. Credit: NASA/JPL-Caltech.
Imagine trying to keep all these balls in the air at the same time, and then factor in the need to manage the temperature of the various systems, which can be manipulated through heaters or excess heat from the various instruments and systems onboard. The critical computer system, radio transmitter and receiver electronics and other instruments would quickly cool to mere tens of degrees above absolute zero without heat management, and now, with the interplay of instruments being shut down, power savings have to be weighed against temperature.
We’re in what NASA calls the Voyager Interstellar Mission, and we have scant time remaining, despite the heroic efforts of controllers, to continue receiving data before the science instruments have to be turned off in their entirety. But for now our first interstellar craft, even if not remotely designed for that purpose, are still communicating and capable of sending data.
Keeping Voyager alive is a tale worth telling and will one day produce a book of its own. For now, though, I recommend Jim Bell’s The Interstellar Age and Stephen Pyne’s Voyager: Seeking Newer Worlds in the Third Great Age of Discovery. Bell slants more toward science and engineering, while Pyne opts for context and philosophy. Someone in the Voyager interstellar team is bound to produce the next book, which will doubtless become a testament to making missions achieve the seemingly impossible.
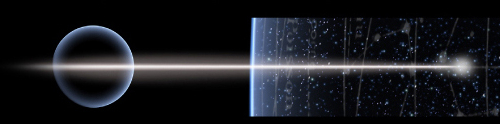
by Paul Gilster | Jan 29, 2020 | Asteroid and Comet Deflection |
Asteroids are objects of obvious scientific interest, not only for their intrinsic properties but also our need to understand how we can change their motion in space in case one looks like it will come dangerously close to Earth in the future. OSIRIS-REx is extracting all kinds of valuable data from asteroid 101955 Bennu, but we should also keep in mind that Bennu itself is a potentially hazardous object, with a small chance (1-in-2700, according to current estimates) of striking the Earth between 2175 and 2199. Thus the second ‘S’ in OSIRIS, which stands for ‘security’, and is all about measuring the factors that affect the object’s trajectory.
When we get samples from Bennu, we’ll have a better idea about the asteroid’s chemistry and morphology, useful for understanding the early Solar System as well as assessing how hazardous such an object is. But we need to know more, which is where NASA’s Double Asteroid Redirection Test (DART) mission comes in. Here the purpose is planetary defense from the start, for DART will demonstrate how a kinetic impactor can change the motion of an asteroid in space. The target is a binary near-Earth asteroid called (65803) Didymos.
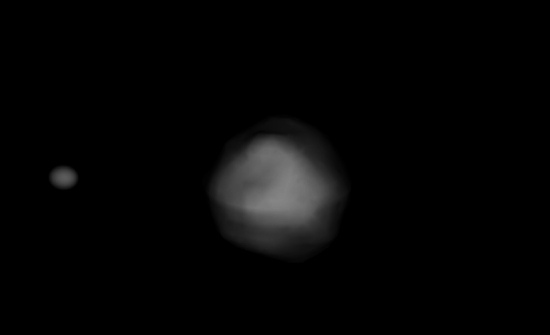
Image: Simulated image of the Didymos system, derived from photometric lightcurve and radar data. The primary body is about 780 meters in diameter and the moonlet is approximately 160 meters in size. They are separated by just over a kilometer. The primary body rotates once every 2.26 hours while the tidally locked moonlet revolves about the primary once every 11.9 hours. Almost one sixth of the known near-Earth asteroid (NEA) population are binary or multiple-body systems. Credit: Naidu et al., AIDA Workshop, 2016.
DART will carry an imaging instrument called DRACO (Didymos Reconnaissance & Asteroid Camera for OpNav), which is based on the now familiar LORRI high-resolution imager that flew on New Horizons, and will use roll-out solar arrays (each 8.6 meters by 2.3 meters) and a NEXT-C ion engine for propulsion. The plan is simplicity itself: DART will crash into the Didymos moonlet at 6.6 kilometers per second, which should change the moonlet’s orbital speed around the main body by a fraction of one percent, and the orbital period by several minutes.
Flying with DART will be LICIA, the Light Italian CubeSat for Imaging of Asteroid, which will observe the impact ejecta in the early phase of crater formation following the impact. The dynamic changes DART’s impact produces will be measured partly by what LICIA learns about the fallback ejecta on both asteroids and the subsequent Hera observations of unweathered fresh material on the two objects. NASA describes LICIA this way:
The LICIA Cube is a 6U CubeSat provided by the Italian Space Agency. It will be carried along with DART to Didymos and released approximately 2 days before the DART impact. LICIA Cube will perform a separation maneuver to follow behind DART and return images of the impact, the ejecta plume, and the resultant crater as it flies by. It will also image the opposite hemisphere from the impact. LICIA Cube is 3-axis stabilized and has a propulsion capability of 56 m/s. The onboard imager has a 7.6 cm aperture, F/5.2 telescope, and an IFOV of 2.9 arcsec/pixel.
