by Paul Gilster | Feb 28, 2013 | Missions |
Existential risks, as discussed here yesterday, seem to be all around us, from the dangers of large impactors to technologies running out of control and super-volcanoes that can cripple our civilization. We humans tend to defer thinking on large-scale risks while tightly focusing on personal risk. Even the recent events near Chelyabinsk, while highlighting the potential danger of falling objects, also produced a lot of fatalistic commentary, on the lines of ‘if it’s going to happen, there’s nothing we can do about it.’ Some media outlets did better than others with this.
Risk to individuals is understandably more vivid. When Apollo 8 left Earth orbit for the Moon in 1968, the sense of danger was palpable. After all, these astronauts were leaving an orbital regime that we were beginning to understand and were, by the hour, widening the distance between themselves and our planet. But even Apollo 8 operated within a sequenced framework of events. Through Mercury to Gemini and Apollo, we were building technologies one step at a time that all led to a common goal. No one denied the dangers faced by every crew that eventually went to the Moon, but technologies were being tested and refined as the missions continued.
Inspiration Mars is proposing something that on balance feels different. As described in yesterday’s news conference (see Millionaire plans to send couple to Mars in 2018. Is that realistic? for more), the mission would be a flyby, using a free return trajectory rather than braking into Martian orbit. The trip would last 501 days and would be undertaken by a man and a woman, probably a middle-aged married couple. Jonathan Clark, formerly of NASA and now chief medical officer for Inspiration Mars, addresses the question of risk head-on: “The real issue here is understanding the risk in an informed capacity – the crew would understand that, the team supporting them would understand that.” Multi-millionaire Dennis Tito, a one-time space tourist who heads up Inspiration Mars, says the mission will launch in 2018.
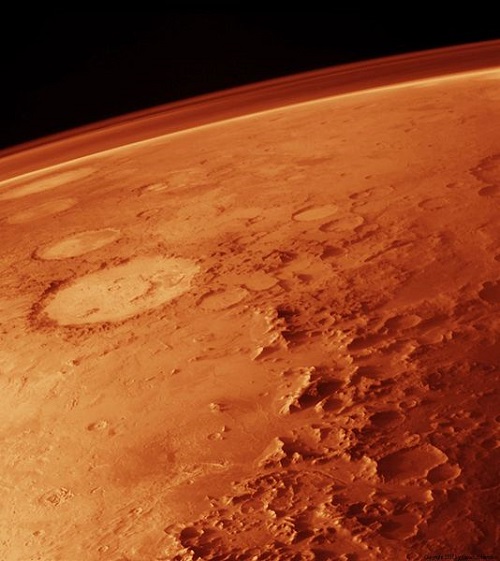
Image: A manned Mars flyby may just be doable. But is the 2018 date pushing us too hard? Image credit: NASA/JPL.
We’ll hear still more about all this when the results of a mission-feasibility study are presented next weekend at the 2013 IEEE Aerospace Conference in Montana. Given the questions raised by pushing a schedule this tightly, there will be much to consider. Do we have time to create a reliable spacecraft that can offer not only 600 cubic feet of living space but another 600 for cargo, presumably a SpaceX Dragon capsule mated to a Bigelow inflatable module? Are we ready to expose a crew to interplanetary radiation hazards without further experience with the needed shielding strategies? And what of the heat shield and its ability to protect the crew during high-speed re-entry at velocities in the range of 50,000 kilometers per hour?
For that matter, what about Falcon Heavy, the launch vehicle discussed in the feasibility analysis Inspiration Mars has produced for the conference? This is a rocket that has yet to fly.
No, this doesn’t feel much like Apollo 8. It really feels closer to the early days of aviation, when attention converged on crossing the Atlantic non-stop and pilots like Rene Fonck, Richard Byrd, Charles Nungesser and Charles Lindbergh queued up for the attempt. As with Inspiration Mars, these were privately funded attempts, in this case designed to win the Orteig Prize ($25,000), though for the pilots involved it was the accomplishment more than the paycheck that mattered. Given the problems of engine reliability at the time, it took a breakthrough technology — the Wright J-5C Whirlwind engine — to get Lindbergh and subsequent flights across.
Inspiration Mars is looking to sell media rights and sponsorships as part of the fund-raising package for the upcoming mission, which is already being heavily backed by Tito. I’m wondering if there is a breakthrough technology equivalent to the J-5C to help this mission along, because everything I read about it makes it appear suicidal. The 2018 date is forced by a favorable alignment between Mars and the Earth that will not recur until 2031, so the haste is understandable. The idea is just the kind of daring, improbable stunt that fires the imagination and forces sudden changes in perspective, and of course I wish it well. But count me a serious skeptic on the question of whether this mission will be ready to fly on the appointed date.
