by Paul Gilster | Sep 29, 2021 | Exoplanetary Science |
Hot Jupiters (notice I’ve finally stopped putting the term into quotation marks) were the obvious early planets to detect, even if no one had any idea whether such things existed. I suppose you could say Greg Matloff knew, at least to the point that he helped Buzz Aldrin and John Barnes come up with a plot scenario involving a planet that fit the description in their novel Encounter with Tiber (Grand Central, 1996), which was getting published just as the hot Jupiter 51 Pegasi b was being discovered. Otto Struve evidently predicted the existence of gas giants close to their star as far back as 1952, but it’s certainly true that planets like this weren’t in the mainstream of astronomical thinking when 51 Pegasi b popped up.
Selection effect works wonders, and it makes sense that radial velocity methods would bear first fruit with a large planet working its gravitational effects on the star it orbits closely. Today, using transits, gravitational microlensing, astrometry and even direct imaging, we’re uncovering a much more representative sample of what’s out there, and hot Jupiters are somewhere around 1 percent of the catalog. Similarly rare, I’m sure, are the planets classed as ultra-short period worlds (USP), though they’re much smaller, with radii in the range of 2 Earth radii and periods of less than a day. We now have evidence for somewhere around 100 of these planets.
Two recently discovered examples are the planet candidates TOI-1634b and TOI-1685b, both of them TESS catches and subsequently examined by the Subaru Telescope (Mauna Kea, Hawai?i), supplemented with data from other observatories. An international team of astronomers have been at work on this duo, led by Teruyuki Hirano (University for Advanced Studies, Tokyo). The planet candidates are in Perseus, the first about 114 light years away; the second is 122 light years out. Both have now been confirmed to be rocky super-Earths (and I’m no longer going to put that term in quotation marks either). Both are likewise USPs, with ultra-tight orbits of less than 24 hours.
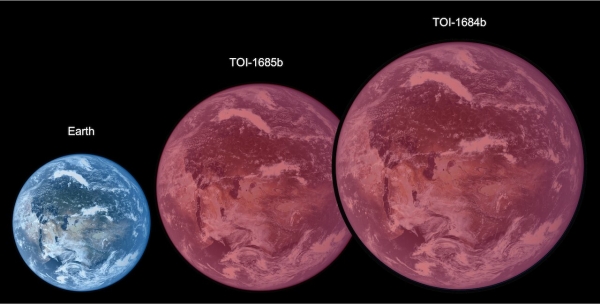
Image: Artist’s conceptual image showing the sizes of the planets observed in this study. The radius of TOI-1634b is 1.5 times larger than Earth’s radius and TOI-1685b is 1.8 times larger [note: the planets are mislabeled in the image]. The planets would appear red, due to the light from the red dwarf stars they orbit. Credit: Astrobiology Center.
As you’ll note from the caption above, the Subaru work (using the InfraRed Doppler — IRD — spectrograph mounted on the telescope) has been able to measure the masses of these transiting worlds. Here the chief interest is the fact that both lack a primordial hydrogen-helium atmosphere, which may or may not be the result of their proximity to their host stars.
Are there secondary atmospheres here, made up of gases released from within the planets? It’s an interesting speculation, as examining their constituents would tell us a lot about how these planets formed. The origins of USPs have been discussed in the literature in terms of inward migration, their highly circularized orbits the result of tidal interactions. There has been some suggestion that they represent remnant cores from hot Jupiters whose atmospheres have dissipated, but their hosts stars have different metallicity values than hot Jupiter host stars.
TOI-1634b and TOI-1685b, both of them orbiting M-dwarfs and relatively close to the Earth, may help us move forward in probing these issues, because to date there are few well characterized planets in the USP category; in fact, only the red dwarfs LTT 3780 and GJ 1252 have USP planets whose masses have been precisely measured. The authors note that TESS has been deeply involved in the hunt for more USPs, with 151 candidates announced as of February of 2021. 31 of these are known to orbit M-dwarfs.
