by Paul Gilster | Nov 30, 2009 | Exotic Physics |
I had intended to start the week with a look at Charlie Stross’ ideas on the ‘starship’ metaphor, but I’ll hold the Stross essay until tomorrow because I want to finish up Marcus Chown’s piece in New Scientist. On Friday we talked about the idea of using Hawking radiation from a man-made black hole to propel a starship. That’s outrageous idea number one, but Chown actually began the article with a look at Jia Liu’s ideas on using dark matter to propel a ship, something along the lines of a Bussard ramjet without the hydrogen.
The Uses of Speculation
I enjoy looking at speculative concepts, even when they’re so far out on the edge that they’ve attained a kind of intellectual redshift, but New Scientist‘s squib for the story surprised me: “We could reach the stars if we built a black hole starship or a dark matter rocket – we’ve got the physics to do it.”
Er, no, we don’t have the physics to build a dark matter rocket. We don’t know what dark matter is. The case for its existence seems strong, based on anomalous galactic rotations and galactic cluster gravitational lensing, but we’re nowhere close to understanding the stuff. The rhetorical stance that should be de rigeur for discussing ideas on the edge is a simple acknowledgment that speculation is worth doing for its own sake and does not necessarily imply fast or feasible implementation. No further justification needed as long as we don’t play to the grandstand.
Robert Bussard is well known among propulsion theorists, having proposed as far back as 1960 that a ramjet scooping up interstellar hydrogen with magnetic fields could sustain a fusion reaction and thus work its way up close to the speed of light. Indeed, the Tau Zero Foundation was named after Poul Anderson’s wonderful novel Tau Zero (1970), which posited a runaway Bussard ramjet whose crew couldn’t shut down the engine, to the point where the ship was punching through entire galaxies in mere minutes of time as experienced by the crew. No spoiler here — you’ll need to read the book (a classic) to see how it ends.
An Alternative to the Bussard Ramjet
Jia Liu (New York University) has the notion of modifying the Bussard ramjet to use dark matter, and this is precisely where we do not have the physics to proceed (at least, not yet). If dark matter is made of neutralinos, just one of the dark matter candidates, then the curious thing is that neutralios are their own antiparticles. As Chown writes: “…two neutralinos colliding under the right circumstances will annihilate each other.” The benefit is obvious — 100 percent of the mass of the dark matter is converted into energy.
As with the Bussard concept, the faster the dark matter starship goes, the better. From the article:
Liu points out that the faster his dark matter rocket travels, the quicker it will scoop up dark matter and accelerate. Precisely how quickly it can accelerate depends on the density of the surrounding dark matter, the collecting area of the engine and the mass of the rocket. In his calculations, Liu assumes the starship weighs a mere 100 tonnes and has a collecting area of 100 square metres. “Such a rocket might be able to reach close to the speed of light within a few days,” he says. So the journey time to Proxima Centauri would be slashed from tens of thousands of years to just a few.
A Dark Matter Transportation Hub
But a rocket like this would be at its best when encountering dense regions of dark matter. It’s optimized, in other words, for the galactic core and any areas where dark matter should be concentrated. In his recent paper on the subject, Liu says that fact makes him think of current human transportation, especially when he examines N-body simulation pictures of dark matter:
In the picture, there are bright big points which stand for large dense halos, and the dim small points for small sparse halos. Interestingly, these halos have some common features with the cities on the Earth. The dense halos can accelerate the spaceship to higher speed which make it the important nodes for the transportation. However, the sparse halos can not accelerate the spaceship to very high speed, so the spaceship there would better go to the nearby dense halo to get higher speed if its destination is quite far from the sparse halos.
Remind you of anything? Well, I’m just back from Austin, which I had to reach by going through Dallas. To get to Cleveland from here, I have to go to Chicago.
Similarly, if we want to take international flight, we should go to the nearby big cities. The small cities usually only have flights to the nearby big cities, but no international flights. Thus we can understand the dense halos may be very important nodes in the future transportation, like the big cities on the Earth.
Too Weak an Interaction?
I must say there are airline hubs that remind me more of black holes than dark matter concentrations, but let that pass. The ‘hub’ comparison is familiar, but everything else about this has us shooting in the dark. Just as we have not nailed down our model for dark matter, so we have no accurate idea of where all the dark matter concentrations are located in our own galaxy, other than the apparent clustering at the core. We will presumably develop maps of the stuff one day. We also run into the problem that some models of dark matter assume extremely weak interactions with normal matter. Assuming dark matter exists (and I believe it does), this would account for the extreme difficulty thus far in identifying dark matter particles through ongoing experiments here on Earth.