And if it’s not? I like the realism in the concluding remarks of the feasibility study:
A manned Mars free-return mission is a useful precursor mission to other planned Mars missions. It will develop and demonstrate many critical technologies and capabilities needed for manned Mars orbit and landing missions. The technology and other capabilities needed for this mission are needed for any future manned Mars missions. Investments in pursuing this development now would not be wasted even if this mission were to miss its launch date.
Exactly so, and there would be much development in the interim. The study goes on:
Although the next opportunity after this mission wouldn’t be for about another 13 years, any subsequent manned Mars mission would benefit from the ECLSS [Environmental Control and Life Support System], TPS [Thermal Protection System], and other preparation done for this mission. In fact, often by developing technology early lessons are learned that can reduce overall program costs. Working on this mission will also be a means to train the skilled workforce needed for the future manned Mars missions.
These are all good reasons for proceeding, leaving the 2018 date as a high-risk, long-shot option. While Inspiration Mars talks to potential partners in the aerospace industry and moves ahead with an eye on adapting near-Earth technologies for the mission, a whiff of the old space race is in the air. “If we don’t fly in 2018, the next low-hanging fruit is in ’31. We’d better have our crew trained to recognize other flags,” Tito is saying. “They’re going to be out there.”
In 1968, faced with a deadline within the decade, NASA had to make a decision on risk that was monumental — Dennis Tito reminded us at the news conference that Apollo 8 came only a year after the first test launch of the Saturn 5. Can 2018 become as tangible a deadline as 1970 was for a nation obsessed with a Moon landing before that year? If so, the technologies just might be ready, and someone is going to have to make a white-knuckle decision about the lives of two astronauts. If Inspiration Mars can get us to that point, that decision won’t come easy, but whoever makes it may want to keep the words of Seneca in mind: “It is not because things are difficult that we dare not venture. It is because we dare not venture that they are difficult.”
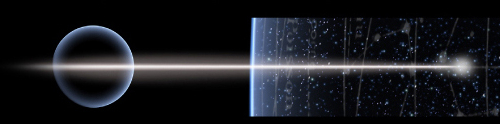
by Paul Gilster | Feb 27, 2013 | Uncategorized |
At the top of my list of people I’d someday like to have a long conversation with is Nick Bostrom, a philosopher and director of Oxford’s Future of Humanity Institute. As Centauri Dreams readers will likely know, Bostrom has been thinking about the issue of human extinction for a long time, his ideas playing interestingly against questions not only about our own past but about our future possibilities if we can leave the Solar System. And as Ross Andersen demonstrates in Omens, a superb feature on Bostrom’s ideas in Aeon Magazine, this is one philosopher whose notions may make even the most optimistic futurist think twice.
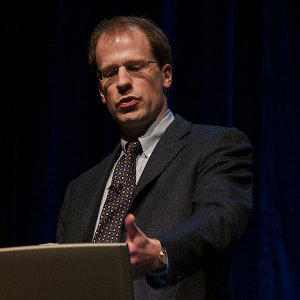
I suppose there is such a thing as a ‘philosophical mind.’ How else to explain someone who, at the age of 16, runs across an anthology of 19th Century German philosophy and finds himself utterly at home in the world of Schopenhauer and Nietzsche? Not one but three undergraduate degrees at the University of Gothenburg in Sweden followed. Now Bostrom applies his philosophical background, along with training in mathematics, to questions that are literally larger than life. As Andersen reminds us, ninety-nine percent of all species that have lived on our planet are now extinct, including more than five tool-using hominids. Extinctions paved the way for the emergence of new species, but for the species du jour, survival is the imperative.
Image: Philosopher Nick Bostrom at a 2006 summit at Stanford University. Credit: Wikimedia Commons.
Colonizing Waves or Individual Explorers?