The authors drew data from instrumentation ranging from Subaru itself to the MuSCAT imager at the Okayama Astro-Complex in Japan, the TCS telescope at Teide Observatory in Spain, the Gemini North telescope and Keck Observatory on Mauna Kea. Thus we’re examining data from ground-based transit photometry to high-resolution imaging, reconnaissance spectroscopy and radial velocity measurements, refining the orbital periods of these planets by more than an order of magnitude. Their future utility in atmospheric studies is clear. From the paper:
TOI-1634b is one of the largest and most massive USP planets having an Earth-like composition, and therefore, would become a benchmark target to study the formation and evolution history of massive USP planets. Both planets are listed among the best suitable targets for future atmospheric studies of small rocky planets by emission spectroscopy thanks to the brightness of the host stars, which encourages future characterizations using large aperture telescopes including JWST. Although small USP planets (< 2 R?) are likely to have lost the primordial atmospheres dominated by H2 and He, one may be able to probe and constrain the secondary atmosphere formed via the outgassing from the planet interior.
The paper is Hirano et al, “Two Bright M Dwarfs Hosting Ultra-Short-Period Super-Earths with Earth-like Compositions,” The Astronomical Journal Vol. 162, No. 4 (23 September, 2021). Abstract / Preprint.
by Paul Gilster | Sep 28, 2021 | Exoplanetary Science |
The gradual accretion of material within a protoplanetary disk should, in conventional models, allow us to go all the way from dust grains to planetesimals to planets. But a new way of examining the latter parts of this process has emerged at the University of Arizona Lunar and Planetary Laboratory in Tucson. There, in a research effort led by Erik Asphaug, a revised model of planetary accretion has been developed that looks at collisions between large objects and distinguishes between ‘hit-and-run’ events and accretionary mergers.
The issue is germane not just for planet formation, but also for the appearance of our Moon, which the researchers treat in a separate paper to extend the model for early Earth and Venus interactions that appears in the first. In the Earth/Venus analysis, an impact might be a glancing blow that, given the gravitational well produced by the Sun, could cause a surviving large part of an Earth-impactor (the authors call this a ‘runner’) to move inward and subsequently collide with Venus. So we’re not talking about impacts alone, but about impact ‘chains.’ The implications of this multi-impact theory on planet composition may be profound.
Alexandre Emsenhuber (now at Ludwig Maximilian University, Munich) is lead author of the paper on Earth/Venus interactions, pointing to the different impact scenarios for Earth and Venus:
“The prevailing idea has been that it doesn’t really matter if planets collide and don’t merge right away, because they are going to run into each other again at some point and merge then. But that is not what we find. We find they end up more frequently becoming part of Venus, instead of returning back to Earth. It’s easier to go from Earth to Venus than the other way around.”
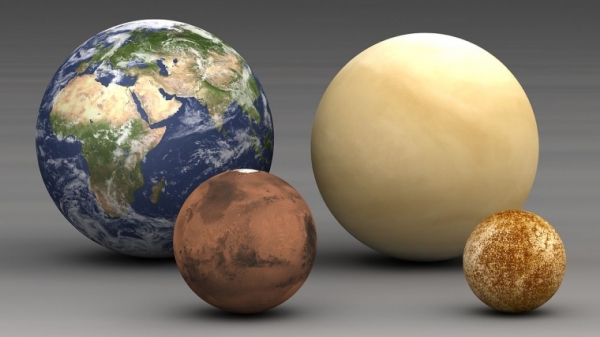
Image: The terrestrial planets of the inner solar system, shown to scale. According to ‘late stage accretion’ theory, Mars and Mercury (front left and right) are what’s left of an original population of colliding embryos, and Venus and Earth grew in a series of giant impacts. New research focuses on the preponderance of hit-and-run collisions in giant impacts, and shows that proto-Earth would have served as a ‘vanguard’, slowing down planet-sized bodies in hit-and-runs. But it is proto-Venus, more often than not, that ultimately accretes them, meaning it was easier for Venus to acquire bodies from the outer solar system. Credit: Lsmpascal – Wikimedia Commons.
