by Paul Gilster | Jul 30, 2021 | Deep Sky Astronomy & Telescopes |
The first direct observation of light from behind a black hole has just been described in a paper in Nature. What is striking in this work is not so much the confirmation, yet again, of Einstein’s General Relativity, but the fact that we can observe the effect in action in this environment. Having just read Heino Falcke’s Light in the Darkness: Black Holes, the Universe, and Us (HarperOne 2021), I have been thinking a lot about observing what was once thought unobservable, as Falcke and the worldwide interferometric effort called the Event Horizon Telescope managed to do when they produced the first image of a black hole.
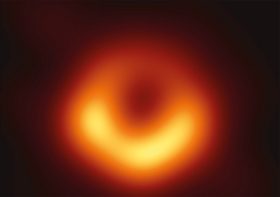
The famous image out of that work that went worldwide in the media was of the supermassive black hole at the center of the galaxy M87, while the new paper — which offers no image but rather data on telltale X-ray emissions — covers a galaxy called I Zwicky 1 (I Zw 1), a Seyfert galaxy 800 million light years from the Sun. These are active galaxies with supermassive black holes at their centers and quasar-like nuclei. The data on this one were drawn from observations of I Zw 1 in early 2020 by the X-ray telescopes NuSTAR and XMM-Newton.
Image: The image of the black hole at the center of M87 shows the effect of the accretion disc as well as the black hole’s shadow. Credit: Akiyama et al. and ApJL.
Dan Wilkins, lead author of the paper on this work in Nature, is a research scientist at the Kavli Institute for Particle Astrophysics and Cosmology at Stanford and SLAC National Accelerator Laboratory. He explains how the malleability of spacetime makes the observations of I Zw 1’s black hole possible:
“Any light that goes into that black hole doesn’t come out, so we shouldn’t be able to see anything that’s behind the black hole. The reason we can see that is because that black hole is warping space, bending light and twisting magnetic fields around itself.”
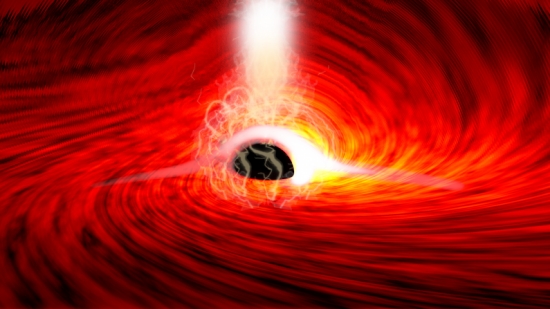
Image: Researchers observed bright flares of X-ray emissions, produced as gas falls into a supermassive black hole. The flares echoed off of the gas falling into the black hole, and as the flares were subsiding, short flashes of X-rays were seen – corresponding to the reflection of the flares from the far side of the disk, bent around the black hole by its strong gravitational field. Credit: Dan Wilkins.
Material moving in the innermost regions of the accretion disk around a supermassive black hole forms a compact and variable corona of X-ray light near the object that allows scientists to map and characterize the accretion disk. Here superheated gas creates a magnetized plasma caught in the black hole’s spin. The magnetic field twists around itself, eventually breaking. The X-rays are reflected from the accretion disk, which gives us a look at events just outside the black hole’s event horizon.
All of this fits current thinking about how the black hole corona forms, but the researchers then detected a series of smaller X-ray flashes, reflections from the inner part of the accretion disk with calculable reverberation time delays. Wilkins calls these ‘echoes’ because they are the same X-ray flares but reflected from the back of the disk. In essence, this gives us the first information we have to characterize the far side of a black hole, and follows from Wilkins’ earlier research. The scientist adds:
“This magnetic field getting tied up and then snapping close to the black hole heats everything around it and produces these high energy electrons that then go on to produce the X-rays. I’ve been building theoretical predictions of how these echoes appear to us for a few years. I’d already seen them in the theory I’ve been developing, so once I saw them in the telescope observations, I could figure out the connection.”