Liu is upfront about this, positing in the second paragraph of his paper that the particles he’s dealing with do interact with normal matter: “Here we assume the DM particle and the annihilation products can not pass through the wall of the box.” The ‘box’ is the spacecraft engine, which he sees as having an open door that accepts incoming dark matter, after which “…we compress the box and raise the number density of the DM for annihilation, where we assume the annihilation process happens immediately.”
Later in the paper, Liu focuses again on the problem of weak interactions in various dark matter models:
The most serious problem comes from DM which are weakly interacting with matter. Current direct searches of DM have given stringent bound on cross-section of DM and matter. It may be difficult using matter to build the containers for the DM, because the cross-section is very small. However, the dark sector may be as complex as our baryon world, for example the mirror world. Thus the material from dark sector may build the container, since the interactions between particles in dark sector can be large.
A New Reaction Mass
So we don’t know what dark matter is yet, and we may be dealing with something that can’t be housed in any propulsion system made of normal matter because it fails to interact with it. But let’s not give up on dark matter as reaction mass just yet. Given that it is thought to outweigh visible matter by about six to one, finding a way to exploit it for propulsion would be a major breakthrough, and one we cannot rule out for the far future. If the neutralinos needed for Liu’s starship are available, exploiting their energy may be well beyond our current technology, but until we’ve got a handle on what dark matter actually is and how it interacts, continuing speculation seems advisable.
The paper is Jia Liu, “Dark Matter as a Possible New Energy Source for Future Rocket Technology,” available online.
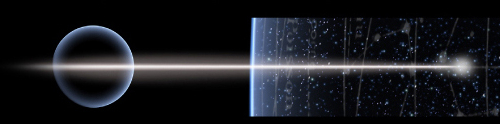
by Paul Gilster | Nov 27, 2009 | Exotic Physics |
When you consider that conventional chemical rockets extract a mere 10-8 of the energy locked up in their fuel, the attraction of antimatter becomes undeniable. Could we build an engine that extracts 100 percent of the energy created by matter-antimatter annihilation? Louis Crane (Kansas State University) is dubious, pointing to problems of storage and the difficulty of making enough antimatter to get the job done.
Black Holes as a Propulsion Option
Working with colleague Shawn Westmoreland, Crane has been exploring a different and far more speculative option for upping the energy extraction levels. What about using black holes for propulsion? Specifically, Crane and Westmoreland ask whether Hawking radiation from black holes can power a starship, calculating that a black hole of about a million tons would be just the right size, small enough to generate the needed Hawking radiation, while large enough to survive for the duration of a century-long star crossing. Adam Crowl has written fascinatingly about this in Crowlspace.
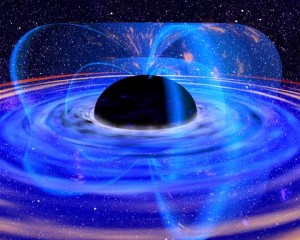
Crane and Westmoreland’s paper on using Hawking radiation for this purpose has been kicking around on the Net for a bit, never quite making it to the top of the queue here, but Marcus Chown gives it a good look in the latest New Scientist, so let’s pause to examine it now. Rather than finding a nearby black hole, the two suggest using a gamma ray laser powered by solar energy to create one. The energy needed would be enormous, calling for solar panels 250 kilometers across in close solar orbit, a Robert Forward-esque engineering challenge.
Image: An artist’s impression of a black hole. Credit: Jörn Wilms (Tübingen) et al/ESA.
But if you could create such solar panels and let them soak up the needed sunlight to power up your black hole production facility, you’d wind up with something tiny that offered tremendous power. Says Chown:
The resulting million-tonne black hole would be about the size of an atomic nucleus. The next step would be to manoeuvre it into the focal range of a parabolic mirror attached to the back of the crew quarters of a starship. Hawking radiation consists of all sorts of species of subatomic particles, but the most common will be gamma ray photons. Collimated into a parallel beam by the parabolic mirror, these would be the starship’s exhaust and would push it forward.
Darwinian Selection Among Universes
Crane and Westmoreland think a starship powered this way could accelerate to close to the speed of light in a few decades, fast enough that relativistic time dilation would occur and vast distances could be crossed by human crews. Interestingly, a black hole starship like this should create gravitational waves that might be detectable here on Earth, assuming some nearby extraterrestrial civilization were using the technology. If coalescing black holes and neutron stars ought to be producing low-frequency gravitational waves, a black hole starship should leave a gravitational signature at ultra-high frequencies.
