by Paul Gilster | Nov 26, 2019 | Exoplanetary Science |
The interest in ‘Oumuamua and comet 2I/Borisov makes it clear that interstellar neighbors wandering into our system generate loads of media coverage. And why not: Here is a way to study material from another stellar system while remaining within our own. 2I/Borisov, for example, reaches its closest approach to Earth in early December, closing to within roughly 300 million kilometers. Whatever pushed an object like this out of the parent system cannot be known, but we’re likely dealing with gravitational disruption related to planets in the birth system. But more about that in a moment.
For thanks to Yale University astronomers Pieter van Dokkum, Cheng-Han Hsieh, Shany Danieli, and Gregory Laughlin, we have a fine new image of 2I/Borisov. This was taken on November 24 using the W.M. Keck Observatory’s Low-Resolution Imaging Spectrometer in Hawaii. The tail of the comet, according to van Dokkum, is about 160,000 kilometers long. Note the size comparison below to be reminded, as always, of the immensity of the objects we routinely study in the sky. For those of us who occasionally get jaded, here is another corrective.
Image: Left: A new image of the interstellar comet 2l/Borisov. Right: A composite image of the comet with a photo of the Earth to show scale. (Pieter van Dokkum, Cheng-Han Hsieh, Shany Danieli, Gregory Laughlin).
Gregory Laughlin points out that 2I/Borisov is evaporating as it moves through the Solar System, releasing the gas and dust so visible in its tail. Says Laughlin: “Astronomers are taking advantage of Borisov’s visit, using telescopes such as Keck to obtain information about the building blocks of planets in systems other than our own.” So true, and what an opportunity we’re opening up as we begin what will become the more routine study of such objects.
Bear in mind that according to a current Laughlin paper, written with Yale graduate student Malena Rice, we can expect a few such objects showing up every year, with much smaller (and therefore all but impossible to detect) objects coming into the Solar System in the hundreds per year. The duo studied three protoplanetary disks from the Disk Substructures at High Angular Resolution Project (DSHARP). Says Rice:
“We were looking for disks in which it was pretty clear a planet was there. If a disk has clear gaps in it, like several of the DSHARP disks do, it’s possible to extrapolate what type of planet would be there. Then, we can simulate the systems to see how much material should be ejected over time…This is actual material that makes up planets in other solar systems, being flung at us. It’s a completely unprecedented way to study extrasolar systems up close — and this field is going to start exploding with data, very soon.”
The paper on the frequency of interstellar objects is Laughlin & Rice, “Hidden Planets: Implications from ‘Oumuamua and DSHARP,” Astrophysical Journal Letters Vol. 884, No. 1 (10 October 2019). Abstract / Preprint.
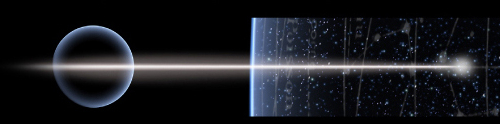
by Paul Gilster | Nov 21, 2019 | Exoplanetary Science |
Our fascination with Alpha Centauri doubtless propels at least some of the recent interest in binary star systems, as we ponder the chances for habitable worlds around the nearest stars. But given that the population of binary or multiple star systems in our galaxy is as high as it is (multiple systems are common, and about 50 percent of stars have binary companions), determining the factors that influence habitability in this environment has much broader significance. A new study out of the Georgia Institute of Technology has been looking at the issue by modeling an Earth twin in various binary scenarios.
So how does Alpha Centauri fare? We can find habitable zones in the Centauri A/B system, and into these the researchers introduced a simulated Earth around Centauri B to examine its axis dynamics. They also investigate the dynamical evolution of planets within the habitable zone of either star, generalizing from these results to the larger binary star population. The issue to be addressed: Does the axial tilt, or obliquity, of our planet, apparently a key feature in maintaining a habitable climate, survive the transition into a binary system with the features of Alpha Centauri? The answer is not encouraging for life, at least not around Centauri B.