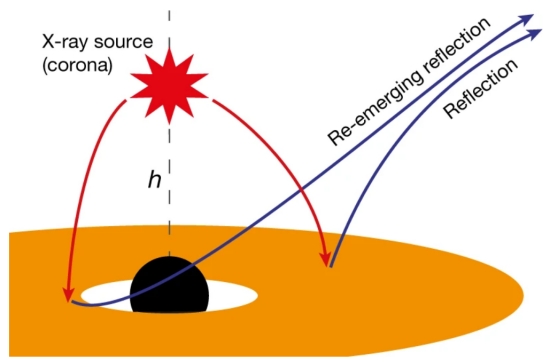
Image: This is Figure 2 from the paper, labelled “Schematic of the X-ray reverberation model.” Caption: X-rays are emitted from a corona of energetic particles close to the black hole. Some of these rays reach the observer directly, but some illuminate the inner regions of the accretion disk and are observed reflected from the disk. Strong light bending in the gravitational field around the black hole focuses the rays towards the black hole and onto the inner regions of the disk. Rays reflected from the back of the disk can be bent around the (spinning) black hole, allowing the re-emergence of X-rays from parts of the disk that would classically be hidden behind the black hole. Credit: Wilkins et al.
What I find extraordinary is that we are getting a glimpse of the accretion disk at work to a fine level of detail. The flares in this black hole’s corona “reveal the temporal response of the illuminated accretion disk,” as the paper puts it, going on to explain the strong Doppler effects at play here:
Emission line photons from different parts of the disk experience different Doppler shifts, due to the variation in the line-of-sight velocity across the disk, and also experience gravitational redshifts, which increase closer to the black hole. The energy shifts of the line photons therefore contain information about the positions on the accretion disk from which they were emitted. The light travel time varies according to the distance of each part of the disk from the corona, and the line emission at different energy shifts is expected to respond to the flare at different times.
Data from the coronal flares and their reverberations allow the team to measure the height of the X-ray source above the disk and also to measure the mass of the black hole itself, which turns out to be on the order of 30 million solar masses. The authors go into detail about the factors at play in the mass estimate, and as the paper is available online, I’ll leave that analysis for those interested in following it up themselves.
Once again black holes provide a way of seeing what had been thought unobservable. Co-author Roger Blandford, also at Stanford, takes note of how far we’ve come:
“Fifty years ago, when astrophysicists started speculating about how the magnetic field might behave close to a black hole, they had no idea that one day we might have the techniques to observe this directly and see Einstein’s general theory of relativity in action.”
Pushing yet deeper will be the European Space Agency’s X-ray observatory Athena (Advanced Telescope for High-ENergy Astrophysics). Wilkins is part of the team developing the Wide Field Imager detector for the telescope, scheduled for launch in 2031.
The paper is Wilkins et al., “Light bending and X-ray echoes from behind a supermassive black hole,” Nature 595 (2021), 657-660 (abstract).
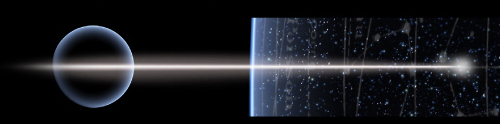
by Paul Gilster | Jun 16, 2021 | Deep Sky Astronomy & Telescopes |
About 1,000 light years away in the constellation Perseus, the stellar nursery designated NGC 1333 is emerging as a priority target for astronomers planning to use the James Webb Space Telescope. Brown dwarfs come into play in the planned work, as do the free-floating ‘rogue’ planets we discussed recently. For NGC 1333 is a compact, relatively nearby target, positioned at the edge of a star-forming molecular cloud. It’s packed with hundreds of young stars, many of them hidden from view by dust, a venue in which to observe star formation in action.
Hoping to learn more about very low mass objects, Aleks Scholz (University of St Andrews, UK) lays out plans for using JWST to chart the distinctions between objects that emerge out of gravitational collapse of gas and dust clouds, and objects that grow through accretion inside a circumstellar disk. Says Scholz:
“The least massive brown dwarfs identified so far are only five to 10 times heftier than the planet Jupiter. We don’t yet know whether even lower mass objects form in stellar nurseries. With Webb, we expect to identify cluster members as puny as Jupiter for the first time ever. Their numbers relative to heftier brown dwarfs and stars will shed light on their origins and also give us important clues about the star formation process more broadly.”
