by Paul Gilster | Aug 31, 2006 | Deep Sky Astronomy & Telescopes |
Cosmological shadows? Theory predicts that objects between us and the source of the cosmic microwave background should cast them. Specifically, the hot gases found in clusters of galaxies should show a measurable shadow effect produced by that background radiation, and there are reports of such effects from various observers. The scattering of the cosmic microwave background by high-energy electrons is known as the Sunyaev-Zel’dovich effect.
However, a new study from the University of Alabama at Huntsville raises real problems. The first to study the phenomena with data from the Wilkinson Microwave Anisotropy Probe (WMAP), the team reports an oddly sporadic shadow effect for a background thought to be afterglow radiation from the Big Bang.
And that raises questions about the Big Bang model itself. Says physicist Richard Lieu, after an investigation involving 31 clusters of galaxies:
“These shadows are a well-known thing that has been predicted for years. This is the only direct method of determining the distance to the origin of the cosmic microwave background. Up to now, all the evidence that it originated from as far back in time as the Big Bang fireball has been circumstantial.”
And Lieu’s team is reporting that among the clusters studied, some showed the shadow effect and some did not. In fact, the actual shadow effect is about one-fourth of what was predicted. Which is decidedly odd, for the microwave background radiation’s effects ought to be obvious. “If you see a shadow…it means the radiation comes from behind the cluster,” Lieu adds. “If you don’t see a shadow, then you have something of a problem.”
All of which is certain to raise controversy and a spate of new studies using the publicly-available WMAP data. The Huntsville team examined hot ionized gases at the center of galaxy clusters; the free electrons there interact with the background radiation to create the shadow effect. Because the observed shadow effect more or less equals in strength the natural variations already observed in the microwave background, the question becomes acute. If the background radiation is closer than the galaxy clusters under study, what exactly is it and how did it arise? And what does this mean about the Big Bang?
The paper is Lieu, Mittaz and Shuang-Nan Zhang, “The Sunyaev-Zel’dovich effect in a sample of 31 clusters: A comparison between the X-ray predicted and WMAP observed decrement,” Astrophysical Journal, Sept. 1, 2006, Vol. 648, No. 1, p. 176. Now available here on arXiv.
by Paul Gilster | Aug 30, 2006 | Deep Sky Astronomy & Telescopes |
A black hole two billion times more massive than the Sun is not something you find every day. Even more unusual is to find it embedded in a quasar that is 12.7 billion light years from Earth. But that’s just what Tomotsugu Goto (Japan Aerospace Exploration Agency) seems to have found using the Subaru optical-infrared telescope on Mauna Kea. How a black hole of this mass could have formed only a billion years after the birth of the universe is only one of the questions this find poses.
For the object, found in the direction of Cancer, also shows via its spectrum that much of the hydrogen between the quasar and Earth is ionized. What would cause neutral hydrogen to be converted to ionized hydrogen in this early epoch? Ultraviolet radiation is thought to be the key, but observational evidence helping us understand how and when this occurred has always been tricky to gather for a reionization event that occurred over 12 billion years ago.
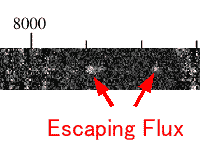
Quasars are useful beacons by which to study this reionization. Gamma ray bursts are also helpful in determining how the phenomenon occurred, but they’re brief and sporadic, whereas quasars appear bright and stable over long periods of time. Hence Goto’s plan to investigate still more distant quasars using the same methods he used here: searching the Sloan Digital Sky Survey for objects having the same color in visible light as quasars at 12.7 billion light years distance, then observing the candidate objects on Mauna Kea to eliminate all but the quasars being investigated.
Image: The CCD image of the spectrum. The fact that light is detected between 800 and 830 nanometers indicates that much of the hydrogen between Earth and the quasar must be ionized. Credit: Subaru Telescope, National Astronomical Observatory of Japan (NAOJ).