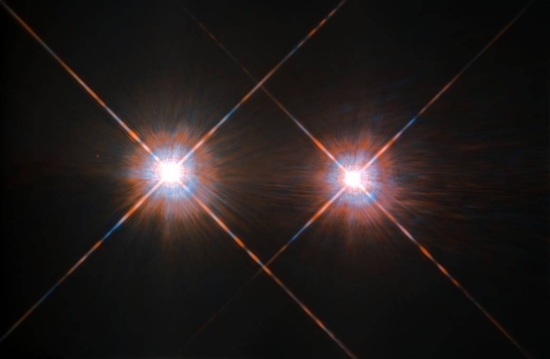
Image: The Alpha Centauri group is the closest star system outside of our own at a distance of 4.3 lightyears; it can be found in the night sky in the constellation Centaurus. The stars Alpha Centauri A and Alpha Centauri B comprise a binary system, in which the two stars orbit one another, and close by is an additional faint red dwarf, Alpha Centauri C, also called Proxima Centauri. Some astronomers have hoped to someday find an exoplanet capable of harboring advanced life in the system, but a new study lowers those expectations while raising them for the rest of the universe. Credit: NASA/ESA Hubble Space Telescope.
Remember that Centauri B, a K-class dwarf, and the G-class Centauri A orbit each other tightly, with an orbital period of 79.91 years. We’re dealing with an elliptical orbit that varies the distance between the two stars anywhere from 35 AU to 11 AU, the latter being not much further than Saturn is from the Sun in our own system. From the perspective of a planet orbiting Centauri B, Centauri A swings relatively close and then backs away during the 80-year period, causing the simulated Earth to vary considerably in terms of obliquity. The Moon is often cited as a help in stabilizing Earth’s axial tilt, but that doesn’t help here, says lead author Billy Quarles.
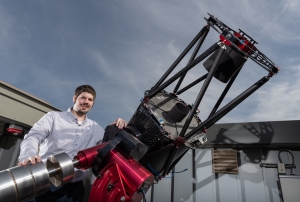
“Around Alpha Centauri B, if you don’t have a moon, you have a more stable axis than if you do have a moon. If you have a moon, it’s pretty much bad news.”
From the paper:
…the added spin precession from a Luna-like moon increases the obliquity variation of an Earth-like planet orbiting ? Cen B, but a different type of moon, in terms of its mass or semimajor axis, may have a negligible or more beneficial effect.
Image: Astrophysicist Billy Quarles, author of a new study on exoplanet axis tilt, stands with Georgia Tech’s largest telescope housed at its observatory. Credit: Georgia Tech / Rob Felt.
And without a moon? Quarles again:
“The biggest effect you would see is differences in the climate cycles related to how elongated the orbit is. Instead of having ice ages every 100,000 years like on Earth, they may come every 1 million years, be worse, and last much longer.”
The separation of the two stars is the key here. According to this work, Centauri A and B are simply too close for comfort, but a wider separation, which holds in most binary systems, would allow the second star’s effects to be less disruptive to the simulated Earth. I was curious about the effects of a moon, though, and went further into the paper, which notes that the disruptive effects of a moon on a simulated Earth around Centauri B are:
…in contrast to our own Earth-based expectations, where our moon does the opposite (Laskar et al. 1993b). The amount of spin precession from a moon depends on the moon’s mass and semimajor axis, where a Pluto-mass moon at a Luna-like semimajor axis aluna would have a negligible effect. A smaller semimajor axis (0.2 aluna) would allow a Pluto-mass moon to increase the spin precession and allow for larger obliquity variations. The degree to which a moon can increase the overall spin precession depends on many factors, where they are neither needed nor necessarily even desirable to obtain relatively low obliquity variations.
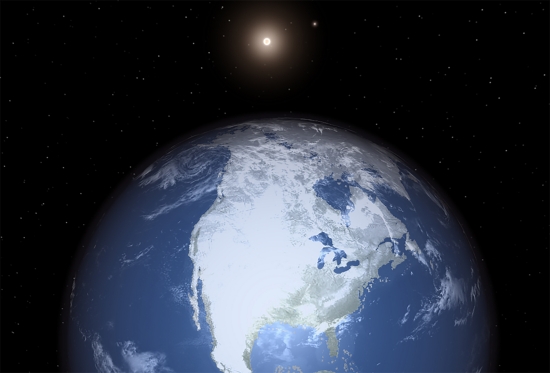
Image: Modeled into an orbit in the habitable zone around Alpha Centauri B, in this artist’s rendering by an author of a new study, our planet appears rather icy and inhospitable to advanced life. Credit: Georgia Tech / Billy Quarles.