You’ll want to read Andersen’s essay in its entirety (helpfully, there’s a Kindle download link) to see how Bostrom sizes up existential risks like asteroid impacts and supervolcanoes. One of the latter, the Toba super-eruption about 70,000 years ago, seems to have pumped enough ash into the atmosphere to destroy the food chain of our distant ancestors, leaving a scant few thousand alive to move into and populate the rest of the planet. We do seem to be a resilient species. Bostrom likes the long view, which means he sees a 100,000 year hiatus as humans bounce back from a possible future catastrophe as little more than a pause in cosmic time. That perspective has interesting consequences, as Andersen notes:
It might not take that long. The history of our species demonstrates that small groups of humans can multiply rapidly, spreading over enormous volumes of territory in quick, colonising spasms. There is research suggesting that both the Polynesian archipelago and the New World — each a forbidding frontier in its own way — were settled by less than 100 human beings.
This is a point worth remembering as we contemplate the possibility of interstellar flight. We sometimes think of enormous colonies of humans moving to nearby stars, but early human settlements may be the result of tiny groups who, for reasons we can only guess at, decide to cross these fantastic distances. Maybe rather than a planned program of expansion, our species will see sudden departures of groups heading out for adventure or ideology, small bands who leave the problems of Earth behind and create entirely new societies outside of any central planning or control. Meanwhile, the great bulk of humans choose to stay at home.
Andersen’s essay is so rich that I will, for today at least, pass over his discussions with Bostrom about artificial intelligence and the dangers it represents — you should read this in its entirety. Let’s focus in on the Fermi paradox and why Bostrom hopes that the Curiosity rover finds no signs of life on Mars. For the consensus at Bostrom’s Future of Humanity Institute, shared by several of the thinkers there, is that the Milky Way could be colonized in a million years or less, leading to the question of why we don’t see this happening.
Filters Past and Future
Are we looking at an omen of the human future? Robin Hanson, another familiar name to Centauri Dreams readers, works with Bostrom at the Institute. He tells Andersen that there appears to be some kind of filter that keeps civilizations from developing to the point where they build starships and fill the galaxy. The filter would exist somewhere between inert matter and cosmic transcendence, and thus could be somewhere in our past or in our future.
In other words, what if we have somehow survived a filter that keeps life from developing on most planets? Or perhaps it’s a filter that acts to screen out intelligent life-forms, and we have somehow made our way through it. If the ‘great filter’ is in our past, then we can hope to expand into a cosmos that may be largely devoid of intelligent life. If it is in our future, then we can’t predict what it will be, but the ominous silence of the stars bodes ill for our survival.
But let Andersen tell it, in one of his conversations with Bostrom:
That’s why Bostrom hopes the Curiosity rover fails. ‘Any discovery of life that didn’t originate on Earth makes it less likely the great filter is in our past, and more likely it’s in our future,’ he told me. If life is a cosmic fluke, then we’ve already beaten the odds, and our future is undetermined — the galaxy is there for the taking. If we discover that life arises everywhere, we lose a prime suspect in our hunt for the great filter. The more advanced life we find, the worse the implications. If Curiosity spots a vertebrate fossil embedded in Martian rock, it would mean that a Cambrian explosion occurred twice in the same solar system. It would give us reason to suspect that nature is very good at knitting atoms into complex animal life, but very bad at nurturing star-hopping civilisations. It would make it less likely that humans have already slipped through the trap whose jaws keep our skies lifeless. It would be an omen.
This essay will take up half an hour of your day, but I suspect that, like me, you’ll go back and read it again, reflecting on its themes for days to come. Are there questions of philosophy that are more urgent than others? Ponder a moral issue that is much in play at the Future of Humanity Institute, the idea that existential threats to our species may outweigh our obligations to serve those who are suffering today. “The casualties of human extinction,” Andersen writes, ” would include not only the corpses of the final generation, but also all of our potential descendants, a number that could reach into the trillions.”
In my view, that’s an argument for, among other things, a robust space program going forward, one that is capable of securing our planet from impact threats and establishing off-world colonies that would survive any other forms of planetary catastrophe, from runaway artificial intelligence to the weaponization of microbes. It’s also an argument for taking the kind of long-term perspective so lacking in modern culture, the case for which has never been made more clearly than in this elegant and illuminating essay.
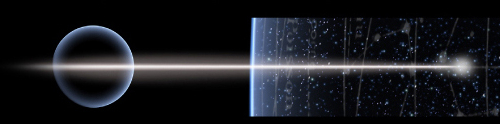
by Paul Gilster | Feb 22, 2013 | Uncategorized |
Michael Michaud wrote the essay that follows back in 1978 for a now-defunct magazine that never published it. In recent correspondence about Daedalus designer Alan Bond, Michael referred to the essay and I asked him to forward a copy, which had also passed through the hands of Freeman Dyson and Bob Forward not long after he wrote it. Although it is dated, Starships of the Mind does a wonderful job presenting the major interstellar propulsion ideas, leavened with Michael’s innate optimism, which has inspired me for many years. It’s a bit of starship history that deserves to be in circulation, and Michael was kind enough to agree. Many thanks are owed as well to my friend David Warlick, who scanned the original and, through the wonders of optical character recognition, rendered it into digital form.