Centauri Dreams‘ note: When the early universe cooled sufficiently, electrons and protons combined to produce neutral hydrogen, an event that probably began about 300,000 years after the Big Bang. Today most of that hydrogen has been ionized, splitting into separate electrons and protons; the absorption of light at particular wavelengths causes this to happen. Because the spectrum of this quasar shows there was not enough neutral hydrogen to absorb the light between the wavelengths of 800 to 830 nanometers, we can put further constraints on the state of ionization in the early universe.
A good backgrounder on all this, with links to other important quasar work, is available from Rennan Barkana (Tel Aviv University). Barkana is also the author of “The First Stars in the Universe and Cosmic Reionization,” Science 313. No. 5789 (18 August 2006), pp. 931-934, with abstract available here.
by Paul Gilster | Aug 29, 2006 | Exoplanetary Science |
What sort of stars harbor the planetary systems we’ve thus far identified? The answer is easy: most of the known exoplanets were found through radial velocity surveys, and these focus on nearby Sun-like stars. Thus we’re looking at a range of stars between late-F and early-K class dwarfs, and almost all are within 50 parsecs of the Sun. It is also apparent that planetary systems in our scope of observation increase with increasing metallicity of the parent star, a measure of elements higher than hydrogen and helium.
Are there other trends we can identify? Perhaps not. As I. Neill Reid (Space Telescope Science Institute) writes in a new paper on the subject, “With the possible exception of a higher mean velocity perpendicular to the Plane, the planetary hosts appear to be unremarkable members of the Galactic Disk.” There is not, for example, a correlation we might expect to find in metal-rich stars between the mass of the primary star and the masses of its planetary companions (consider the M-class dwarf Gl 876, which harbors two Jupiter-class planets). Reid thinks this points to high metallicity as a trigger for planet formation rather than a player in the formation mechanism itself.
What’s fascinating about this work is what Reid calls a ‘back of the envelope’ calculation of how many Solar-type stars may have gas giant companions. These are systems that would be detectable by today’s radial velocity measurements were we within range. Reid examined a ring of space (call it the ‘solar circle’) drawn around the galaxy at roughly Sol’s distance from galactic center.
The answer, developed with reference to metallicity distribution in our local galactic neighborhood: 3.5 X 107 such planetary systems detectable via radial velocity methods within a range of 6 to 10 kiloparsecs from galactic center. In other words, 35 million planetary systems around Sun-like stars within a ring of space defined as roughly 20000 to 32000 light years from the center of the Milky Way.
Centauri Dreams‘ note: By way of comparison, our Sun is roughly 26,000 light years (8000 parsecs) from galactic center, so we’re getting a view of the density of planetary systems within a ring drawn around the galaxy at roughly the same distance from the center as Sol. Back of the envelope it may be, but it’s the first such calculation I know of that draws on existing metallicity data to reach a conclusion that moves beyond pure guesswork, and it’s heartening for the encouragement it provides that we’re going to find planetary systems in all but limitless abundance as we expand the search.
by Paul Gilster | Aug 28, 2006 | Breakthrough Propulsion |
Are we ever going to understand what makes matter resist acceleration? If we can get a handle on inertia, we’ll have a better idea what’s possible when it comes to exotic propulsion. 19th Century physicist Ernst Mach believed that inertia was the result of matter being acted upon by all other objects in the universe, even the most distant ones. At the University of California at Fullerton, James Woodward has been studying inertia in a Machian context for some time, and an implication that appears to grow out of it: an object undergoing acceleration may experience transient fluctuations in its mass.
It will take a great deal of experimentation to find out whether there is anything to this, but the idea is interesting enough to keep Woodward working. His theories are put to the test in the laboratory, for they predict an effect that should be measurable. Indeed, his work with capacitors produces results that can be interpreted as mass reduction, though getting a clear data signal through the experimental noise is not easily done. How such mass reduction squares with the laws of physics, and just how far it can be taken — is this a clue to possible anti-gravity effects? — are questions that remain unanswered.