Quarles points to Mars as an example of obliquity extremes influencing climate. The axial tilt of Mars varies between 10 and 60 degrees every 2 million years, as opposed to Earth’s axial tilt (between 22.1 and 24.5 degrees over a course of 41,000 years). Earth’s Moon stabilizes our planet’s obliquity, which would otherwise be affected by gravitational influences from the inner planets as well as Mars and Jupiter.
If the precession of Mars’ axis seems to have helped deplete its atmosphere, imagine an Earth precessing the same 60 degrees, which is the figure Quarles deduces for an Earth without its Moon. Clearly, the presence of a moon can have widely varying effects depending on the stellar masses and orbital parameters involved. We learn that the presence of a single large moon is just one factor in questions of habitability, and its effects are not always benign.
But the broader outlook for habitable zone planets in binary star systems seems encouraging. According to the study, a high percentage of such systems can support exo-Earths with axial tilts similarly steady to Earth’s, thus ensuring climate stability. Back to the paper:
We combine our results with population studies of binary stars (Raghavan et al. 2010; Moe & Di Stefano 2017) and find the chance that an Earth-like rotator orbiting the primary star would experience small (< 2.4°) obliquity variations is 87%, 74%, or 54%, depending on the mass of the primary (0.8, 1.0, or 1.2 M? Solar-Type stars, respectively).
All told, those aren’t bad numbers given the number of multiple star systems throughout the Milky Way. The paper shows us that the evolution in axial tilt for a single Earth-like planet in the habitable zone of a binary system depends on the orbital precession induced by the companion star, while also being influenced by orbital distance, nearby terrestrial planets and moons. It’s interesting, too, to see that many disks are misaligned by around 10 percent from the binary plane, which would produce a typical obliquity variation in the neighborhood of 20%. Clearly, orbiting nearly planar with the binary would be the most desirable place to be.
It’s worth noting that the Transiting Exoplanet Survey Satellite (TESS) is expected to uncover ?500,000 eclipsing binary systems. We’ll have no shortage of candidates for further study. Both TESS and Gaia observations, the authors say, would allow a more robust statistical approach to emerge as we examine the obliquity of planets around Sun-like stellar binaries.
The paper is Quarles et al., “Obliquity Evolution of Circumstellar Planets in Sun-like Stellar Binaries,” Astrophysical Journal Vol. 886, No. 1 (19 November 2019). Abstract / Preprint.
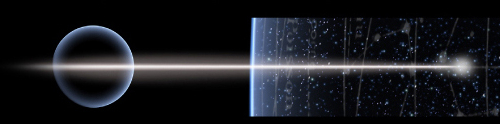
by Paul Gilster | Nov 19, 2019 | Outer Solar System |
When I think about mapping new places, I remember Vincent Van Gogh, who once said “To look at the stars always makes me dream, as simply as I dream over the black dots of a map representing towns and villages. Why, I ask myself, should the shining dots of the sky not be as accessible as the black dots on the map of France?”
Why not indeed? The exciting process of mapping new worlds continues to take place as we pursue our reconnaissance of the Solar System, now pushing well into the Kuiper Belt. Mapping Saturn’s giant moon Titan is particularly satisfying, because as you’ll recall, Voyager 1 was diverted from its original trajectory because Titan was just too interesting a target to miss. No Titan mapping then, though we did have useful scientific results, because Voyager 1 saw a world shrouded in orange smog. Cassini changed the game, of course, and now we’re looking deep beneath the clouds.
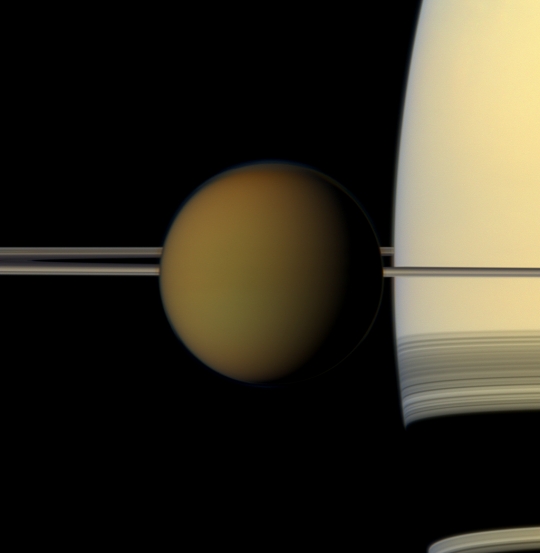
Image: The colorful globe of Saturn’s largest moon, Titan, passes in front of the planet and its rings in this true color snapshot from NASA’s Cassini spacecraft. The north polar hood can be seen on Titan (5,150 kilometers across) and appears as a detached layer at the top of the moon here. This view looks toward the northern, sunlit side of the rings from just above the ring plane. Images taken using red, green and blue spectral filters were combined to create this natural color view. The images were obtained with the Cassini spacecraft narrow-angle camera on May 21, 2011, at a distance of approximately 2.3 million kilometers from Titan. Image scale is 14 kilometers per pixel on Titan. Credit: NASA/JPL-Caltech/Space Science Institute.