Centauri Dreams readers will know Michael Michaud as the author of Contact with Alien Civilizations: Our Hopes and Fears about Encountering Extraterrestrials (Springer, 2007), an essential book for anyone interested in SETI. Among his numerous other works are many on space exploration. Michael was a U.S. Foreign Service Officer for 32 years, serving as Counselor for Science, Technology and Environment at the U.S. embassies in Paris and Tokyo, and Director of the State Department’s Office of Advanced Technology. He has also been chairman of working groups at the International Academy of Astronautics on SETI issues.
By Michael A.G. Michaud
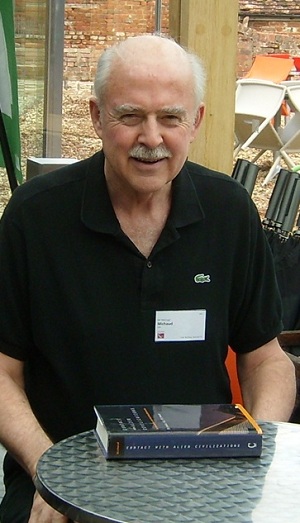
A giant is loose in the darkness of interstellar space. Its voracious maw, seventy five miles wide, sucks in particles of gas and dust, cutting a tunnel through the interstellar medium. In the monster’s interior, the miniature sun of a fusion engine converts atoms and molecules into propulsive energy, driving the giant forward, leaving a wake of superheated gas. Accelerating toward the speed of light, it races through the galaxy.
Have we tuned in on Star Trek? Not at all. This is merely a description of an interstellar ramjet, proposed by nuclear physicist Robert Bussard as one way of achieving interstellar flight.
Starflight is one of the great dreams of the 20th century. As long ago as 1918, the American rocket pioneer Robert Goddard wrote “The Ultimate Migration”, describing a journey to the stars by humans fleeing a dying Sun. During the 1930’s, science fiction writers like Olaf Stapledon began using interstellar scenarios in their stories. But, while Leslie Shepherd published a pioneering article on interstellar flight in 1952, few technical experts paid much attention.
Many scientists still argue that humans will never travel to other suns. If it is possible at all, they say, it would be so difficult, expensive, and time-consuming as to be not worth while. But others, with solid backgrounds and reputations in technical fields, are seeking ways to traverse the interstellar void. Starships are forming in their minds.
Distant Candles
The stars are the end of dream. They are the ultimate symbol of remoteness, of unknowability, of superlative achievement per ardua ad astra — through struggle to the stars. But their existence tantalizes us, suggesting infinite variety, new worlds, other lives. They are distant candles in the outer dark, luring Humankind outward from its modest origins.
The French philosopher Auguste Comte, writing in the 19th century, described the chemical nature of the stars as the ultimate example of something humans could never know. Yet in his own time astronomers were beginning to use a new tool — the spectroscope — to determine the elements in distant suns. We began to see stars as they truly are, not glowing supernatural entities beyond human ken, but measurable expressions of physical law. Measurement was the first step toward bringing them within the realm of human aspiration.
The stars are huge, yet so distant that even large telescopes show them only as points of light. In 1974, astronomers at Kitt Peak National Observatory in Arizona used a new computer-assisted technique called speckle interferometry to build up an image of Betelgeuse in the constellation Orion, a red giant star 800 times the size of our Sol. For the first time, we saw the boiling, irregular, blotched surface of another sun. The stars seemed a little closer.
But interstellar distances still stagger the human imagination. We think of our solar system as being large; the ice-dwarf Pluto, recedes to as much as 4 billion miles from the Earth in its eccentric orbit around the Sun. Only in 1978 — 48 years after its discovery — did astronomers find that Pluto has a moon. But the star system nearest to the Sun, the triple we call Alpha Centauri, is about 25 trillion miles away — 6,000 times as far as Pluto. To make the numbers manageable, astronomers measure distances to the stars in light years — the distance light travels in one year at 186,282 miles a second.
The Solar Neighborhood
Our Sun is located in the disk of the spiral galaxy we call the Milky Way, about 33,000 light years out from the center. It is on the inner edge of one of the galaxy’s spiral arms, though our view of the galactic nucleus is obscured by clouds of interstellar dust. The galaxy is enormous: about 100,000 light years across and 12,000 light years thick at the hub, containing as many as 250 billion stars.