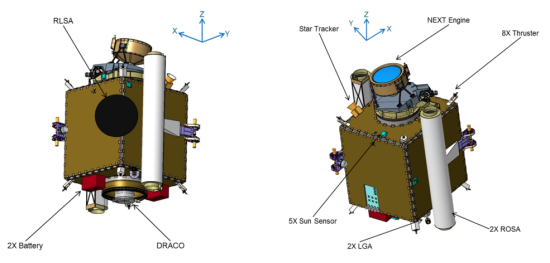
Image: Two different views of the DART spacecraft. The DRACO (Didymos Reconnaissance & Asteroid Camera for OpNav) imaging instrument is based on the LORRI high-resolution imager from New Horizons. The left view also shows the Radial Line Slot Array (RLSA) antenna with the ROSAs (Roll-Out Solar Arrays) rolled up. The view on the right shows a clearer view of the NEXT-C ion engine. Credit: NASA.
The European Space Agency’s Hera mission — powered by solar arrays with a hydrazine propulsion system — is to be the follow-up, making a post-impact survey using high-resolution visual, laser and radio science to map what will be the smallest asteroid yet visited by spacecraft. The DART collision is scheduled for 2022, with immediate results probably hidden by an expected dust cloud. Hera will investigate the asteroid impact crater and surrounding surface in 2026, allowing scientists to refine their numerical models of the impact process. All of this works toward building a deflection technique for planetary defense.
Earth-based observations of the Didymos system gathered during its close approach in February-May 2017 were analyzed at a workshop in Prague in 2018, allowing constraints to be placed on the strength of the YORP effect, which results from uneven heating on the surface that can alter the object’s spin state. Further observations will tighten these constraints, making the effects of the DART impact easier to separate from the pre-impact state of the system.
A key Hera role in all this will be to measure Didymos’ mass, which will help scientists calculate the efficiency of the impact momentum transfer once we’ve also measured the change in the small moon’s orbital period. Thus the two missions will result in accurate modeling of the response to the impact as well as the likely internal structure of the asteroid. Hera will be carrying two six-unit cubesats of its own to provide spectral measurements of the surface of both asteroids, with a Cubesat called APEX (Asteroid Prospection Explorer) actually landing on one of them. A second CubeSat (Juventas) will measure the gravitational field and internal structure of the small moon, doing a low-frequency radar survey of the asteroid interior.
The DART launch window opens in late July of 2021, with launch aboard a SpaceX Falcon 9, with intercept of the Didymos moonlet in late September of 2022, when the system is about 11 million kilometers from Earth. Earth-based telescopes and planetary radar will be able to measure the effects of the impact to back up the findings of the spacecraft on the scene. The results should be small but highly useful in giving us data on how impacts affect asteroids of this size, with the added benefit of enhancing international cooperation on a matter of global importance.
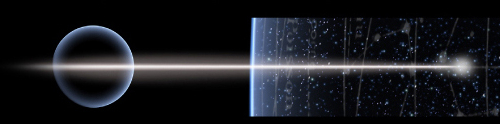
by Paul Gilster | Jan 28, 2020 | Asteroid and Comet Deflection |
The latest operations of the OSIRIS-REx spacecraft at asteroid Bennu remind me how powerful a wave we’ve unleashed in the coupling of robotics and ever more capable spacecraft components. We’re not exactly at the stage of ‘routine’ asteroid missions, but Hayabusa2 and OSIRIS-REx when seen in the context of upcoming missions like NASA’s DART experiment and the European Space Agency’s Hera are part of our renaissance of this class of object, with results beneficial to science but also practically useful in terms of future impact mitigation. More on DART and Hera tomorrow.
Small objects have plenty to say about our future in space, and I haven’t even mentioned Lucy, which will be studying multiple Jupiter trojans, or the Psyche mission targeting what may be the exposed core of a planetary embryo, or for that matter, the remarkably successful Dawn, which unlocked so many mysteries at Vesta and Ceres. It goes without saying that having an operational spacecraft in the Kuiper Belt is likewise a sign that we are getting pretty good at doing robotic exploration even as we continue to wrestle with the human role on the Moon and Mars.