But the implications are intriguing. Be aware, then, of two sites that focus exclusively on Woodward’s activities. The polymath (he’s a member of both the history and physics faculty at Fullerton) maintains a research page of his own with links to older papers explaining his theories, and a bibliography of recent publications. A PDF on propellantless propulsion is useful, as is the older paper “Mach’s Principle and Impulse Engines: Toward a Viable Physics of Star Trek?” which Woodward presented at NASA’s Breakthrough Propulsion Physics workshop in 1997.
Woodward doesn’t refer to what he’s studying as the ‘Woodward Effect,’ but the name has begun to settle in, and Peter Vandeventer maintains a Woodward Effect site containing background papers (unpublished) and links to further information. As Vandeventer notes, Woodward prefers to talk about the ‘Mach Effect,’ a refreshing dose of humility in a world filled with people intent on naming things after themselves. Whatever we call it, the effect studied by Woodward and others gives some credence to the notion of a ‘Mach-Lorentz thruster,’ a spacecraft that, as Woodward once said, “…puts out thrust without blowing stuff out the tailpipe.”
Be aware, too, of a recent paper by Martin Tajmar, Florin Plesescu and team that discusses work sponsored by both the US Air Force and the European Space Agency. The authors attempted to “…measure the gravitational field induced by a non-stationary gravitomagnetic field generated by an angularly accelerated superconducting ring.” If confirmed, these findings would appear to demonstrate the production of a gravitomagnetic field of measurable magnitude in the laboratory. Which is to say that years of research lie ahead to examine such effects and place them in a sound mathematical context.
We may find, of course, that there are other explanations for the results of both Woodward’s and Tajmar’s experiments — Tajmar, for example, notes quite different results from earlier claims by Evgeny Podkletnov about gravitational shielding effects above rotating superconductors. But provocative work that tests the boundaries of known physics is worth keeping an eye on as followup investigations continue. Most hypotheses fail — this is how science works — and we are early on in putting a number of fascinating concepts to the test. Let’s hope funding for the most testable of these ideas continues to emerge as we get an idea of which hypotheses make sense.
by Paul Gilster | Aug 26, 2006 | Deep Sky Astronomy & Telescopes |
Now and then it’s good to step back from interstellar advocacy, especially on a weekend, and just look at the sky. To that end, the photograph below can be considered an object of contemplation, something to quiet the rush of the work week and return the mind to the far reaches. You’re looking at a region within the Large Magellanic Cloud that contains hot blue stars, some of them brighter than a million Suns. That kind of energy pushes stellar ‘winds’ — charged particles moving at tremendous speeds — out into the surrounding interstellar gas.
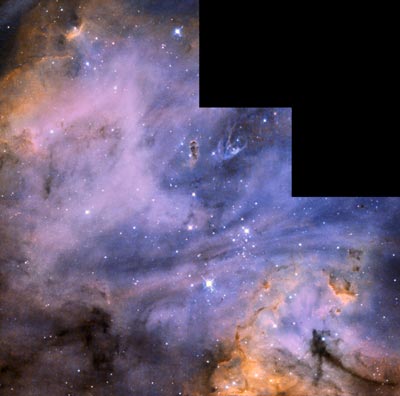
Image: This active region of star formation in the Large Magellanic Cloud (LMC), as photographed by NASA’s Hubble Space Telescope, unveils wispy clouds of hydrogen and oxygen that swirl and mix with dust on a canvas of astronomical size. The LMC is a satellite galaxy of the Milky Way. Credit: NASA, ESA, and the Hubble Heritage Team (STScI/AURA).
And look at those dust streamers running the length of the nebula, as well as the bright orange of dust clouds at bottom right and top left. Put enough charged particles into this environment and you begin to compress the interstellar dust, leading to another round of star formation. Filters were used on this Hubble image to isolate and colorize the hydrogen and oxygen gas, achieving this lovely result. The image was made in 1998 by Hubble’s Wide Field Planetary Camera 2.