Better to start with our own obscure neighborhood, out here in the galactic boondocks. Within 20 light years of Sol are about 90 known stars — 51 single, 15 double, and 3 triple. A few, like the bright star Sirius, are massive, hot, and short-lived. But most are cooler, reddish stars, smaller and dimmer than our Sun. A few are very similar to Sol, and may be the most likely to have planets with Earth-like conditions.
The space between the stars is not completely empty. The Sun is surrounded by planets, moons, asteroids, comets, and a disk of rarefied gas and dust. Beyond the outermost planet may lie a cloud of ice balls, marking the outer edge of the solar system; this “Oort cloud” may be the source of the comets. From that distance, billions of miles beyond Pluto, the Sun would be only a bright star. Circling in the dark, these chunks of frozen gas would be the outposts of the Sun’s empire, at the edge of the interstellar void. Other stars also might have disks of material reaching far out from them, offshore reefs around the galaxy’s islands of light.
We know that interstellar space is thinly sprinkled with atoms of gas and dust, which sometimes collect to form the clouds we call nebulas. But there also may be dark objects we have not yet found. We cannot exclude even small, dark stars, too faint for our present instruments to see.
Crossing the Gulf
We already have launched our first spacecraft to the stars: the unmanned probe Pioneer-10, which flew by Jupiter in 1973 and is now on its way out of the solar system. This spindly robot is a speedy craft by our present standards; it took only 11 hours to travel from the Earth to the distance of the Moon, and was doing over 90,000 miles an hour when it whipped by Jupiter. But its speed is so modest on interstellar scales that it would take over 100,000 years to reach the nearest star — if it were headed in that direction. Its electronic systems would die long before then, and its weak radio could not signal the Earth to tell of its discoveries. Our machines do not yet match the scale of interstellar flight. [Editor’s note: The Voyager probes had just been launched when this article was written. Pioneer 10 is now moving at 12.51 kilometers per second. The faster Voyager 1, moving at 17.1 kilometers per second, would make the Alpha Centauri crossing in about 75,000 years].
Thinking about interstellar travel by human beings brings us face to face with a more fundamental limitation: the human lifespan. No adult could count on surviving a trip of`more than about 50 years. Even at the speed of light, this would limit us to a tiny sampling of the Galaxy, and confine us to our neighborhood. Human longevity might even prevent a commitment to an unmanned probe mission lasting longer than 50 years; those who launched it would not live to hear the results. The fundamental problem of interstellar flight, then, is not distance, but time, and our attitude toward it.
The obvious, superficial solution to the time problem is high velocity, shortening the duration of the journey. But there are limits. Scientists believe that nothing can exceed the speed of light — a barrier that looks far more absolute than the speed of sound did before we broke it. And the problem is more subtle. As an object accelerates to a significant fraction of light velocity, its mass increases, as does the amount of energy needed to propel it. A large starship traveling to a nearby star at 99 per cent of the speed of light would have to convert completely into energy a solid body the size of the Moon. To reach the speed of light, it would need an infinite amount of energy to move its infinite mass.
But can we even get close to light speed? The Apollo moon landing missions used enormous chemical rockets, standing over 300 feet tall, to lift small vehicles out of the Earth’s gravity field; the weight of the payload, when compared to the weight of the propulsion system, was tiny. Chemical rockets are inadequate for interstellar flight. Even nuclear fission reactors, used to heat fuel or to accelerate charged particles as exhaust, are not good enough.
The propulsion problem causes many scientists to conclude that starflight simply is not feasible. Interstellar travel, said astrophysicist Edward Purcell, belongs right back where it came from — on the cereal box.
Engines for Starflight
Serious theoretical work on interstellar propulsion began soon after the dawn of the Space Age. Scientists and engineers, recognizing the limitations of chemical and nuclear fission energy sources, began to spin off new ideas.
In the early 1960’s, scientists like Dwain Spencer and Leonard Jaffe calculated the potential speed of rockets with nuclear fusion engines, which could heat fuel to high exhaust velocities and accelerate ships to high speeds, perhaps one-third that of light. These ships would have to carry along very large masses of fuel, such as deuterium and helium-3. Energy research might bring closer the age of the fusion starship; its engines might be easier to develop than fusion generating plants on Earth because the problem of containing the superheated plasma might be less. Fusion reactors could be used to create ion rockets, accelerating charged particles as exhaust.