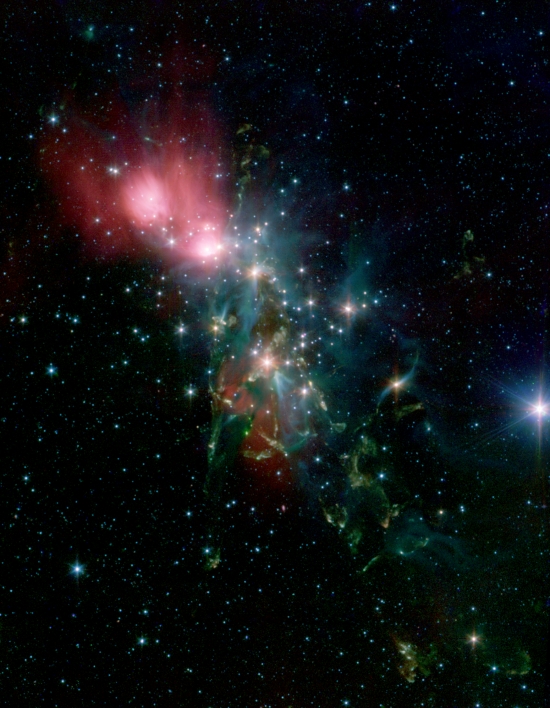
Image: Scientists will use Webb to search the nearby stellar nursery NGC 1333 for its smallest, faintest residents. It is an ideal place to look for very dim, free-floating objects, including those with planetary masses. Credit: NASA/JPL-Caltech/R. A. Gutermuth (Harvard-Smithsonian CfA).
Flying aboard JWST is an instrument called the Near Infrared Imager and Slitless Spectrograph (NIRISS), which Scholz and colleagues will use to analyze the temperature and composition of low-mass objects like these. It is the absorption signature of a particular object, especially water and methane molecules, that will be critical for the work. The advantage of the NIRISS instrument is that it can provide simultaneous spectrographic data on dozens of objects, shortening and simplifying the observational task. One of Scholz’ team, Ray Jayawardhana (Cornell University) has been involved in JWST instrumentation since 2004, and was active in the design and development of NIRISS.
Unable to sustain hydrogen fusion, a brown dwarf may have a mass between 1% and 8% that of the Sun. Most light emitted by these objects is in the infrared, and the already tricky targets are at the top of the size range in this study. Investigating free-floating planets takes us to another level, and even with that in mind, the distinction between a brown dwarf and a giant planet can be blurry. Koraljka Muzic (University of Lisbon), also on Scholz’ team, explains:
“There are some objects with masses below the 10-Jupiter mark freely floating through the cluster. As they don’t orbit any particular star, we may call them brown dwarfs, or planetary-mass objects, since we don’t know better. On the other hand, some massive giant planets may have fusion reactions. And some brown dwarfs may form in a disk.”
Looking through Scholz’ publication list, I noticed a recent paper (“Size and structures of disks around very low mass stars in the Taurus star-forming region” — citation below) that notes the challenge to planet formation models posed by the structure of disks around such stars.
In particular, several giant planets have been found around brown dwarfs, leaving open the question of whether they formed as binary companions or as planets. If the latter, models of planetesimal accretion are hard pressed to explain the process in this environment. The movement of dust presents a problem:
Different physical processes lead to collisions of particles and their potential growth, such as Brownian motion, turbulence, dust settling, and radial drift… All of these processes have a direct or indirect dependency on the properties of the hosting star, such as the temperature and mass. For instance, from theoretical calculations, settling and radial drift are expected to be more efficient in disks around VLMS [Very Low Mass Stars] and BDs [Brown Dwarfs], with BD disks being 15-20% flatter and with radial drift velocities being twice as high or even more in these disks compared to T-Tauri disks…. With radial drift being a more pronounced problem in disks around BDs and VLMS, it is still unknown how this barrier of planet formation is overcome in these environments where the disks are more compact, colder, and have a lower mass.
The paper on the Taurus star-forming region draws on data from ALMA (Atacama Large Millimeter/submillimeter Array), and notes the problems that we can hope JWST will alleviate:
Detection rate of substructures: millimeter dust substructures were directly detected in only 50% of the targets in our sample. Our results suggest that the detection of substructures in disks around VLMS is limited by angular resolution and sensitivity, since the dust radial extent is very small and these disks are also very faint. Deep, high angular resolution observations over a non-brightness biased sample of VLMS should confirm the ubiquity of substructures in these disks.
This is going to be an exciting area of research. As the paper points out, for every ten stars that form in our galaxy, somewhere between two and five brown dwarfs also form, and we already know that low-mass M-dwarfs account for as much as 75 percent of the Milky Way’s stars. When massive objects form around or near brown dwarfs, we are challenged to adjust our models of interactions within the disk and re-consider models of gravitational collapse. Interesting brown dwarf issues await JWST if we can just get it into operation.
The Scholz paper cited above is “Size and structures of disks around very low mass stars in the Taurus star-forming region,” Astronomy & Astrophysics Vol. 645, A139 (January 2021). Abstract.
