by Paul Gilster | Feb 29, 2008 | Culture and Society |
A friend of mine who knows more about classical music than anyone I’ve ever met, and who has turned his passion for it into a second career, asked me a question a few years ago that stays with me. A great admirer of Toscanini, he wondered whether some of the the conductor’s prodigious output was in some sense still ‘out there.’ For Toscanini went to work in New York after he left Italy, conducting the first broadcast concert of the NBC Symphony Orchestra in 1937. His NBC broadcasts were, of course, recorded, but my friend’s thoughts had turned interstellar and he wondered where those radio signals were now.
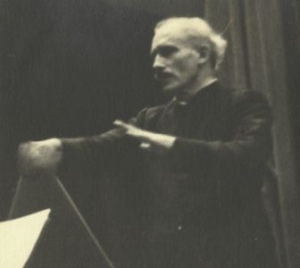
We discussed radio signals propagating outwards at the speed of light, so that a 1937 broadcast would now be 71 light years out, and in answer to his query, I said yes, if you could somehow position yourself through superluminal means 71 light years from here, you would be on the wavefront as the initial Toscanini broadcast swept over you. But, I assured him, you wouldn’t be able to hear it. For one thing, most of the radio signal, given its frequency range, would have bounced off the ionosphere and returned to Earth. What signal might have managed to leak out would have become so attenuated as to essentially fade into background noise.
Image: The great Toscanini at work. Want to hear his first 1937 NBC Symphony Orchestra concert? You’ll need to be 71 light years from Earth, and armed with some serious receiving equipment.
Or would it? The question keeps coming up because we are actively scouring the heavens looking for radio or optical signals from other civilizations. Would we actually be able to hear not just a directed beacon, but extraneous transmissions of the sort that the Toscanini broadcasts represent? Broadcasts aimed only at a local audience on a particular planet but nonetheless spreading outwards into the nearby galactic neighborhood? Opinion on this seems mixed, but an online essay by Seth Shostak makes the case that television signals may be detectible, depending on the kind of equipment being used to handle the reception.
Shostak figures that an alien civilization with an antenna farm 15 miles on a side, one that used a collection of simple rooftop antennae spaced every ten feet that hooked up to receivers no more noise-free than those we currently build for our radio telescopes, could pick up TV carrier signals from fifty light years away. A carrier is easier than any other part of the TV broadcast to pick up because the signal is more concentrated within it, and picking it up would offer priceless information.
“The carrier would tell them we’re here – that the red “on the air” sign is lit, and intelligent critters exist on planet Earth. If they found the carrier too boring, and wanted to actually watch Lucy drive Desi nuts, they’d need an antenna farm 150 times bigger in each direction. That’s a large herd of antennas, approximately the size of the United States, and probably not something you’d appreciate outside your picture window. But it’s hardly an unimaginable engineering feat, especially if the aliens are somewhat ahead of us in technical development.
Another example from the article: Arecibo’s one megawatt radar transmitter (that great, endangered instrument we need so badly in the hunt for Earth-crossing asteroids), would be receivable at 320 light years by a receiver with Arecibo-like characteristics. If this is the case, then my friend’s question is answered in a gratifying way for a man who reveres Toscanini’s memory. Yes, in some sense, the great maestro’s music still lives as the wavefront pushes inescapably outwards.
The advantage to us? Assuming a civilization roughly at the stage of development of our planet from, say, 1900 to the present, we might be able to pick up its extraneous signals with sufficiently sensitive equipment (think ‘dark side of the Moon’ in terms of receiver location, and assume advances we might expect shortly). Listening for interstellar beacons has always seemed unproductive to me because of the faint likelihood we might be chosen for such a signal, but simple by-products of normal broadcasting activities would indeed be more likely, assuming such were available and — the big ‘if’ — assuming the existence of a roughly parallel civilization within a star system in the surrounding hundred or so parsecs.