In the 1960’s, physicists Theodore Taylor, Freeman Dyson and others worked on Project Orion — a nuclear pulse engine. In this concept, one atomic bomb would be ejected every second from the tail of the spacecraft to explode behind it, pushing the ship forward. Dyson showed that, with present technology, we could accelerate a ship to a thirtieth of the speed of light within 10 days at an acceleration of one gravity by exploding hydrogen bombs one kilometer behind the ship, catching 10 per cent of the energy with a screen one kilometer across. A shock absorber would transmit the energy to the ship.
The ultimate rocket using an internal energy source would be one in which the matter of the fuel was converted completely into energy. The only known way to do this is to combine matter with anti-matter in a controlled way. Since anti-matter would destroy anything else it contacted, it would have to be held in a magnetic field and released in small amounts. So far, only minute quantities of anti-matter have been created in accelerators, and only for fractions of a second. Yet this “fuel” is so powerful that some scientists are looking for ways to overcome the problems of an anti-matter drive.
Starships would be more efficient if they did not have to carry both a fuel supply and an energy source. NASA is taking a step toward an externally powered system by developing a solar electric engine, in which solar panels provide energy to accelerate charged particles as exhaust. This could be used first for a mission to a comet in the l980’s. But solar panels would not work in the dark of interstellar space. Here Bussard’s ramjet becomes an elegant concept because it uses the galaxy’s raw material as reaction mass for a fusion engine, sucking in rarefied gas the way a jet sucks in air. But the ramjet scoop would have to be enormous to gather up enough fuel; no one knows how to create and maintain a cone-shaped magnetic field reaching a hundred miles or more out in front of the starship.
The invention of the laser led to suggestions that we beam energy to the starship from our solar system, heating the fuel of its propulsion system. This might require huge batteries of lasers, perhaps energized by giant solar power stations orbiting the Earth or the Sun. Or lasers could be aimed at giant “light sails” of thin material, stretched out in front of the starship. In the novel The Mote in God’s Eye, by Larry Niven and Jerry Pournelle, the bright light that appears in front of a nearby star turns out to be a green laser driving an interstellar craft across the void. Philip Norem has suggested how a laser could be used to decelerate a “starjammer” as it approached its target system.
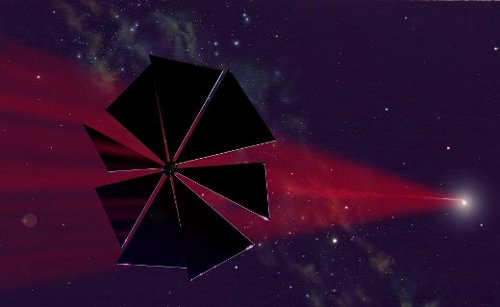
Image: An interstellar sail pushed by laser or microwave. Credit: Michael Carroll.
Freeman Dyson proposed that interstellar vehicles use “gravity machines”, swinging by massive stars that would accelerate them and fling them onward in new directions. T.A. Heppenheimer, author of Colonies in Space, has pointed out that an interstellar probe aimed at one nearby star could, in some cases, be slung onward to another in a game of celestial billiards. Engineer Peter Bielkowicz has described a hypothetical journey to a nearby neutron star; too close an approach would tear the ship apart.
Starflight, then, may be possible if we do not aim for speeds near that of light. But one can dream, writes aerospace engineer Maxwell Hunter, that the most intriguing question among the engineers and scientists of a future time will be an assault on the light barrier.
Probing the Stars
In 1973, a small group of British scientists and engineers led by Alan Bond, all members of the British Interplanetary Society, set out to design an interstellar probe based on foreseeable technology. The requirements were that the probe traverse the 6 light year distance to Barnard’s Star within 50 years, deploy sensors, and radio its findings back to Earth. The study was named Project Daedalus, after the mythical Greek artificer who escaped from Crete on wings made of feathers and wax (his son, Icarus, fell from the sky when he flew too close to the Sun).
The B.I.S. Starship Study, published in 1978, described a colossal two-stage vehicle weighing almost 3,000 tons without fuel. Half of each stage would be an engine, consisting of a device to inject 250 small pellets of deuterium and helium-3 a second into the center of a bell-shaped combustion chamber hundreds of feet across. Electron beams would zap the pellets, causing them to implode and fuse, producing a continuing series of small thermonuclear blasts that would accelerate the starship out of our solar system. The first stage would fire for two years before it dropped off; the second would accelerate for another year and three quarters. After reaching over 12 per cent of the speed of light, the probe would coast the rest of the way, managed by an advanced on-board computer with characteristics of an artificial intelligence, and tended by large maneuverable robots called wardens. The probe would flash through the Barnard’s Star system in a few hours, launching up to 18 sub-probes to study that star and the planets that may circle it. The main probe, by then well beyond Barnard’s Star, would collect the information and radio it back to our solar system, where it would be received six years later.