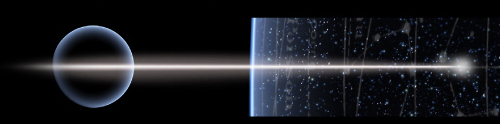
by Paul Gilster | Jun 10, 2021 | Deep Sky Astronomy & Telescopes |
Hard to believe that Fast Radio Bursts (FRBs) were only discovered in 2007, as it seems we’ve been puzzled by them for a lot longer. Thus far about 140 FRBs have been detected, but now we have news that the Canadian Hydrogen Intensity Mapping Experiment (CHIME) has pulled in a total of 535 new fast radio bursts in its first year of operation between 2018 and 2019. The catalog growing from this work was presented this week at the annual meeting of the American Astronomical Society.
“Before CHIME, there were less than 100 total discovered FRBs; now, after one year of observation, we’ve discovered hundreds more,” says CHIME member Kaitlyn Shin, a graduate student in MIT’s Department of Physics. “With all these sources, we can really start getting a picture of what FRBs look like as a whole, what astrophysics might be driving these events, and how they can be used to study the universe going forward.”
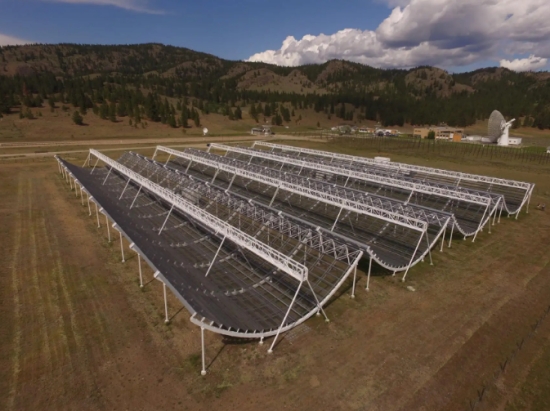
Image: The large radio telescope CHIME, pictured here, has detected more than 500 mysterious fast radio bursts in its first year of operation, MIT researchers report. Credit: Courtesy of CHIME.
CHIME involves four cylindrical radio antennas that MIT describes as “roughly the size and shape of snowboarding half-pipes” located in British Columbia, and operated by the National Research Council of Canada. A correlator instrument — a digital signaling processor — digs through data from the stationary array at a rate of 7 terabits per second, allowing it to detect FRBs at a thousand times the pace of conventional radio telescopes.
We learn, for one thing, that FRBs are common, and frequent. Kiyoshi Masui (MIT) presented the catalog to conference goers on Wednesday the 9th:
“That’s kind of the beautiful thing about this field — FRBs are really hard to see, but they’re not uncommon. If your eyes could see radio flashes the way you can see camera flashes, you would see them all the time if you just looked up.”
It becomes clear from these data that the FRBs detected in the first year were evenly distributed in space, appearing in all parts of the sky. Their rate is thus far calculated to be 800 per day across the entire sky, a figure that is considered the most precise estimate of the phenomena’s occurrence that has yet been presented. Most bursts appear to have originated within distant galaxies, meaning they were highly energetic.
Two categories of FRB also emerge: Those that repeat and those that do not. 18 of the CHIME sources do repeat, with the rest one-time events. Among the repeating signals, each burst lasts slightly longer and emits more focused radio frequencies then bursts from single, non-repeating FRBs. We seem to be looking at two different kinds of astrophysical sources, or at least separate mechanisms, and it will be a goal of future data collection to clarify the differences between the two.
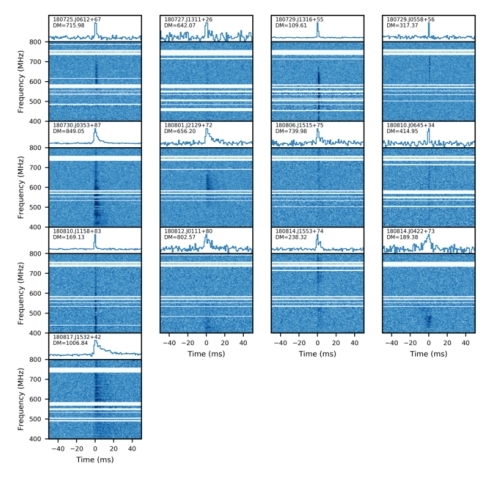
Image: The first 13 FRBs found by CHIME/FRB (from CHIME/FRB Collaboration, 2019, Nature, 566, 230). In this plot, the effects of dispersion have been removed from each source. Credit: CHIME.