This work draws on a 2019 analysis by the same authors that first examined hit-and-run collisions and subsequent mergers of the two bodies. The authors point out that most simulations of this stage of planetary evolution assume perfect mergers for all impacts that are not completely catastrophic. Reflecting on this, they write:
Emsenhuber & Asphaug (2019a, hereafter Paper I) showed that this is not generally the case. They studied the fate of the runner following hit-and-runs into proto-Earths at 1 au, for thousands of geometries, and found that, contrary to expectation, only about half the time (depending on the runner’s egress velocity, which depends on the impact velocity and angle) do they return to collide again with proto-Earth. When they do, the return collision happens on a timescale of thousands to millions of years.
That work — fully treated in the first of the papers cited below — also revealed that the majority of the runners that did not return to the forming Earth would be likely to collide with Venus, given the assumption of their current masses and orbits. Those runners that did return would show an impact velocity in the second collision similar to the egress velocity after the first hit and run, thus slower than the original impact because of momentum loss. Follow-on collisions, then, are likely to be slow.
So we have a scenario in which the Earth takes repeated hits and spins off many impactors toward the inner system as they fall deeper into the Sun’s gravity well rather than eventually assimilating them itself. It’s an interesting notion given that, while Earth and Venus (so-called ‘sister planets’) have similar mass and density, Venus is nonetheless in a distinctly different state, its rotation retrograde compared to other planets, with a single rotation taking 243 days. There are also no moons at Venus. Do impacts during formation account for the differences?
To put the thesis to the test, the scientists built predictive models from 3D simulations of such impacts, drawing on machine learning techniques. They simulated terrestrial planet evolution over the course of 100 million years, calculating both hit-and-run collisions and those in which the impactor merged with the object struck.
The simulations explore the dynamical evolutions of remnants of hit-and-run collisions until the impactor is finally accreted or ejected.The different scenarios, says Asphaug, portray a sharply different formation history for the two worlds:
“In our view, Earth would have accreted most of its material from collisions that were head-on hits, or else slower than those experienced by Venus. Collisions into the Earth that were more oblique and higher velocity would have preferentially ended up on Venus…. We find that most giant impacts, even relatively ‘slow’ ones, are hit-and-runs. This means that for two planets to merge, you usually first have to slow them down in a hit-and-run collision. To think of giant impacts, for instance the formation of the moon, as a singular event is probably wrong. More likely it took two collisions in a row.”
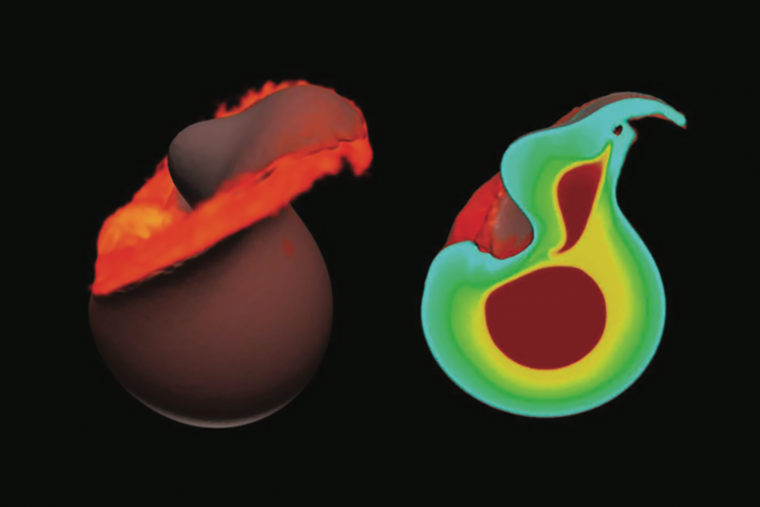
Image: The Moon is thought to be the aftermath of a giant impact. According to a new theory, there were two giant impacts in a row, separated by about 1 million years, involving a Mars-sized ‘Theia’ and proto-Earth. In this image, the proposed hit-and-run collision is simulated in 3D, shown about an hour after impact. A cut-away view shows the iron cores. Theia (or most of it) barely escapes, so a follow-on collision is likely. Credit: A. Emsenhuber/University of Bern/University of Munich.