Frankly, I think the odds on that are longer than of my friend’s finding a way to travel 71 light years to listen to his Toscanini broadcasts. But the question of our ‘visibility’ to neighboring star systems is intriguing enough that I’m surprised I’m not seeing more work on it. Shostak mentions a recent TV broadcast in which a “…pundit apparently proclaimed that earthly TV broadcasts would be hopelessly scrambled after penetrating only a few light-years into space.” Does anyone have pointers to recent work on this question that I haven’t been able to track down? And as far as AM radio from the 1930s goes, am I right that a tiny bit of such broadcasts might make it through the ionosphere, or do we have to wait for the advent of FM and television signals to make that jump?
by Paul Gilster | Feb 28, 2008 | Interstellar Medium |
We think about our interstellar neighborhood in terms of stars, like Alpha Centauri and Tau Ceti, but the medium through which our relative systems move is itself a dynamic and interesting place. The Sun is currently passing through a shell of material known as the Local Interstellar Cloud. And that cloud is, in turn, located at the edge of a vast region known as the Local Bubble, scoured of material by supernova explosions in the nearby Scorpius-Centaurus and Orion Association star-forming regions. Within the past 105 years, the Sun emerged from the interior of the Local Bubble; it now moves obliquely in the direction of the high-density molecular clouds of the Aquila Rift, a star-forming region that itself reminds us how energetic ’empty’ space really is.
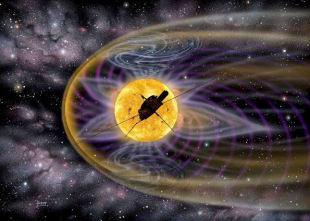
If we’re ever going to send fast missions outside the Solar System, we’re going to need plenty of data about the materials through which our vehicles move, particular as velocities mount to the point where collision with even small particles can be devastating. As of now, we’re only piecing together a broad idea of the galactic environment within 500 parsecs of the Sun, and remain in need of more detailed, in situ results from regions much closer in. Fortunately, space-based data have begun to accumulate.
Image: An artist’s take on the Ulysses mission, now approaching the end of its life, but a workhorse that has taught us much about the interstellar medium. Credit: Jet Propulsion Laboratory.
Take the Ulysses mission, which Harald Krüger (Max-Planck-Institut für Sonnensystemforschung) and Eberhard Grün (Max-Planck-Institut für Kernphysik) examine in their recent paper. The scientists take a hard look at the Sun’s position and its relation to nearby interstellar dust, noting interesting clues like the Arecibo radar findings about micron-sized interstellar meteor particles, which seem to radiate from the direction of the Geminga pulsar. Are these evidence that the supernova responsible for the Geminga pulsar created the Local Bubble itself?
At this point, we simply don’t know, but space-based studies of these exotic materials should help to clarify things. First examined during the 1930s, when astronomical evidence of starlight scattering and weakening gave clear signs of its existence, interstellar dust became available for close study when spacecraft began flying dust detectors. It became clear thirty years ago that interstellar dust grains can cross the heliospheric boundary and make their way toward the Solar System. In the 1990s, Ulysses’ dust instrument identified the mass, speed and approach direction of impacting grains as they swept through the heliosphere.
Krüger and Grün write about using future Ulysses studies to learn more, but recent NASA news seems to rule that scenario out, as the spacecraft’s power systems are in a state of terminal decline. Future Ulysses studies would have been useful for two reasons: The spacecraft’s data would have covered the entire 22-year range of a solar cycle, offering a unique set of measurements. And further readings from the outer heliosphere might have made it possible to explain the origin of an odd thirty degree shift in the flow direction of the dust Ulysses monitored.
For data from the period 1996 to 2000 showed a specific impact direction from interstellar dust, which little prepared investigators for the following:
Six years later, when Ulysses was travelling through almost the same spatial region and had an almost identical detection geometry for interstellar grains, the situation was vastly different: ?rst, the range in approach directions of the grains was somewhat wider…; second, and more noticeable, in 2005/06 the approach direction of the majority of grains was shifted away from the helium ?ow direction. Preliminary analysis indicates that this shift is about 30? away from the ecliptic plane towards southern ecliptic latitudes… At the moment, we do not know whether it is a temporary shift limited to the time period stated above or whether it continues to the present time. Furthermore, the reason for this shift remains mysterious. Whether it is connected to a secondary stream of interstellar neutral atoms shifted from the main neutral gas ?ow… is presently unclear.