While researchers work to learn what could cause such bright, fast signals, it’s fascinating to compare the FRB work with the use of supernovae as ‘standard candles.’ Evidence for the accelerating expansion of the universe was found by such measurements. Can FRBs be used as standard candles for other kinds of detections? Each FRB yields information about its propagation in terms of how gas and matter are distributed along the way to us. Kaitlyn Shin refers to the possibility of using them as “cosmological probes,” a potential enhanced by this new and growing catalog.
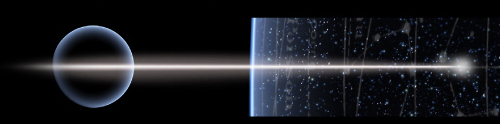
by Paul Gilster | Jun 4, 2021 | Deep Sky Astronomy & Telescopes |
If we assume that the Oort Cloud, that enveloping shroud of comets that surrounds our Solar System and extends to 100,000 AU or beyond, is a common feature of stellar systems, then it’s conceivable that objects are interchanged between the Sun and Alpha Centauri where the two clouds approach each other. That makes for the ‘slow boat to Centauri’ concept I’ve written about before, where travel between the stars essentially mines resources along the way in migrations lasting thousands of years. The resulting society would not be planet-oriented.
When the Dutch astronomer Jan Hendrik Oort deduced the cloud’s existence, he theorized that there was an inner, disk-shaped component as well as an outer, spherical cloud, as shown in the image below. The outer cloud is only loosely bound to the Sun, making the interchange of cometary materials between stars a likely event over the aeons, while gravitational nudges from passing stars can dislodge comets in the other direction as well, causing them to move toward the inner system. Most long-period comets probably come from the Oort Cloud.
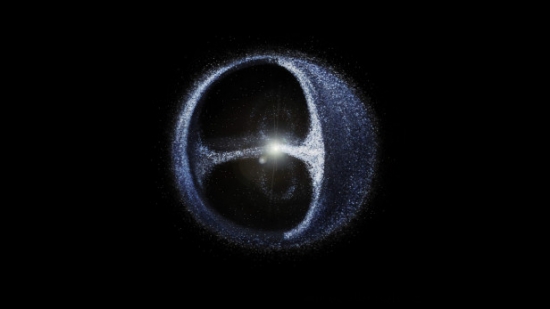
Image: Artist’s impression of the Oort cloud. The density has been hugely exaggerated. (c) Pablo Carlos Budassi [CC BY-SA 4.0] via Wikimedia.
I should also mention that we can find the Oort Cloud concept, though obviously not that name, discussed in the work of the Estonian astronomer Ernst Öpik in 1932, which is why you sometimes see the cometary cloud referred to as the Öpik–Oort Cloud. Compared to it, the Kuiper Belt is practically in our backyard at 30 to 50 AU. We’ve pushed probes into the Kuiper Belt, but it’s a matter of pure speculation when we’ll have the technologies to reach the Oort, though of course any future interstellar probes will need to pass through this region. Not to worry, though; comets out here are presumed to be tens of millions of kilometers apart.
At Leiden University in the Netherlands, Simon Portegies Zwart has run simulations mapping the growth of the Oort Cloud that are to be presented in a forthcoming paper in Astronomy & Astrophysics. The work confirms the idea that the Oort is a remnant of the protoplanetary disk from which the Solar System grew some 4.6 billion years ago. While much of it comes from comets ejected from the young Solar System by the gas- and ice-giant planets, the research team suggests that a second population of comets comes from other stars.
Bear in mind that at the Sun’s birth, numerous other stars would have been nearby, from which objects in their circumstellar disks could have been exchanged, along with free-floating debris in the parent star cluster and other interstellar objects. Indeed, a high percentage of the Oort Cloud’s material could have come from such sources, as the paper notes: “About half the inner Oort cloud, between 100 and 104 au, and a quarter of the material in the outer Oort cloud ? 104 au could be non-native to the Solar system but was captured from free-floating debris in the cluster or from the circumstellar disk of other stars in the birth cluster.”
Let’s look further into the paper on how mass moves around as the Cloud is formed:
According to Cai et al. (2019) 20–80% (with an average of 50%) of the circumstellar material survives the first 100 Myr of its evolution in the parent cluster. The majority of this mass is lost through encounters with other stars. The amount of material lost from the solar system in the simulation presented here falls in this range, meaning that the circumstellar disk has lost about half its mass due to interactions with other stars in the parent cluster, or about 100 M? to 3000 M?. Each of the other processes results in a mass loss of roughly 20%. A small fraction of the ejected asteroids acquire bound orbits in the Oort cloud.