Earth’s impact history thus has a telling influence on planetary composition. From the paper:
…if the terrestrial planets formed in multiple giant impacts, then Venus is significantly more likely than Earth to have accreted a massive outer solar system body during the late stage of planet formation. Earth, by contrast, has no terrestrial planet beyond its orbit to act as a vanguard. Mars is about the same mass as the late-stage projectiles…, 0.1 M?, and thus relatively inconsequential in terms of slowing them down through hit-and-run, so Earth has to do it on its own.
The late stage of terrestrial planet evolution in our own Solar System thus may hinge on how each world dealt with these impact runners. One thing that emphatically emerges from the work is that, according to these simulations, the terrestrial planets were hardly isolated during this period. Hit-and-run objects strike one planet, then the other, the probability of the impacts factored into the simulation via relative velocity and orbital configuration choices in the analysis.
In this study, Earth slows down projectiles, but accretes no more than half of them itself. Venus becomes a sink for these objects, retaining the majority of them in all simulations after their encounter with Earth as the slowed velocity of the runner allows for subsequent accretion. This would naturally lead to differences in composition between Venus and Earth and would account for differences in everything from Venus’ spin state, its formation (or lack of it) of moons, to its core-mantle dynamics. The authors promise a follow-up paper exploring these issues.
The papers are Emsenhuber et al., “Collision Chains among the Terrestrial Planets. II. An Asymmetry between Earth and Venus,” Planetary Science Journal Vol. 2, No. 5 (23 September, 2021), 199 (full text). The second paper is Asphaug et al., “Collision Chains among the Terrestrial Planets. III. Formation of the Moon,” Planetary Science Journal Vol. 2, No. 5 (23 September, 2021), 200 (full text)
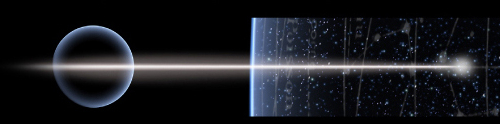
by Paul Gilster | Sep 24, 2021 | Exoplanetary Science |
A ‘hot Saturn’ with a difference, that’s WASP-127b. Although it’s 525 light years away, we’ve learned a surprising amount about the planet’s atmosphere. Details come via the ongoing Europlanet Science Congress 2021, now being held virtually for pandemic reasons, at which Romain Allart (iREx/Université de Montréal and Université de Genève) spoke this week.
WASP-127b is quite an unusual planet with or without cloud cover. It’s orbiting its star in a scant four days, amped up by stellar irradiation levels 600 times what the Earth receives from the Sun. That would, the researcher points out, produce temperatures in the range of 1100 degrees Celsius (over 1370 Kelvin). The result of all these factors is a world with a fifth the mass of Jupiter actually inflating into a radius 1.3 larger than Jupiter. The word in vogue among astrophysicists for a planet like this seems to be ‘fluffy,’ which pretty much describes it.
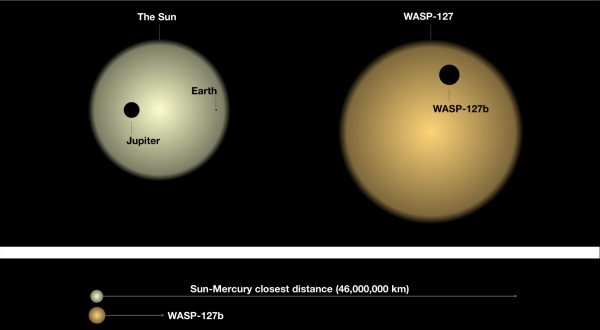
Image: WASP-127b compared with planets of our Solar System. Credits: David Ehrenreich/Université de Genève, Romain Allart/Université de Montréal.