Cassini made something like 120 flybys of Titan in the course of its long campaign at Saturn between 2004 and 2017, using its radar imager to penetrate the atmosphere of nitrogen and methane, while supplementing these views with data from visible and infrared instruments that could make out the larger geological features of the Mercury-sized world. Now Rosaly Lopes (JPL) has led a team of researchers in producing the first map that shows Titan’s global geology, pulling together all of these data to reveal a wide range of terrains. Says Lopes:
“This study is an example of using combined data sets and instruments. Although we did not have global coverage with synthetic aperture radar (SAR), we used data from other instruments and other modes from radar to correlate characteristics of the different terrain units, so we could infer what the terrains are even in areas where we don’t have SAR coverage.”
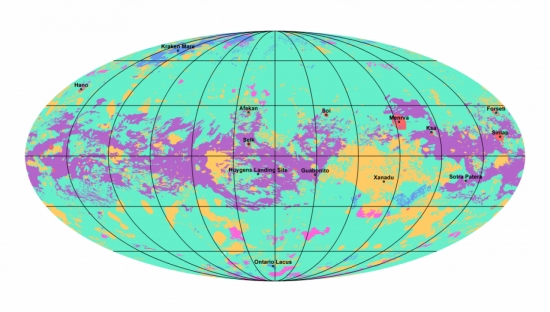
Image: The first global geological map of Saturn’s largest moon, Titan, is based on radar and visible and infrared images from NASA’s Cassini mission, which orbited Saturn from 2004 to 2017. Labels point to several of the named surface features. Also located is the landing site of the European Space Agency’s Huygens Probe, part of NASA’s Cassini mission. Credit: NASA/JPL-CalTech/ASU.
When we turn Titan into a map, we begin to sense how many features on the surface resemble similar features on Earth, evidencing similar geological processes at work, with methane/ethane being the operative fluid here. We’re seeing a hydrologic cycle active on a surface rich in hydrocarbon rain, a place segmented by rivers and punctuated by lakes and seas. It’s interesting to see, by the way, that David Williams (Arizona State), who was a major player in extrapolating radar images into areas not covered by radar, is also the man who will make the first global geologic map of (16) Psyche on a mission scheduled for a 2022 launch. For more on that one, see Psyche Mission Moved Up, a 2017 Centauri Dreams article.
The paper is Lopes et al., “A global geomorphologic map of Saturn’s moon Titan,” Nature Astronomy 18 November 2019 (abstract).
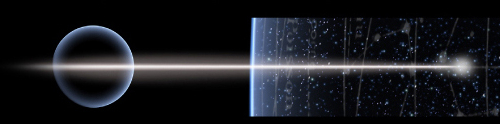
by Paul Gilster | Nov 18, 2019 | Sail Concepts |
The electric sail is an intriguing propulsion concept that Pekka Janhunen at the Finnish Meteorological Institute has been championing for some years. It’s currently the subject of a NASA Phase II study and continues to draw attention despite the fact that we’re in the early stages of turning what looks like sound physical theory into engineering. What captures the imagination here is the same thing that is so attractive about solar sails — in both cases, we are talking about carrying no propellant, but instead relying on natural sources to do the work.
Here we have to be careful about terminology, because it’s all too easy to refer to solar photons as a kind of ‘wind,’ especially since the predominant metaphor is sailing. So let’s draw the lines sharply. There is indeed a ‘solar wind’ in today’s parlance, but it refers not to light but to the stream of particles, plasma and magnetic fields flowing out from the Sun into the heliosphere. An electric sail will ride this solar wind to achieve interplanetary velocities. A solar sail, on the other hand, will use solar photons, which carry no mass but do convey momentum.