The transport of material from one star to another is seen in the simulations to be “rather symmetric.” While the Solar System is what the authors call “a copious polluter of interstellar space,” so too is it receiving material from other systems. The authors argue that stars in the Sun’s birth cluster would have experienced numerous encounters with other stars, and that the Solar System shows evidence of both single strong encounters and a series of relatively weak encounters, based on the orbital parameters of Sedna and the complexity of the orbits found in the scattered Kuiper Belt beyond 45 AU.
These simulations demonstrate that the Oort Cloud evolved using materials from numerous sources. Here is how lead author Portegies Zwart puts the matter:
“With our new calculations, we show that the Oort cloud arose from a kind of cosmic conspiracy, in which nearby stars, planets and the Milky Way all play their part. Each of the individual processes alone would not be able to explain the Oort cloud. You really need the interplay and the right choreography of all the processes together. And that, by the way, can be explained quite naturally from the Sun’s birth environment. So although the Oort cloud is complicatedly formed, it is probably not unique.”
The paper is Zwart et al., “Oort cloud Ecology II: The chronology of the formation of the Oort cloud,” accepted at Astronomy & Astrophysics (preprint).
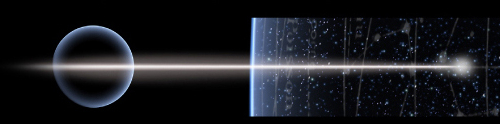
by Paul Gilster | May 26, 2021 | Deep Sky Astronomy & Telescopes |
The galaxy UGC 10738 resonates with the galaxy described yesterday — BRI 1335-0417 — in that it raises questions about how spiral galaxies form. In fact, the team working on UGC 10738 thinks it goes a long way toward answering them. That’s because what we see here is a cross-sectional view of a galaxy much like the Milky Way, one that has both ‘thick’ and ‘thin’ disks like ours. The implication is that these structures are not the result of collisions with smaller galaxies but typical formation patterns for all spirals.
Nicholas Scott and Jesse van de Sande (ASTRO 3D/University of Sydney) led the study, which used data from the European Southern Observatory’s Very Large Telescope in Chile. As you can see from the image below, the galaxy, some 320 million light years away, presents itself to us edge on, offering a cross-section of its structure. Key to the work was the team’s assessment of stellar metallicity, as van de Sande explains:
“Using an instrument called the multi-unit spectroscopic explorer, or MUSE, we were able to assess the metal ratios of the stars in its thick and thin discs. They were pretty much the same as those in the Milky Way – ancient stars in the thick disc, younger stars in the thin one. We’re looking at some other galaxies to make sure, but that’s pretty strong evidence that the two galaxies evolved in the same way.”
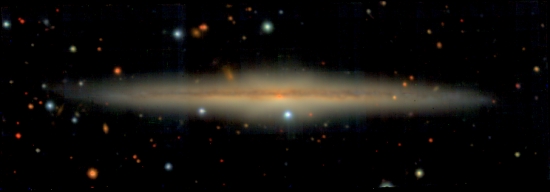
Image: Galaxy UGC 10738, seen edge-on through the European Southern Observatory’s Very Large Telescope in Chile, revealing distinct thick and thin discs. Credit: Jesse van de Sande/European Southern Observatory.
Published in Astrophysical Journal Letters, the study argues that the thin and thick disks found in the Milky Way — and the similar structures found in UGC 10738 — indicate that rather than being the result of chance collisions with other galaxies, the different disks reveal a standard path of galaxy formation and evolution. They would be unlikely to be repeated elsewhere if the result of a rare and violent merger.
The ‘thick’ and ‘thin’ disks are interesting features, with the former primarily made up of ancient stars that show a low ratio of iron to hydrogen and helium. Tracing the metallicity of the thin disk stars reveals that they are younger and contain more metals. Our Sun is a thin disk star, and as the authors note, it is made up of about 1.5% elements heavier than helium (the definition of ‘metals’ in astronomical parlance). Stars of the thick disk show three to ten times less metal content, a striking difference.
Finding the same differences in the two disk structures in other spiral galaxies points to the likelihood that the Milky Way formed in a way common to such galaxies. Says Scott:
“It was thought that the Milky Way’s thin and thick discs formed after a rare violent merger, and so probably wouldn’t be found in other spiral galaxies. Our research shows that’s probably wrong, and it evolved ‘naturally’ without catastrophic interventions. This means Milky Way-type galaxies are probably very common. It also means we can use existing very detailed observations of the Milky Way as tools to better analyse much more distant galaxies which, for obvious reasons, we can’t see as well.”