The work on WASP-127b’s atmosphere involves infrared observations from Hubble combined with data from the extraordinary ESPRESSO spectrograph at the European Southern Observatory’s Very Large Telescope in Chile. The method at the VLT is transmission spectroscopy, analyzing the light of the star during a transit as it passes through the planet’s atmosphere. Allart pointed out to the virtual Europlanet Science Congress attendees that surprises were in store:
“First, as found before in this type of planet, we detected the presence of sodium, but at a much lower altitude than we were expecting. Second, there were strong water vapour signals in the infrared but none at all at visible wavelengths. This implies that water-vapour at lower levels is being screened by clouds that are opaque at visible wavelengths but transparent in the infrared. We don’t yet know the composition of the clouds, except that they are not composed of water droplets like on Earth. We are also puzzled about why the sodium is found in an unexpected place on this planet. Future studies will help us understand not only more about the atmospheric structure, but about WASP-127b, which is proving to be a fascinating place.”
The zone within the atmosphere that the scientists were able to probe with their combined datasets proved to be clouds in an atmospheric layer with a pressure ranging between 0.3 and 0.5 millibars. We also know that this is a planet in a retrograde orbit, something Allart says is unexpected for a hot Saturn in a stellar system that is thought to be a whopping 10 billion years old. If things have not settled down by this time (and the planet orbits as well in a different plane than equatorial), it may point to the presence of an unseen planetary companion.
Is planetary migration a possibility here? From the paper:
Our best-fit model shows that the old star WASP-127 is a slow rotator while its planet has a misaligned retrograde orbit (a view of the system is represented in Fig. 4). We also note that the WASP-127 system does not fit in the known dichotomy of hot exoplanets. Winn et al. (2010); Albrecht et al. (2012) have reported that stars with Teff below 6250 K have aligned systems which is not the case of WASP-127b (Teff = 5842 ± 14 K). One possible scenario is that WASP-127b remained trapped in a Kozai resonance with an outer companion for billions of years, and only recently migrated close to its star (see e.g. the case of GJ436b, Bourrier et al. (2018)). An in depth analysis of the system dynamic is beyond the scope of the present study.
Image: This is Figure 4 from the paper. Caption: Fig. 4: View of the star planet system at the ingress transit. The stellar disk velocity has a color gradient from blue to red for negative to positive stellar surface velocity. The stellar rotation axis is shown as the black top arrow. The planet is represented by the black disk and occults first the red part of the star and then the blue part, following its misaligned orbit shown in green. The green arrow represents the orbital axis. Credit: Allart et al.
So we’ve reached the point where we can tell the difference between cloudy worlds and their cloud-free counterparts by measuring water content, acquiring data that also reveals the pressure of the cloud deck from which the measurements are taken. It’s early days in this kind of analysis, but we can assume that these methods will work their way into future surveys on planetary atmospheres. Understanding atmospheric structure and composition is a path into the formation history and evolution of any exoplanets. Beginning with planets in extreme conditions, we can look forward to future surveys as these techniques are applied to smaller worlds.
The paper is Allart et al., “WASP-127b: a misaligned planet with a partly cloudy atmosphere and tenuous sodium signature seen by ESPRESSO,” Astronomy & Astrophysics Vol. 644 (December, 2020), A155 (abstract / preprint).