So even within the Local Interstellar Cloud, through which we now move, the concentration of dust is little understood. Properly interpreted, these dust grains may be able to tell us more about how heavy elements are moved about within the interstellar medium and how they are affected by the five distinct clouds of gaseous material within five parsecs of the Sun. Data from the recent Stardust mission should be of help, their interpretation aided by what Ulysses has found so far. What we lack, of course, are direct observations of interstellar dust outside the Solar System, something that future missions like Innovative Interstellar Explorer may remedy if funded for launch some time in the next decade.
The paper is Krüger and Grün, “Interstellar Dust Inside and Outside the Heliosphere,” submitted to Space Science Reviews and available online.
by Paul Gilster | Feb 27, 2008 | Astrobiology and SETI |
I’ve always found the idea of panspermia oddly comforting. Growing out of the work of Swedish chemist and Nobel Prize winner Svante Arrhenius, panspermia assumes that life can move between worlds by natural means, and implies that planets with the right conditions will wind up with living things on them. That idea of all but universal life, and the weird notion that we might all be in some way ‘related,’ was exhilarating to thinkers like Fred Hoyle and Chandra Wickramasinghe, who went on to suggest that the influx of life from space triggers continuing changes on Earth today, which might involve epidemics and new diseases.
Now comes a variant called lithopanspermia, which questions whether rocks blasted off a planetary surface by impacts might not be the transfer vehicles for microorganisms that travel between planets and perhaps further. After all, we have found Martian meteorites in Antarctica, forty or so to date, so the real question becomes the survival possibilities. Can a microorganism survive the impact of the original projectile into its home world and the journey through space, which in the case of Martian meteorites ranges from between one and twenty million years?
A study in the latest issue of Astrobiology makes the case that survival is possible. Endolithic cyanobacteria and epilithic lichens can withstand incredibly harsh conditions here on Earth and become prime candidates for investigating their potential passage through space. The team simulated shock pressures of the sort the organisms might have experienced when being blasted off the Martian surface, with results that support space transport at least within planetary systems. From the paper (internal references deleted for brevity):
A vital launch window for the escape from Earth’s gravity field may only be achieved by very large impact events that blow out at least part of the atmosphere… The direct ejection and escape of rocks from Earth is very difficult because of the required very high escape velocity (11 km/s) and the decelerating effect of Earth’s dense atmosphere. Therefore, “mega-impacts,” which occurred frequently only during the “early heavy bombardment phase,” i.e., before 3.75 billion years ago…, would be required to transfer sufficiently large fragments of moderately shocked rocks into space. Our results enlarge the number of potential organisms that might be able to reseed a planetary surface after “early,” very large impact events… and suggest that such a re-seeding scenario on a planetary surface is possible with diverse organisms.
Re-seeding a planetary surface is an interesting scenario in itself. It implies that a major asteroid strike might not spell complete doom for life on the planet, no matter how devastating the effects on the surface below. Rocks eventually falling back to their home world have the potential for launching life processes all over again. And, of course, the concept of life moving between entirely different planets receives another boost from this work, which gives “…further support to the hypothesis of lithopanspermia for a viable transport from Mars to Earth or from any Mars-like planet to another habitable planet in the same stellar system.”
The paper is Horneck et al., “Microbial Rock Inhabitants Survive Hypervelocity Impacts on Mars-Like Host Planets: First Phase of Lithopanspermia Experimentally Tested,” Astrobiology Vol. 8, No. 1 (2008), pp. 17-44 (available online).
by Paul Gilster | Feb 26, 2008 | Missions |
When you’re thinking interstellar, long time frames are inescapable. Are we capable as a culture of planning missions that last not only longer than a single human lifetime, but longer than multiple generations? Steve Kilston (Ball Aerospace & Technologies), with help from Sven and Nancy Grenander, clearly thinks so. The three are behind the fittingly named Ultimate Project, a starship designed to carry one million humans across the light years separating us from the nearest stars, creating colonies and perhaps going on from there, a ten thousand year star journey that could turn into a trek through the galaxy lasting for millions more.
For just to get such a mission to the launch point, Kilston is thinking in terms of century-long segments within an overall 500-year plan. 100 years to develop the plan for the mission. 100 more years to achieve a detailed design. Now a century for prototyping and demonstrating technologies, followed by a century to assemble materials and construct the spacecraft. A final one hundred years for a shakedown cruise in the Solar System. You can see that we’re looking at societal commitments on the order of the great cathedrals of Europe, but going substantially beyond even their protracted construction in terms of time and expense.