OSIRIS-REx (Origins, Spectral Interpretation, Resource Identification, Security-Regolith Explorer) has now completed a 620 meter flyover of the site called ‘Nightingale’ on its target asteroid as part of the mission’s analysis of the primary sample collection site. To make this happen, the spacecraft left a 1.2 kilometer home orbit and performed an 11-hour transit over the asteroid, accumulating data about the 16-meter wide sample site and returning to safe orbit.
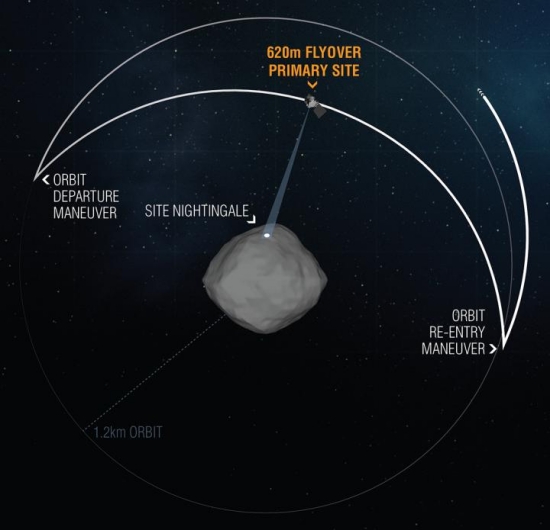
Image: During the OSIRIS-REx Reconnaissance B flyover of primary sample collection site Nightingale, the spacecraft left its safe-home orbit to pass over the sample site at an altitude of 0.4 miles (620 m). The pass, which took 11 hours, gave the spacecraft’s onboard instruments the opportunity to take the closest-ever science observations of the sample site. Credit: NASA/Goddard/University of Arizona.
The spacecraft has been compiling a Natural Feature Tracking image catalog by way of mapping the tiniest details among the boulders and craters of the landing site. The OSIRIS-REx team is also studying observations from the spacecraft’s Thermal Emissions Spectrometer (OTES), the OSIRIS-REx Visual and InfraRed Spectrometer (OVIRS), the OSIRIS-REx Laser Altimeter (OLA), and the MapCam color imager.
So there’s a lot happening at Bennu, including an upcoming flyover, scheduled for February 11, of the backup sample site, which has been given the name ‘Osprey.’ Further flybys in March (Nightingale) and May (Osprey) will take OSIRIS-REx even closer to the surface as the spacecraft goes into Reconnaissance C phase, operating at 250 meters. Assuming all goes well, a multi-hour sampling maneuver will begin in August, using the spacecraft’s robotic arm and sampler head to make contact with the asteroid. We will eventually gain between 60 and 2000 grams of surface material, with return to Earth scheduled for September of 2023.
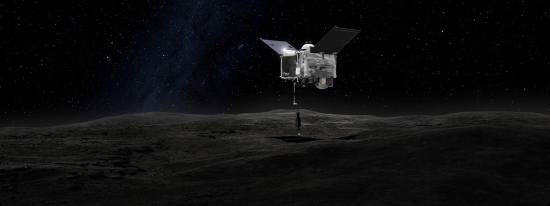
Image: This artist’s concept shows OSIRIS-REx contacting asteroid Bennu with its sample return instrument. Credit: NASA’s Goddard Space Flight Center.
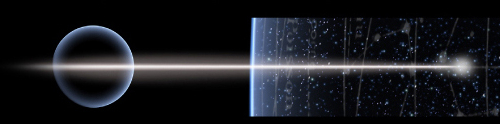
by Paul Gilster | Jan 27, 2020 | Deep Sky Astronomy & Telescopes |
Cataclysmic variable stars (CVs) are binary phenomena, usually consisting of a white dwarf that is accreting material out of a nearby companion star. As you would imagine, a wide range of CVs in various stages of accretion and subsequent outburst can be detected. When the accretion disk around the white dwarf becomes unstable, we get what is known as a dwarf nova (DN), and in systems with orbital periods less than two hours, there can be much more violent outbursts, feeding off orbital resonances in the orbit of the two stars.
Now we have a newly discovered cataclysmic variable (KSN:BS-C11a) in an interesting configuration, a white dwarf apparently feeding off a brown dwarf companion that is about 10 times less massive. The ‘super-outburst’ from the dwarf nova turned up in data from the decommissioned Kepler Space Telescope. Grad student Ryan Ridden-Harper (Australian National Observatory), lead author of the paper on this work, likes to refer to this cataclysmic variable as ‘a vampire star system.’ Whatever we call it, the level of activity here is noteworthy:
“The incredible data from Kepler reveals a 30-day period during which the dwarf nova rapidly became 1,600 times brighter before dimming quickly and gradually returning to its normal brightness. The spike in brightness was caused by material stripped from the brown dwarf that’s being coiled around the white dwarf in a disk. That disk reached up to 11,700 degrees Celsius at the peak of the super-outburst.”