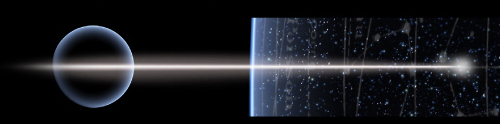
by Paul Gilster | Sep 23, 2021 | Asteroid and Comet Deflection |
What makes the asteroid 16 Psyche interesting is that it may well be the exposed core of a planet from the early days of Solar System formation, a nickel-iron conglomeration that normally would lie well below a surface mantle and crust. It’s also an M-class asteroid, a category of which it is the largest known sample. These are mostly made of nickel-iron and thought to be fragmented cores, though many have a composition that has not yet been determined.
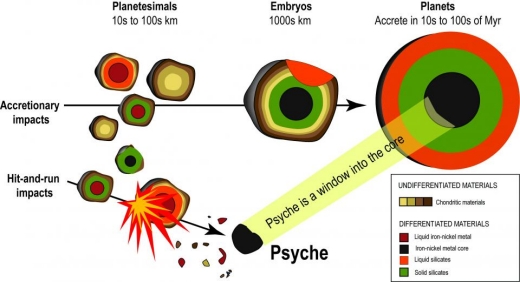
Image: Deep within the terrestrial planets, including Earth, scientists infer the presence of metallic cores, but these lie unreachably far below the planets’ rocky mantles and crusts. Because we cannot see or measure Earth’s core directly, asteroid Psyche offers a unique window into the violent history of collisions and accretion that created the terrestrial planets. Credit: University of Arizona.
M-class asteroids have been imaged before — the Rosetta spacecraft imaged the non-metallic 21 Lutetia in 2010, and 216 Kleopatra has been imaged by ES0’s 3.6 meter telescope at La Silla as well as Arecibo — but now we’ll see one from close orbit.
For Psyche will be visited by a spacecraft of the same name, slated for launch in 2022 via Falcon Heavy, with arrival in 2026 after a 3.5-year cruise under solar electric propulsion. A single Mars flyby will occur along the way. The plan is to spend 21 months in orbit around the asteroid. You’ll recall that this mission will be testing NASA’s DSOC package (Deep Space Optical Communication), using laser techniques to communicate with Earth, See Deep Space Network: A Laser Communications Future for more on the DSOC and its capabilities.
The Psyche spacecraft’s Hall thrusters, using solar arrays as the power source, will be used beyond lunar orbit for the first time in this mission. The propellant is xenon, a neutral gas that will be accelerated and expelled from the spacecraft by electromagnetic fields after being ionized, producing a now familiar blue beam. The spacecraft will carry 922 kilograms of xenon, enough to run the Hall thrusters for years without exhausting available fuel supplies. NASA engineers estimate that chemical methods would require about five times that amount of propellant to achieve the same mission.
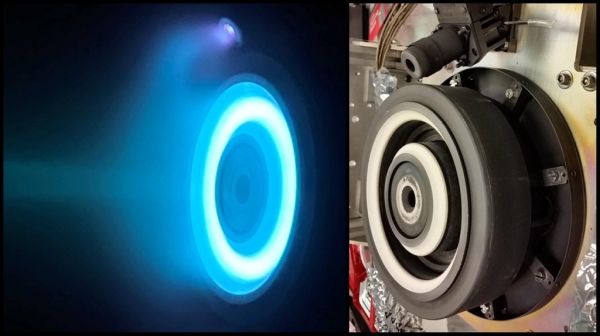
Image: The photo on the left captures an operating electric Hall thruster identical to those that will propel NASA’s Psyche spacecraft, which is set to launch in August 2022 and travel to the main asteroid belt between Mars and Jupiter. The xenon plasma emits a blue glow as the thruster operates. The photo on the right shows a similar non-operating Hall thruster. The photo on the left was taken at NASA’s Jet Propulsion Laboratory in Southern California; the photo on the right was taken at NASA’s Glenn Research Center.
We have fairly scant information about Psyche, as witnessed by the image below, which comes out of an asteroid-imaging project nicknamed HARISSA that is being run by the European Southern Observatory, using adaptive optics on the Very Large Telescope. The survey data along with ground-based radar imaging has determined that Pyche is roughly 226 kilometers wide and contains two interesting surface features, the first of which is a bright area christened Panthia. The second is a huge crater about half the size of the asteroid itself, which the ESO team calls Meroe. No moons have turned up in this work, at least none larger than one kilometer, eliminating one marker for determining the asteroid’s mass.