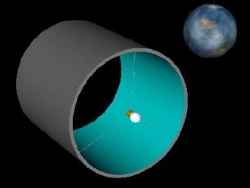
A few assumptions travel with this project. Because scientific discoveries are much harder to predict than changes in technology, the Ultimate Project assumes new technology but no new physics. And it operates with the certainty that experiments and discoveries in coming centuries will alter its plans, that experience triumphs over theory every time, and that advances en-route will continue the process of updating the plan until the mission is accomplished. I’m drawing these premises from a presentation Steve Kilston made at the second Space Mission Challenges for Information Technology conference, which provides far more background material than I can offer here.
That being said, the current daunting specs are what you might expect of an interstellar ark: A vehicle of 100 million tons, with a cost of $50 trillion, built as a cylindrical shell some two kilometers long and two kilometers in diameter, with ten or more decks of habitable space and 1 g of gravity provided by rotation. The great pyramid of Cheops has one-sixteenth the mass of this ship, which carries twice the ‘land’ area of the island of Manhattan, offering 125 square meters per person. The propulsion method: Magnetically confined deuterium/Helium-3 fusion, a technique familiar from Project Daedalus here updated and now using the atmosphere of Uranus for the necessary store of Helium-3.
Expansion plans? Count on twenty Sun-like stars within six parsecs of Earth, a range reachable within 10,000 years. Figure 2000 years to settle a new planet and build two new starships to continue the voyage. The net rate of expansion becomes one parsec every 2000 years, with the Milky Way explored in some forty million years. The Ultimate Project’s Web site is bare-bones at present and the link to the Project’s recent poster presentation at the JPL Exoplanet Fair is broken, but I assume that oversight will be repaired soon. Or perhaps not. With five hundred years to launch, the site operators may see no need to rush.
Steve Kilston has been involved with Webster Cash’s New Worlds starshade concepts and has worked at Ball Aerospace on imaging Earth-like planets around other stars. He may be an optimist in terms of the cost of the Ultimate Project and my guess is that 500 years from now the type of fusion the Project now focuses on will have been superseded, but trans-generational endeavors begin somewhere, and I know of no one else who is currently trying to do what the British Interplanetary Society once did, bringing solid scientific and engineering knowledge to bear on a complete design aimed at reaching the stars. For that reason alone, the Ultimate Project is worth keeping on your watch list.
by Paul Gilster | Feb 25, 2008 | Exoplanetary Science |
What are the chances that we’ll find habitable planets around Alpha Centauri A and B? Centauri Dreams has long kept an eye on the work of Greg Laughlin (UC-Santa Cruz) and colleagues, who have been working on the Alpha Centauri question with ever more interesting results. Following their work on Greg’s systemic site has been fascinating, and for those who would like to be quickly brought up to speed, it’s useful to know that Laughlin has made their recent paper summarizing these findings available online. Anyone serious about the study of these closest stars to Earth will want to download and read these promising results.
Laughlin’s group simulated the formation of planetary systems around Centauri B, beginning with a disk populated with 400 to 900 lunar-mass protoplanets, then following its development over 200 million years. To say the results are encouraging would be an understatement. All the simulations lead to multiple-planet systems, with at least one planet of Earth mass or slightly larger within the tantalizing range of 0.5 to 1.5 AU. I’m going to poach Greg’s diagram from the latest systemic article by way of summarizing these findings below; please consider this a pointer as well to add systemic to your regular reading list.
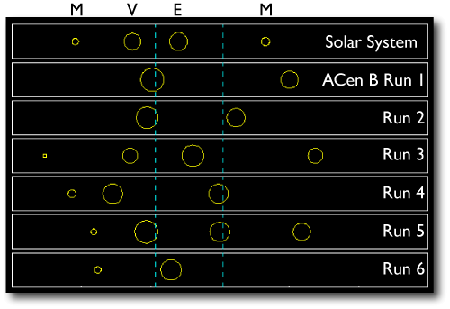
Image: Findings from Laughlin’s team on the kinds of planetary systems we should expect around Alpha Centauri B. The team includes UC-SC graduate student Javiera Guedes, Erica Davis, Eugenio Rivera, Elisa Quintana and Debra Fischer. Credit: Greg Laughlin/systemic.