Two entirely different concepts, even if both have resemblance to traditional nautical sails. Then we have the other terminological complication: A sail designed to be pushed not just by sunlight but rather by a laser or microwave beam is sometimes called a ‘light sail,’ which is how I have always referred to it, but it still uses photons for propulsion, even if they don’t come from the Sun. Maybe Manasvi Lingam and Avi Loeb have it right in their new paper to refer to photon-pushed sails of any kind as ‘light sails,’ distinguishing these from both electric and magnetic sails (magsails) that use the ‘solar wind’ as their driver. Thus:
Light sails — solar sails and those driven by beamed arrays — use electromagnetic radiation and the momentum transfer of photons. Electric sails use the particle stream of the solar wind.
The electric sail that Janhunen continues to study is the subject of Lingam and Loeb’s new paper, which has been submitted to Acta Astronautica. At the Florida Institute of Technology and Harvard University respectively, the two scientists have calculated performance possibilities for a spinning spacecraft that deploys a number of long wires to which an electrostatic charge has been induced. Solar wind protons (not photons!) reflect off these wires to produce thrust. The wires are kilometers long, and with that slight positive bias, the spacecraft carries an electron gun to manage the charge, retaining the bias against ambient solar wind electrons.
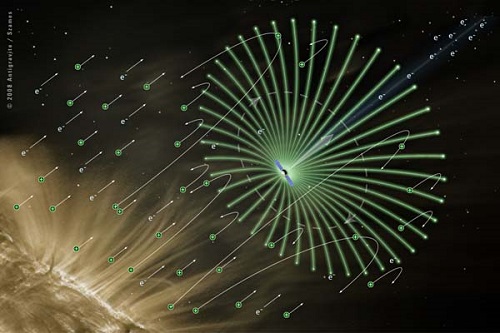
Image: The electric sail is a space propulsion concept that uses the momentum of the solar wind to produce thrust. Credit: Alexandre Szames.
Light sails, to use the Lingam and Loeb terminology, have been considered for interstellar missions for decades now (hats off to the early work of Robert Forward, Gregory Matloff and Geoff Landis, among others), but electric sails are new enough that we need information on how well an electric sail might do for this purpose. Could this technology get us to another star?
For a species like ours, anxious to see missions completed within a few human lifetimes, the answer is no. While a huge laser array like the one contemplated by Breakthrough Starshot could send a small light sail at relativistic speeds to another star, the electric sail cannot achieve the needed velocities.
A species with a different attitude toward time might fare better. The paper explains, for example, how electric sails could leverage the stellar winds of red dwarf stars, which are by far the most common kind of star in the galaxy. Because the interstellar medium itself can decelerate the sail, turning off the electron gun in deep space is essential. Careful maneuvering from star to star over millennia then allows relativistic speeds. From the paper:
…a series of repeated encounters with low-mass stars, and taking advantage of their winds, will enable the electric sail to achieve progressively higher speeds. We showed that sampling ? 104 stars could enable electric sails to achieve relativistic speeds of ? 0.2 c and that this mechanism would require ? 1 Myr. While this constitutes a long timescale by human standards, it is not particularly long in comparison to many astronomical and geological timescales. The ensuing relativistic spacecraft would be well-suited for tackling interstellar and even intergalactic exploration.
This is an eye-opener. We can’t rule out the possibility that species capable of operating in this time frame might deploy electric sails, but the time involved precludes their use as the primary propulsion method for interstellar missions by us. The authors note as well that because an electric sail will have a low cross-sectional area, its presence would be all but undetectable, whereas a light sail driven by a laser would demand huge amounts of energy and would be theoretically detectable at interstellar distances. So for a civilization hoping to explore in ‘stealth’ mode, an electric sail would have its advantages. These are not good SETI targets.
Returning to M-dwarf stars, the authors show that if stars are small enough (less than about 0.2 solar masses), the pressure of the stellar wind dominates over photon pressure, Speeds in the range of 500 kilometers per second seem feasible for electric sails near late-type M-dwarfs. Indeed, for F-, G- and K-class stars, electric sails fare better as propulsion systems in the vicinity of the home star than light sails.