Image: Views of Psyche from the HARISSA survey, with Meroe and Panthia highlighted. Credit: ESO/LAM.
Orbiting between 378 and 497 million kilometers from the Sun, between the orbits of Mars and Jupiter, Psyche takes five years to complete an orbit, with a rotation period of a little over four hours. If the asteroid is the core of what would have been a planet-sized object, the mission’s science instruments should be able to make the call. They include a multi-spectral imager, a magnetometer, a radio instrument for gravity measurement (tricky at this potato-shaped object) and a gamma-ray and neutron spectrometer. We can hope this will be sufficient to untangle a past likely marked by violent collisions in the era in which the planets were forming.
To keep up with Psyche mission developments from the inside, check the Psyche blog at Arizona State. Here’s a snip from the most recent entry (June 14), from Paige Arthur, who captures some of the excitement of being involved in a deep space mission at building 179, the famed home of JPL’s Spacecraft Assembly Facility:
Engineers and technicians adorned in lab coats, gloves, masks, and hair nets meander around on the floor below, taking measurements with multimeters and mating connectors not too different from the ones Will and I had been handling in the testbed moments before. Along the opposite wall are plaques commemorating all of the spacecraft that have been assembled there — Mariner, Ranger, Voyager, Galileo, Cassini, Curiosity, Opportunity, and, most recently, Perseverance. But in this moment I don’t notice any of it, because my attention is totally captured by the massive spacecraft suspended in the middle.
The Psyche Chassis is a huge black, silver, and gold box the size of a car with an antenna dish fixed to one end. Long struts surround the dish like the spindly legs of a massive aluminum spider, and red electronic boards connected by thick, snaking cords populate the sides. I’ve spent the last two and a half years at JPL working with software simulations and engineering models, with bits of pieces of Psyche’s brain, eyes, and heart, but seeing the physical manifestation of all that work suspended before me in the form of such a tremendous machine still brings me chills.
I’ve never worked on a spacecraft, but I’ve felt the chills, particularly at JPL one day many years ago when I watched Spirit and Opportunity being readied for shipment to Florida. Go Psyche.
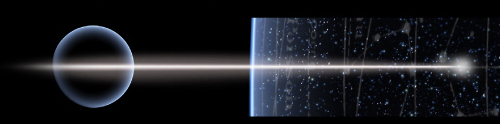
by Paul Gilster | Sep 21, 2021 | Astrobiology and SETI |
I try to keep my ear to the ground (rather than my eye to the sky) when it comes to SETI. What I mean is that there are enough scientists working SETI issues that it’s a challenge to know who is doing what. I try to track ongoing discussions even when, as at a conference, people keep ducking into and out of audibility. Hence the possibility of overlap in SETI efforts and, as Jason Wright points out in a discussion on his AstroWright site, the circulation of the same ideas without moving the ball forward.
This is hardly a new phenomenon, as a look back at my own grad school experience in a much different area reveals. I was a medievalist with an ear for language, and I was always struck by how compartmentalized we tended to be when discussing medieval linguistics. At that time, northern European tongues like Gothic, Old Icelandic, Anglo-Saxon and Old Saxon formed a scholarly thicket I happily wandered through, but in the absence of computerized resources back in the day, the Gothic scholars had a hard time keeping current with the Old Icelandic papers, and new work on the Anglo-Saxon alliterative line arrived mostly by rumor picked up at coffee time. These Germanic languages were definitely not talking to each other.