Chown does a good job with this material, and I recommend you check out his New Scientist article. He points to Lee Smolin’s idea that at the singularity of a black hole, new universes could be created and bud off from their parent. It stands to reason, then, that universes that are optimized for black holes are those likely to give rise to more and more such universes. And if we could make our own black holes, then life would play a role in making infant universes proliferate.
[Crane] believes we are seeing Darwinian selection operating on the largest possible scale: only universes that contain life can make black holes and then go on to give birth to other universes, while the lifeless universes are an evolutionary dead end.
His latest calculations made him realise how uncanny it was that there could be a black hole at just the right size for powering a starship. “Why is there such a sweet spot?” he asks. The only reason for an intelligent civilisation to make a black hole, he sees, is so it can travel the universe.
“If this hypothesis is right,” he says, “we live in a universe that is optimised for building starships!”
I don’t have time this morning to get into Chown’s discussion of Jia Liu (New York University), who has concocted a spacecraft powered by dark matter, but in any case the Jia Liu paper was in queue for next week, so we’ll talk about it then. Talk about living on the speculative edge — we don’t even know what dark matter is at this point! But as fun as these ideas are to kick around, they also let us roam through broad questions of cosmology and physics in ways that can provoke discussion and help us illuminate our current propulsion constraints.
Marcus Chown’s article is “Dark Power: Grand Designs for Interstellar Power,” New Scientist 25 November, 2009 (available online, but get it fast before it disappears behind the magazine’s firewall). The black hole propulsion paper is Crane and Westmoreland, “Are Black Hole Starships Possible,” available online.
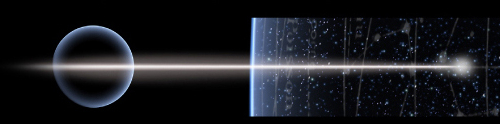
by Paul Gilster | Nov 25, 2009 | Deep Sky Astronomy & Telescopes |
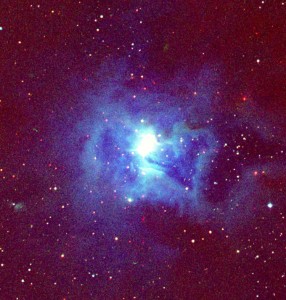
The image at the right shows HD200775, a close binary that contains one star ten times the mass of the Sun. The two stars illuminate the surrounding cavity of molecular gas, the closest of which forms the reflection nebula known as NGC7023. The binary is located some 1400 light years from Earth and is the subject of recent work by an international team that has produced the first well-resolved images of a circumstellar disk around a young, massive star. You can see the disk they found in the mid-infrared in the second image below.
Image: HD200775 and its nebula. The bright star at the center is HD200775, which illuminates the surrounding nebula. The upper and lower part of HD200775 is the reflection nebula NGC7023. The hourglass-shaped nebula extending in the east-west direction (east is left) encircles a cavity formed by past outflow activity. Okamoto et al. zoomed in and imaged an area around HD200775. Credit: DSS/2MASS/Subaru Telescope.
What we’re after is a better understanding of how massive stars — those with masses eight times or more greater than the Sun — originally form. One model is disk accretion, which is the same model we use for low-mass stars like the Sun. Here a cocoon of gas contracts and forms a circumstellar disk, one that may eventually spawn a planetary system. The second model is the merger of less massive stars, but work in the early 2000s has thus far pointed to accretion, and the recent work on HD200775 is telling us much the same story.
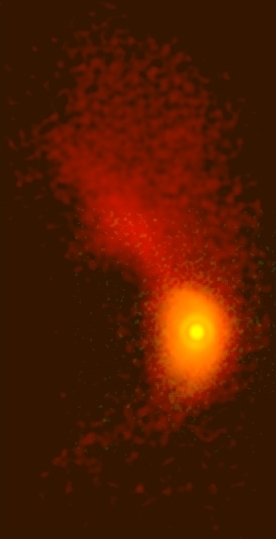
Many images of disks around low mass stars exist that help us understand the process, but no clear images of a disk around a massive star has been available until now. The new work relies on data from the Subaru Telescope fitted with the Cooled Mid-Infrared Camera and Spectrometer (COMICS). What emerges is a circumbinary disk, one that surrounds both stars of the binary system. The disk appears to be flared rather than flat, and it appears that strong ultraviolet radiation from the massive star evaporates the gas of the disk, quickly dissipating it. The conclusion: Accretion is still at work here, but the disk around such a star is likely to be short-lived, which accounts for our difficulty in finding previous examples.