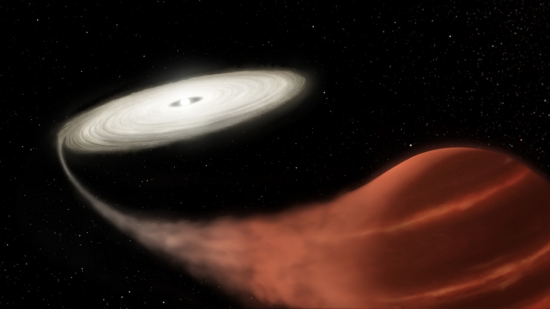
Image: An artist’s impression of a star ‘feeding’ off a nearby brown dwarf. Credit: NASA and L. Hustak (STScI)
Still unexplained is the slow rise in brightness that preceded the outburst. Ridden-Harper has been working with colleagues at ANU as well as the Space Telescope Science Institute (STScI) and the University of Notre Dame. What catches my eye about the discovery of this bright transient is the data mining that turned it up. The team had been searching for new transients in the K2 and Kepler campaigns in a project known as K2: Background Survey. The idea here is that each science target in the data is accompanied by background pixels that have been observed at high cadence, meaning a short time period before re-observing the same target.
These background pixels, the authors note, can contain transient signals that have thus far gone undetected. The paper describes the K2: Background Survey as:
…a systematic search for transients in K2/Kepler background pixels. K2:BS independently analyses each pixel to detect abnormal behaviour. This is done by searching for pixels that rise above a brightness threshold set from the median brightness and standard deviation through a campaign. Telescope motion presents a challenge in candidate detection as science targets may drift into background pixel, triggering false events. False triggers are screened by vetting of events that last < 1 day, chosen for candidates with the 6 hourly telescope resets. Coincident pixels that pass the vetting procedure are grouped into an event mask. All candidate events are checked against the NASA/IPAC Extragalactic Database (NED) 1 and the SIMBAD database (Wenger et al. 2000) to identify potential hosts.
As to the parameters of the system, the brown dwarf orbits the white dwarf every 83 minutes at a distance of approximately 400,000 kilometers, close enough to allow the rapid growth of the accretion disk as material spirals from the brown dwarf inward toward the host star. The Kepler cadence of 30 minutes turns out to have been the key to making these observations, which are particularly useful because only about 100 such dwarf novae systems have been catalogued.
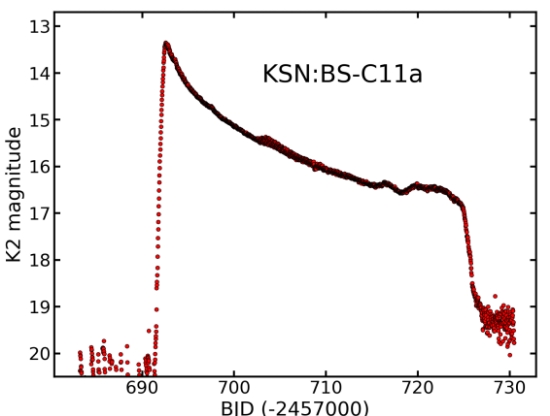
Image: This is Figure 3 from the paper. Caption: The K2/Kepler light curve of the transient KSN:BS-C11a shown with the 30-minute cadence. The time axis is shown in barycentric Julian days and the flux has been converted to Kepler magnitudes. Credit: Ridden-Harper et al.
Thus Kepler/K2 keeps on producing good science, in this case finding a transient whose rarity stems partially from the years or decades such a system can spend between outbursts. We shouldn’t be too surprised that Kepler data can produce such results — after all, the telescope was designed to study the transits of planets across the face of a star, making it ideal for spotting any objects that brighten or dim over time — but now we can see the potential for detecting other rare transient events both in archival data and data from ongoing missions like TESS.
The paper is Harper et al., “Discovery of a new WZ Sagittae-type cataclysmic variable in the Kepler/K2 data,” Monthly Notices of the Royal Astronomical Society,” Vol. 490, Issue 4 pp. 5551-5559 (abstract).
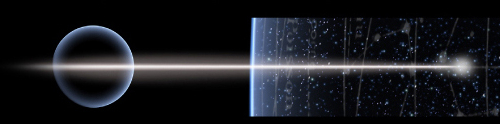