So we have what the authors describe as a ‘tension’ between two formation scenarios, one stochastic, one common to spiral galaxies. But let’s get into the weeds a little. The paper goes on to widen the sample into other subtypes of galaxy:
This tension is further enhanced once early-type disk galaxies with [α/Fe]-enhanced thick disks are included in the population of present day galaxies with similar formation histories (Pinna et al. 2019b; Poci et al. 2019, 2021). That early-type disk galaxies are found to contain similar abundance patterns as found in the Milky Way (i.e. increased mean [α/Fe] off the plane of the disk) is unsurprising. Such galaxies represent one plausible end point for the Milky Way’s evolutionary history, suggesting a shared and generic evolutionary pathway for disk galaxies.
All of this harks back to Edwin Hubble’s classification of galaxies (the Hubble Sequence). The phrase “early-type” galaxies” refers to elliptical and lenticular galaxies, as opposed to spiral and irregular galaxies, without implying an evolutionary path from one type to another. So the authors are speculating when they talk about an ‘evolutionary end point’ for the Milky Way, but it’s an interesting speculation:
The Milky Way is often identified as a so-called ‘green valley’ galaxy (Mutch et al. 2011), a galaxy already undergoing a transition to the red sequence. When fully quenched (and assuming no dramatic structural changes in the mean time) the Milky Way will likely resemble a lenticular galaxy similar to FCC 170 (Pinna et al. 2019a).
The ‘green valley’ refers to galaxies where star formation has been slowed, either because they have exhausted their reservoirs of gas, or (in younger galaxies) have undergone mergers with other galaxies. Both the Milky Way and Andromeda are assumed to be green valley galaxies of the first kind.
FCC 170 (NGC 1381) is a lenticular galaxy found in the constellation Fornax, about 60 million light years from Earth, a member of the Fornax Cluster (hence the FCC designation, standing for Fornax Cluster Catalogue). As a lenticular, it is in a middle stage between a spiral and an elliptical galaxy, with a large scale disk but lacking spiral arms on the scale of a true spiral galaxy. Is this the Milky Way’s future?
And then, of course, there is the merger with Andromeda to look forward to. How much we have to learn about how galaxies change over time!
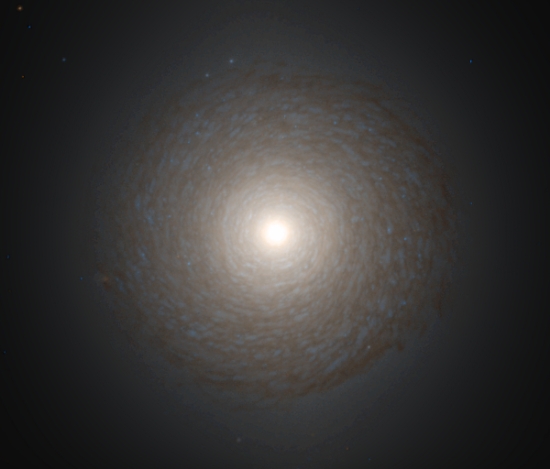
Image: This is NGC 1387, a lenticular galaxy likewise in the Fornax Cluster, and better angled to show the lack of spiral structure in such galaxies than FCC 170. Credit: Fabian RRRR. Based on observations made with the NASA/ESA Hubble Space Telescope, and obtained from the Hubble Legacy Archive, which is a collaboration between the Space Telescope Science Institute (STScI/NASA), the Space Telescope European Coordinating Facility (ST-ECF/ESA) and the Canadian Astronomy Data Centre (CADC/NRC/CSA). CC BY-SA 3.0.
The paper is Scott et al., “Identification of an [α/Fe]—Enhanced Thick Disk Component in an Edge-on Milky Way Analog,” Astrophysical Journal Letters Vol. 913, No. 1 (24 May 2021), L11 (abstract / preprint).
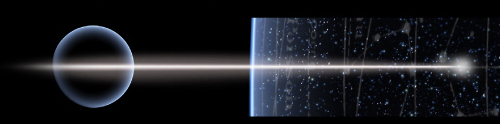
by Paul Gilster | May 25, 2021 | Deep Sky Astronomy & Telescopes |
My fascination with the earliest era of star and galaxy formation leads me to a new paper on an intriguing find. The authors describe the distant object BRI 1335-0417 as “an intensely star-forming galaxy,” and its image as captured by the Atacama Large Millimeter/submillimeter Array (ALMA) is striking. This is a galaxy that formed a mere 1.4 billion years after the Big Bang, making it the most ancient galaxy with spiral structure ever observed.