Image: Mid-infrared image of HD200775 taken with COMICS (Cooled Mid Infrared Camera and Spectrometer) mounted on the Subaru Telescope. The detected disk is the yellow portion in the lower right. HD200775 is in the central position, although the infrared emission mostly arises from dust grains in the vicinity of the star, not from the star itself. (Note that the circular ring immediately surrounding the central star is a diffracted light pattern created by the telescope aperture; it is not a real structure.) The elliptical shape stretching in the north-south direction (top to bottom) is a configuration that indicates we are seeing the stars’ system from an angle inclined from a disk pole (perpendicular to the disk). The structure imaged in red and extending toward the northeast reaches out toward the reflection nebula NGC7023. It probably bridges the disk and an envelope (cloud of dust and gas) that has a structure 30 times larger in scale. Credit: Subaru Telescope.
Exactly how short-lived such disks are is an interesting question, for we’d also like to know whether we can expect planet formation around stars of this size. The paper is Okamoto et al., “Direct Detection of a Flared Disk Around a Young Massive Star HD200775 and its 10 to 1000 AU Scale Properties,” Astrophysical Journal 706 (20 November 2009), pp. 665-675 (abstract, preprint available).
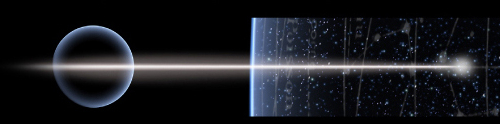
by Paul Gilster | Nov 24, 2009 | Deep Sky Astronomy & Telescopes |
Now that we are looking forward to the WISE mission (Wide-Field Infrared Survey Explorer) and its investigations into nearby brown dwarfs, it’s startling to realize that we detected the first of these objects as recently as 1995. Today they’re all the rage, particularly among that small band of us obsessed with missions to nearby interstellar space. A cool, dim brown dwarf could be the closest star to our Sun, an obvious target for a future probe once long-haul propulsion options begin to mature.
Brown dwarfs are too cool to trigger hydrogen fusion, so it takes infrared capabilities like those of WISE or the Spitzer Space Telescope to tell us much about these dim objects. A key question has been whether they form like planets or stars. Spitzer may have found the answer in the form of two ‘proto brown dwarfs’ that have been located in a cloud called Barnard 213, a region of the Taurus-Auriga complex where young objects abound. The finding is significant because we’ve never before found a clear case of a brown dwarf in its earliest formative period.
David Barrado (Centro de Astrobiologia, Madrid) describes the thinking that went into finding these objects:
“We decided to go several steps back in the process when (brown dwarfs) are really hidden. During this step they would have an (opaque) envelope, a cocoon, and they would be easier to identify due to their strong infrared excesses. We have used this property to identify them. This is where Spitzer plays an important role because Spitzer can have a look inside these clouds. Without it this wouldn’t have been possible.”
The result is an infant brown dwarf called SSTB213 J041757, a find that turns out to include not one but two brown dwarfs that are among the faintest and coolest ever observed. Numerous other sites, from the Caltech Submillimeter Observatory in Hawaii to the Calar Alto Observatory in Spain and the Very Large Array in New Mexico took part in the study, which analyzed the dusty envelope around the objects, allowing astronomers to create a spectral density distribution that shows the amount of energy emitted by the objects in each wavelength.
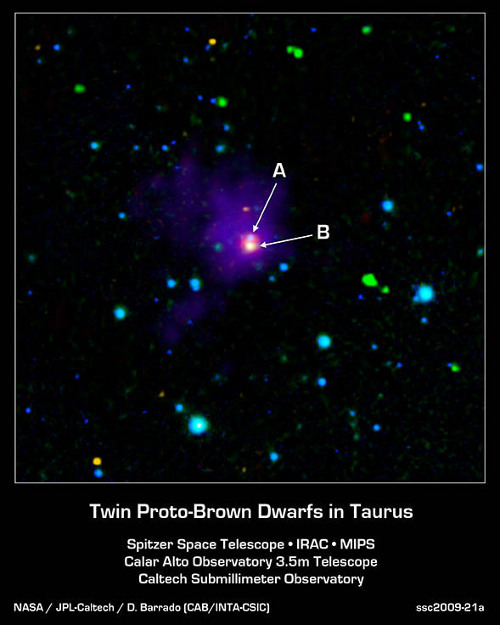
Image: Here we see a long sought-after view of these very young objects, labeled as A and B, which appear as closely-spaced purple-blue and orange-white dots at the very center of this image. The surrounding envelope of cool dust surrounding this nursery can be seen in purple. These twins, which were found in the region of the Taurus-Auriga star-formation complex, are the youngest of their kind ever detected. They are also helping astronomers solve a long-standing riddle: Do brown dwarfs form more like stars or planets? Credit: NASA/JPL-Caltech/D. Barrado (CAB/INTA-CSIC).