So we are looking at a technology that, if it can be properly engineered, could play a role in shaping an interplanetary infrastructure, while yielding to faster methods for missions to other stars, unless we humans somehow attain an all but geological patience.
The paper is Lingam and Loeb, “Electric sails are potentially more effective than light sails near most stars,” in process at Acta Astronautica (preprint). For Pekka Janhunen’s concept of the electric sail as a fast interplanetary probe, see Electric Sails: Fast Probe to Uranus.
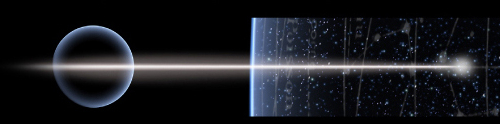
by Paul Gilster | Nov 15, 2019 | Exoplanetary Science |
While the majority of exoplanet-hosting stars discovered so far are single, we do have multiple star systems in various configurations with planetary companions. This is fertile ground for study, and not just because the nearest stellar system, Alpha Centauri, contains a tight binary pair that is being closely investigated for planets. The third star here is, of course, Proxima Centauri, around which we already know of the existence of a planet in the habitable zone. The much broader question is, how likely are multiple star systems to host planets?
Tackling this question in a new study is Markus Mugrauer (Friedrich Schiller University, Jena), who has been investigating how the existence of multiple stars in a system affects the formation and development of planets. Mugrauer has been working with the second data release from the European Space Agency’s Gaia mission (made available in April of last year). This release contains data collected by Gaia during the first 22 months of its mission. Mugrauer’s survey searched for stellar companions of 1367 exoplanet host stars within about 500 parsecs of the Sun, or roughly 1600 light years, as listed in the Extrasolar Planets Encyclopedia.
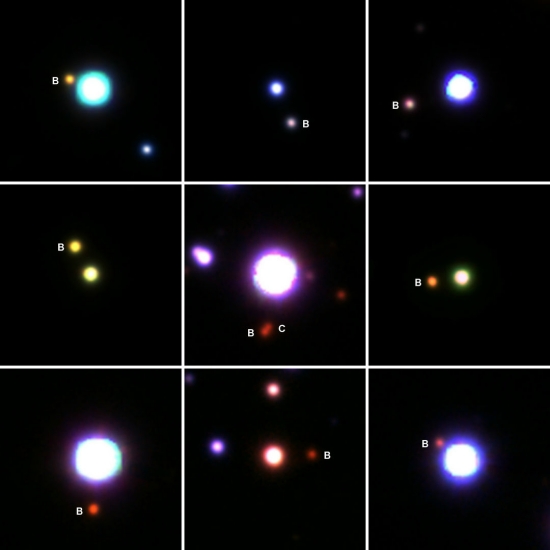
Image: These images show some of the exoplanet host stars with companion stars (B, C) that were found during the project. The images are RGB composite images taken with the Panoramic Survey Telescope and Rapid Response System (PanSTARRS) in the y- (960 nm), i- (760 nm), and g-band (480 nm). The image in the middle shows a hierarchical triple star system. Credit: Markus Mugrauer, PanSTARRS.
207 companion stars turn up in this work, varying strongly in mass, temperature and stage of stellar evolution. Of these, 176 are found to be binaries, 27 triple star systems, and one is a quadruple star system, which produces a multiplicity rate of about 15 percent. In each case, Mugrauer uses the Gaia data to demonstrate that the host stars are equidistant and share a common proper motion.
The heaviest of the companion stars weighs 1.4 solar masses; the lightest is a scant 8 percent of the Sun’s mass. As might be expected from their ubiquity, most companion stars turn out to be low-mass, cool red dwarf stars, although it’s interesting to note that eight white dwarfs are also found among the faint stellar companions. The projected separation between these exoplanet hosting systems ranges from 20 AU to 9100 AU, with the highest frequency being within 1000 AU (by comparison, the mean separation of Centauri A and B is 23 AU).