Note the somewhat larger planetary sizes of the larger planet in each run as compared to the Earth (in the top row). Laughlin notes that Centauri B’s higher metallicity should lead to more massive terrestial planets, as the simulation confirms. The question now arises, with orbits of terrestrial worlds now believed to be theoretically possible around both Centauri A and B, and with simulations showing the possibility of a rocky world in the habitable zone of Centauri B, what can we do to get a confirmed detection? It would be one of the most significant finds in exoplanetary science, one that would fire the imagination and immeasurably boost the case for close-up studies of these stars and their possibilities for life.
We’ve detected almost three hundred exoplanets, some of them (via microlensing) thousands of light years from home. Why haven’t we been able to track down planets around these closest of all stars? The answer lies in the limitations of our radial velocity methods, but as Laughlin and team show, the right kind of survey may be able to surmount them.
But first, what work has been done on Centauri planets? In fact, we have excellent observations of these stars dating back over 150 years because of their proximity and sky-dominating visibility, with radial velocity data for both Centauri A and B tabulated since 1904. A 1999 study was able to determine, for example, that the stars are not orbited by any planet with a mass above ten Jupiter masses. Two years later, a new analysis placed tighter limits on Centauri planets: No planets larger than 2.5 Jupiter masses for Centauri A and 3.5 Jupiter masses for Centauri B could exist.
Laughlin’s team now takes the mass of any planet in a circular orbit within 1 to 3 AU down to somewhere in the neighborhood of 0.3 Jupiter masses around Centauri A and 0.5 Jupiter masses around Centauri B. No gas giants around the Centauri stars! And with consistent findings that Earth-mass planets can form within 2.5 AU of these host stars and remain stable for billions of years, the outlook for small planets is quite promising. Bear in mind, too, that 20 percent of all planets discovered thus far have been found in multiple systems, with three around binaries with an orbital separation similar to that between Centauri A and B.
Finding small planets is no easy task, because it’s much easier to identify a large planet with radial velocity methods; the signature of an Earth-mass planet is vanishingly small. But Laughlin’s team believes it is achievable. From the paper:
A successful detection of terrestrial planets orbiting ? Cen B can be made within a few years and with the modest investment of resources required to mount a dedicated radial-velocity campaign with a 1-meter class telescope and high-resolution spectrograph. The plan requires three things to go right. First, the terrestrial planets need to have formed, and they need to have maintained dynamical stability over the past 5 Gyr. Second, the radial velocity technique needs to be pushed (via unprecedentedly high cadence) to a degree where planets inducing radial velocity half-amplitudes of order cm s?1 can be discerned. Third, the parent star must have a negligible degree of red noise on the ultra-low frequency range occupied by the terrestrial planets.
That latter is worth commenting on. While both of the primary Centauri stars are thought to be likely candidates for planets, Centauri B is the easier to investigate because compared to its companion star, Centauri B is exceptionally quiet in terms of the kind of oscillations and stellar activity that can produce ‘noise’ in radial velocity data. Studies of Centauri B in the X-ray spectrum indicate low chromospheric activity of the sort that would be associated with only weak stellar flares. If that assumption holds true, we may be able to push radial velocity methods down to the exceedingly fine scales needed to detect planets of this size.
The key word is ‘dedicated,’ the authors assuming the need to access a telescope capable of continuous observations over lengthy periods. It takes time to accumulate radial velocity data, particularly when working at this level of detail, but a detection of one or more Centauri planets within a few years, possibly within the habitable zone, makes this an exciting prospect. The Centauri stars thus become not just valuable targets in and of themselves, but also serve as a testbed for pushing radial velocity techniques to new levels. Imagine the magnitude of this discovery:
Our simulations and those of Quintana et al. (2007) show that for disks with small inclinations relative to the binary orbit, large terrestrial planets tend to form in or near the habitable zone of ? Cen B. Thus, it is possible we may detect a habitable terrestrial planet around at least one of our nearest stellar neighbors.
The paper is Guedes et al., “Formation and Detectability of Terrestrial Planets around ? Centauri B,” accepted by The Astrophysical Journal. Now available at the arXiv site.