The study team concluded that the Daedalus probe would require the resources of a society richer than humanity is today, and one with improved spaceflight capabilities — perhaps a solar system civilization. The most feasible source of large quantities of helium-3 appears to be the atmosphere of Jupiter, where compressors hung from giant balloons would collect the gas. The probe itself would have to be assembled and launched from an orbit around Jupiter or one of its moons.
The authors believe that such a probe would not be built or launched until well into the next century. Still, some people have suggested that the Daedalus report is the first step toward putting interstellar flight on a sound engineering basis. It may be comparable to the 1937-1939 British Interplanetary Society Moonship Study that had so many parallels in the Apollo project 30 years later. The B.I.S. Journal now publishes four “Interstellar Studies” issues each year, filled with technical articles and essays on interstellar travel and communication. [Editor’s note: JBIS remains an active venue for interstellar studies under the editorship of Kelvin Long].
Americans too have been active in laying the groundwork for interstellar probes. In 1975, Hughes Research Laboratory physicist Robert Forward proposed a 50 year national program of interstellar exploration, using small probes that might achieve a third of light speed. Forward pointed out that the continuing miniaturization of electronics might allow probe payloads to weigh only a few hundred pounds instead of many tons, drastically reducing the energy requirements for their propulsion. On arrival, a Forward probe would brake into orbit around the most interesting planet; its compact mass would open up into a silvery mesh, a spherical network of interconnected sensors and computer elements over 300 feet across. This latticework probe might use a laser to drive part of its mesh down through the atmosphere to the surface, and relay the sensors’ findings back to our solar system.
Before we launch probes to the stars, we might first take shorter steps out into the interstellar environment. Pioneer 10 and the Voyager probes will send back some information about this unexplored territory before their signals become too faint. In 1970, spaceflight pioneer Krafft Ehricke suggested an “ultraplanetary” probe that would swing around Jupiter or the Sun before flying out into the interstellar cold. Leonard Jaffe of the Jet Propulsion Laboratory in Pasadena recently led a study of an interstellar “precursor” mission that would fly by Pluto and head outward in the direction of the Sun’s motion through the Galaxy, like a scout sent ahead of our civilization. Forward has suggested that we send a probe beyond the solar system and then bring it back in, to see if it is smart enough to detect life.
Why Probes?
The scientific motivation for interstellar probes stems from the limits of astronomy. With telescopes alone, we can never be sure what lies within other solar systems, or if their planets have evolved life and civilization. The most important reason for interstellar probes, says “Interstellar Studies” editor Anthony R. Martin, is simply to see what is out there.
Interstellar probes may become major tools in the search for life and intelligence in the galaxy. In 1966, American astronomer Carl Sagan and Soviet astronomer Iosif Shklovskii argued that, within a few hundred years, humanity could launch probes to all nearby stars of interest. Astronomer A.G.W. Cameron, testifying before the U.S. Congress in 1978 on extraterrestrial intelligence research, said that we might have to use interstellar probes in our search for alien life.
In 1960, radio astronomer Ronald Bracewell suggested that an advanced alien civilization, instead of listening from its home system for radio messages, might send long-lived probes to orbit other stars, to listen for intelligent signals, and to reply if they heard any.
The most important reason for interstellar probes is one even proponents are reluctant to state, for fear of appearing overly ambitious. It is to find new worlds for humanity. RAND Corporation engineer Stephen Dole, in a book with Isaac Asimov titled Planets for Man, estimated that there might be 600 million habitable planets in our galaxy. The nearest star with such a planet may be, on the basis of averaging, only 20 or 30 light years away. If we send probes into the life zones of favorable temperatures around nearby stars, we will be looking as much toward our own future as toward the discovery of aliens.
It is not attractive to send manned starships solely to explore; they would have to be larger and more elaborately equipped than robot craft, increasing their cost. And they would have to make round trips, shortening their range and limiting exploration to the nearest stars. Better to send one-way, expendable unmanned probes to fly by their targets, or to enter looping orbits around other stars, spreading wings of solar cells, and beeping back information to giant antennas in our solar system.