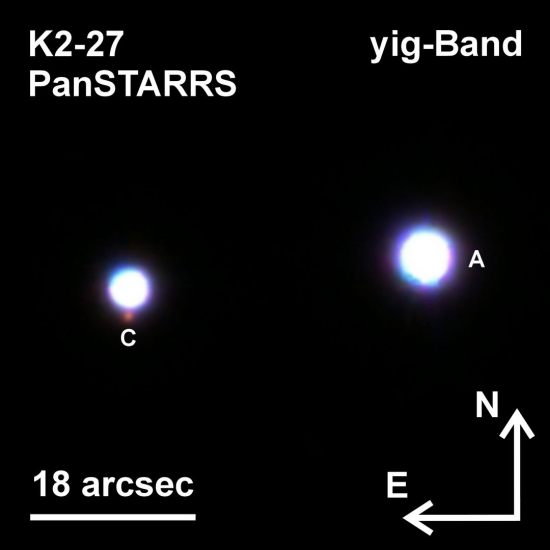
Image; A triple star system approx. 800 light years from the Earth in the Leo constellation with the planetary host star K2-27 (bright star on the left). The image is an RGB composite image taken with PanSTARRS in the y- (960 nm), i- (760 nm), and g-band (480 nm). To the right of it, the first companion star (A) can be clearly distinguished. Just below K2-27 is the second companion star (C) that glows faintly red. Credit: Markus Mugrauer, PanSTARRS.
The results point to what is likely the disruptive influence of several stars in a system where planets are forming, for Mugrauer’s 15 percent incidence of multiple star systems contrasts with the frequency of multiple systems in general. From the paper;
In order to compare the companion star fraction found in this study among exoplanet host stars to that of the solar like stars in general one has to consider only the range of projected separation of the companions detected here, i.e. 19 up to the applied search radius of 10?000?au. This requires the determination of the orbital periods of the detected companions, following the procedure, as described by Raghavan et al. (2010) for wide companions…
Working these calculations, Mugrauer comes up with the following:
The range of separation 19 up to 10?000?au corresponds to orbital periods log(P[d]) = 4.59–8.67. According to the period distribution of companions of solar like stars for this range of period one expect[s] a companion star fraction of 30 ± 2? per?cent, which is about twice as large as the fraction found in the study, presented here, among exoplanet host stars.
In other words, the frequency of exoplanet-hosting multiple star systems is about half what we would expect for solar-like stars in general, and the distances between companion and primary star in exoplanet systems are roughly five times greater than in ordinary systems. We may be looking at the gravitational influence of the companion on the gas and dust disk out of which planets emerge. Says Mugrauer: “These two factors taken together could indicate that the influence of several stars in a star system disrupts the process of planet formation as well as the further development of their orbits.”
Mugrauer’s work is continuing via an international observing campaign being conducted at the Paranal Observatory of the European Southern Observatory in Chile, which will apply data from Gaia to more precisely characterize newly discovered planetary host stars and their companions.
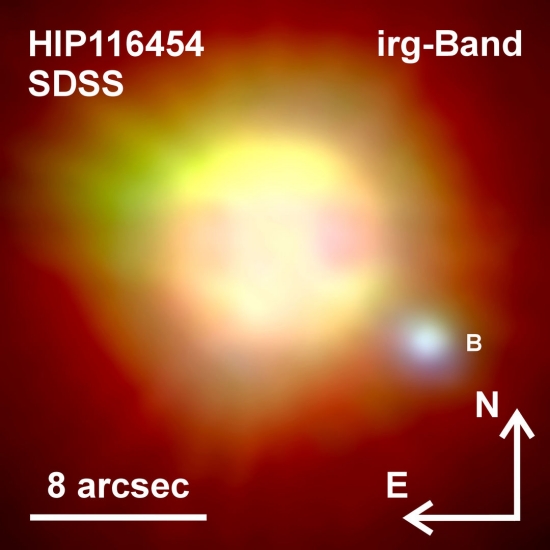
Image: HIP116454 is a planetary host star in the Pisces constellation and it is approx. 200 light years from the Earth. The star is accompanied by a significantly fainter white dwarf (B). The image is an RGB composite image composed of images taken in the i- (760 nm), r- (620 nm), and g-band (480 nm) as part of the Sloan Digital Sky Survey (SDSS). Credit: Markus Mugrauer, SDSS.
The paper is Mugrauer, “Search for stellar companions of exoplanet host stars by exploring the second ESA-Gaia data release,” Monthly Notices of the Royal Astronomical Society 13 November 2019 (full text).
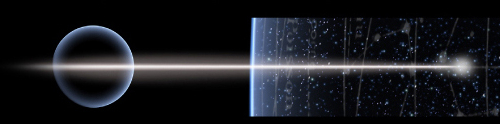