Bear in mind, that was a small, tightly focused community of scholars working on very esoteric stuff, and even then it was hard to keep up with the various strands of the tapestry. SETI’s problems are of a different sort. Here, the work is scattered across numerous journals and in particular, a wide range of disciplines — you might, for example, find a SETI paper in a journal of anthropology, well outside an astrophysicist’s normal range of sampling. Wright also makes the good point that SETI suffers from a lack of a curriculum, though he himself is working to change that at Penn State. What SETI does have going for it that my grad experience didn’t have is the proliferation of online resources, even if many are walled away like medieval monks behind monastic firewalls. Online access remains an emphatically moving target.
A useful paper that is helping to stabilize things is available on the arXiv site under the title “Furthering a Comprehensive SETI Bibliography.” It’s the background on the discovery process and categorization issues of an ongoing bibliographic update called SETI.news, which Centauri Dreams readers will want to know about. The newsletter began in 2016 as a way of gathering academic articles and occasional blog posts with high relevance to the SETI effort and I’m finding it indispensable.
The combined July/August SETI.news mailing is here, thanks to the efforts of Wright and Macy Huston. I should also mention while we’re talking about resource gathering that a bibliographic effort useful to those of us following interstellar exploration is available through the Interstellar Research Group. The database is searchable; updates appear every weekday. The IRG’s remit is obviously broad in scope, ranging through everything from propulsion issues to astrobiology, unlike the highly focused SETI.news. But I think both will be of interest to the Centauri Dreams audience.
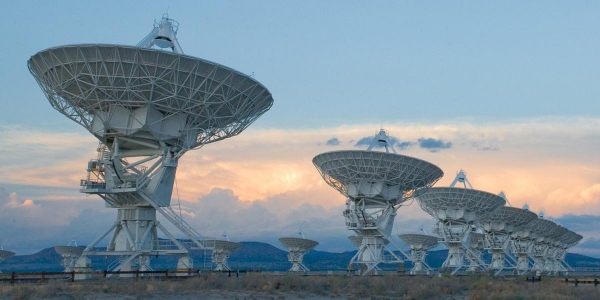
Image: The Very Large Array (VLA) is a collection of 27 radio antennas located at the NRAO site in Socorro, New Mexico, since early 2020 a participant in the hunt for technosignatures. Credit: Alex Savello/NRAO.
I don’t want to leave Wright’s interesting Strategies for SETI III: Advice post without mentioning his comments on the Fermi Paradox and the Drake Equation. He’s wondering whether we haven’t in some ways exhausted the discussion, quoting Kathryn Denning on the matter:
Thinking about that future [of contact with ETI] was itself an act of hope. Perhaps it still is. But I want to suggest something else here: that the best way to take that legacy forward is not to keep asking the same questions and elaborating on answers, the contours of which have long been established, and the details of which cannot be filled in until and unless a detection is confirmed. Perhaps this work is nearly done.
Have we driven the Fermi question into the ground? As per the earlier part of this post, it does seem that the discussion ranges around to the same issues without moving the ball forward, but then, a ball this theoretical is a hard thing to push down-field! The Drake Equation reliably gets an indignant rebuttal every now and then in my email from people who don’t realize that it is, as Wright points out, a heuristic tool that is designed to make us ponder the odds. So when someone writes me to point out that it is not possible to solve the equation, I think I’ll start quoting Wright’s comment:
[The Drake Equation is]…not a foundational equation like the Schrodinger equation from which one derives results, it’s more like a schematic map of the landscape to help orient yourself.
Schematic maps can be quite useful, and the Drake Equation has served its role. Everything in its place as we move forward. My own interest is more tightly focused on papers that can help with actual search efforts rather than theoretical ones, papers that, as Wright puts it, ‘stay close to the data.’ Which is not to underplay the sheer fascination of the topic, but only to say that the nuts and bolts have to be tightened and maintained, and a contribution like SETI.news is tangible and productive.
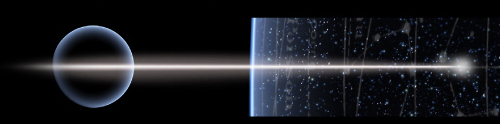