Assuming the work stands up to scrutiny, the implication is that brown dwarfs form more like stars than planets — the spectral density distribution matches other young, low-mass stars. The paper, not yet available, is Barrado et al., “A proto brown dwarf candidate in Taurus,” in press at Astronomy & Astrophysics.
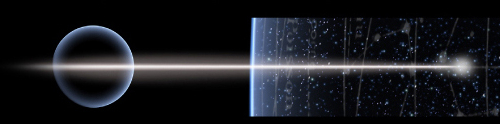
by Paul Gilster | Nov 23, 2009 | Interstellar Medium |
One problem with journeys that are beyond today’s technologies is that we forget, in our zeal to get a payload to the target, how little we know about the regions we’ll pass through along the way. It’s amazing how little we know, for example, about the heliosphere around the Solar System, yet any probe pushing into interstellar space will have to cross from the region of space under the Sun’s influence into a zone where the interstellar medium flows around this ‘bubble,’ disturbing the solar wind and creating a secondary bubble, the heliosheath.
We don’t yet have a global view of what spacecraft will encounter in the heliosheath as the solar wind is heated and slowed by these interactions. Only recently have we gotten the Voyagers into these regions, and in any case these doughty vehicles can only produce single-point measurements. But we’ve got the IBEX (Interstellar Boundary Explorer) spacecraft making observations from near Earth, and now we learn that Cassini, our intrepid Saturn orbiter, has also produced excellent data on what happens at system’s edge.
NASA is now offering an interesting animation of the heliosphere and heliosheath, showing the interstellar medium flowing past, with the interstellar magnetic field moving around the bubble of hot, high pressure particles. The findings that produced this animation are intriguing because it was previously thought that the Solar System moved through the interstellar medium in a shape resembling that of a comet. Instead, scientists using Cassini’s Ion and Neutral Camera sensor on its Magnetospheric Imaging Instrument (MIMI) find that the heliosphere is less like a comet and more like a ball moving through smoke, the ‘smoke’ being the interstellar magnetic field.
This JPL news release uses a more vivid turn of phrase: “…the new results suggest our heliosphere more closely resembles a bubble – or a rat – being eaten by a boa constrictor: as the solar system passes through the “belly” of the snake, the ribs, which mimic the local interstellar magnetic field, expand and contract as the rat passes.”
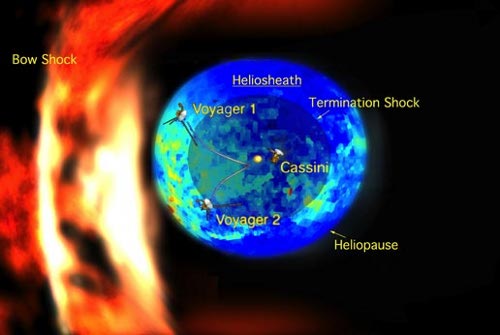
Image: In this illustration, the multicolored (blue and green) bubble represents the new measurements of the emission of particles known as energetic neutral atoms. The energetic neutral atoms were streaming in from the thick boundary known as the heliosheath. The heliosheath is the region between the heliosphere, the region of our sun’s influence, and the interstellar medium, the matter between stars in our galaxy. Areas in red indicate the hottest, most high-pressure regions and purple the coolest, lowest-pressure regions. Credit: NASA/JPL/JHUAPL.
As to the heliosheath itself, the Cassini data indicate it’s somewhere between 40 and 50 AU thick. That’s a remarkable result from a spacecraft that has as its primary mission the investigation of Saturn and its moons. But Cassini’s studies of the energetic electrons and ions trapped in Saturn’s magnetic field, and of the energetic neutral atoms also produced in this environment, have produced a treasure trove of data that includes particles arriving from the outer Solar System.
Usefully, the picture that began to emerge from Cassini squared with what IBEX had already produced, thus deepening our understanding of this outer region. Says MIMI scientist Don Mitchell (JHUAPL):
“I was initially skeptical because the instrument was designed for Saturn’s magnetosphere. But our camera had long exposures of months to years, so we could accumulate and map each particle that streamed through the tiny aperture from the far reaches of the heliosphere. It was luck, but also a lot of hard work.”
The paper is Krimigis et al., “Imaging the Interaction of the Heliosphere with the Interstellar Medium from Saturn with Cassini,” Science, Vol. 326, No. 5955 (13 November 2009), pp. 971-973 (abstract).
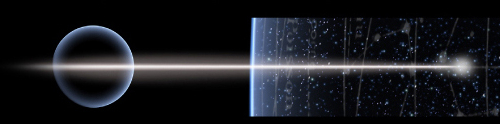