Spirals make up perhaps 70 percent of the galaxies in our catalogs, but how they form is an open question. Indeed, the proportion of spiral galaxies declines the further back in the evolution of the universe we observe. The spiral structure observed here extends 15,000 light years from the center of the galaxy (about a third the size of the Milky Way), while the total mass of stars and interstellar matter roughly equals our own galaxy.
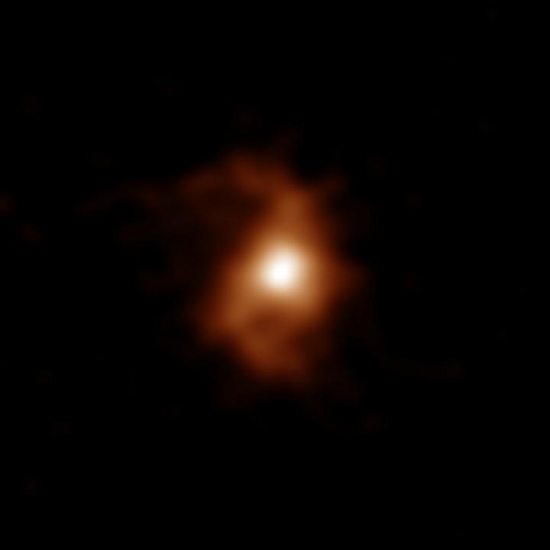
Image: ALMA image of a galaxy BRI1335-0417 in the Universe 12.4 billion years ago. ALMA detected emissions from carbon ions in the galaxy. There is a compact and bright area in the center of the galaxy, and spiral arms are visible on both sides of it. Credit: ALMA (ESO/NAOJ/NRAO), T. Tsukui & S. Iguchi.
The BRI1335-0417 observations were performed by Satoru Iguchi (SOKENDAI/National Astronomical Observatory of Japan), working with graduate student Takafumi Tsukui, who is lead author of the paper reporting the work. Huge amounts of dust exist here, obscuring the object in visible light, but ALMA’s prowess at detecting radio emissions from carbon ions makes the investigation possible.
Even so, Tsukui points out that getting the size of the galaxy right is a difficult matter:
“As BRI 1335-0417 is a very distant object, we might not be able to see the true edge of the galaxy in this observation. For a galaxy that existed in the early Universe, BRI 1335-0417 was a giant.”
The biggest issue is how such a large structure with obvious spiral structure emerged so soon after the Big Bang. This is a ‘starburst’ galaxy: There are areas of active star formation here and gas instabilities in the outer parts of the disk which point to possible interactions with smaller galaxies or other interstellar matter. The paper points out that dusty galaxies with high rates of star formation, like BRI 1335-0417, are the progenitors of elliptical galaxies, with the spiral structure possibly redistributing angular momentum so as to trigger gas inflows into the center of the galaxy.
The authors are quick to note, however, that gas inflows like this are beyond the detection threshold of these observations. What we do see is the possibility of tidal interactions. The authors describe the likelihood of such events:
The high star formation rates of z > 4 [the measure of redshift] galaxies like BRI 1335-0417 are commonly explained as the result of major mergers, which could produce distorted galactic kinematics. We find that BRI 1335-0417 has only slightly disturbed, rotation dominated kinematics, which can be well described by a rotating disk model. This suggests that the high star formation rate must have been maintained long enough for the disk to form after any major merger event. The Q parameter [a measure of stability in a rotating, gaseous accretion disc] shows the outer disk of BRI 1335-0417 is unstable, which could be caused by gas accretion along large-scale filaments of the cosmic web, and/or minor mergers with accreting satellites.
So we have spiral structure, a rotating galactic disk, and a centralized mass. Is BRI 1335-0417 the ancestor of a giant elliptical galaxy? Answering that question of galactic survival and change involves issues of galaxy evolution that remain wide open.
The paper is Tsukui & Iguchi “Spiral morphology in an intensely star-forming disk galaxy more than 12 billion years ago,” published online in Science 20 May 2021 (abstract).
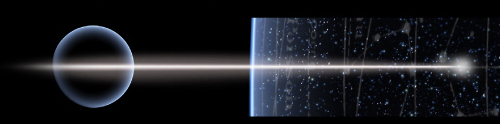