There is only one convincing reason for manned starflight: the colonization of other star systems. We can only guess why our descendants would make this effort. It may be the result of an impending disaster; the pressure of population, conflict or oppression within a solar system civilization; contact with extraterrestrials; or some grander vision of the human role in the universe. Except in an emergency, manned starships would not fly until robot probes told us of a hospitable environment But once we find a new habitat for Humankind in the stars, there may be no turning back.
The Outleap
“Warp Factor Five, Mr. Sulu.”
Science fiction, in showing humans traveling between the stars, has resorted to gimmicks to get around the light barrier. It is full of “space warps” and “hyperspace drives”, “stargates”, and “jumps” through other dimensions of space-time like that made by Han Solo’s Milennium Falcon in Star Wars.
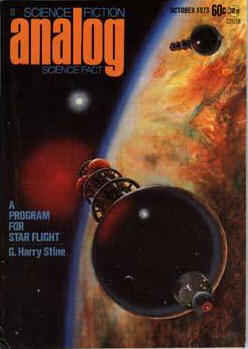
The reality may not be so neat. Early starships carrying human pioneers may be slow boats to Centauri, making perhaps 1/100 the speed of light. Journeys may take centuries. This leads to the concept of the “generation ship”, in which the original crew dies en route, but is succeeded by generation after generation until the space ark finally makes planetfall. Scottish astronomer John MacVey, in Journey to Alpha Centauri, described a pair of giant starships, each carrying 3,000 people to a planet in the nearest star system. Robert Enzmann proposed a convoy of ten ships, aimed at stars where probes had found gas giant planets similar to our Jupiter. Each ship would start with 200 people, but that would grow to 2,000 during the long voyage. G. Harry Stine, expanding on Enzmann’s ideas, suggested that starships failing to find a habitable planet could refuel from a gas giant and head outward to their next destination, on a voyage of uncertain duration.
Image: The Enzmann starship, as pictured on the cover of the October, 1973 issue of Analog, the issue that included Harry Stine’s ideas on interstellar expansion. Cover art by Rick Sternbach.
Interstellar arks might be self-propelled space colonies, like the rotating globular or cylindrical habitats proposed by Princeton physicist Gerard O’Neill, equipped with powerful engines for interstellar flight. The migrants might even hollow out an asteroid, converting much of its interior to reaction mass for a propulsion system.
But could such isolated islands of humanity survive these journeys? No one yet knows how to design a large closed ecology, though the Soviets have kept men in space for six months. Starship societies might become very ingrown; tensions might rise as the initial enthusiasm waned, and middle generations faced the prospect of a journey which, for them, would have neither beginning nor end. The ship would become the totality of their existence. Robert Heinlein, in his 1940 science fiction story “Universe”, suggested that passengers might forget where they were going, and why.
Aging research may bring an extension of the average human lifespan, removing the 50 to 70 year limit on manned interstellar flight. But would crews remain sane during a voyage of centuries? We may need more drastic solutions.
One is suspended animation. The metabolisms of the colonists might be stopped or drastically slowed by new biomedical techniques. These might include cryonics — the freezing of human beings. By making time irrelevant, cryonics could open the road to the stars. Even hibernation would help, by reducing the amount of waking time and the consumption of supplies. But we do not yet have reliable techniques for suspending human metabolism over long periods, or freezing and thawing human bodies without damage.
Science and science fiction writers have suggested that we get around these problems by not sending adult humans at all, but carefully protected ova and sperm. Near the target system, they would be united to become children, to be raised by a motherly computer until they were ready to set foot on a new world, like a second Adam and Eve.
If future humans make breakthroughs in propulsion allowing travel at speeds more than half that of light, they could exploit the effects of relativity. To a hypothetical stationary observer, it would appear that time was passing more slowly inside the starship than on Earth, though the passengers would not notice any difference in their lives. Because of this time dilation, the star travelers would age more slowly than their friends and relatives.
The Challenge of the Starship
Why do humans design starships? Not for material reward; they get no government grants, or fat contracts. It does not help their professional careers; other scientists criticize their proposals as being unrealistic or even outrageous. Our Space Agency funds no work on interstellar flight; NASA advanced planner Jesco von Puttkamer calls it “the wildest science fiction”. While the ebullient Forward hopes to see the first interstellar probe launched before he retires in 1998, few starship designers expect quick results from their work, and none are likely to visit other suns. Yet they persist, a small band of pioneers, from diverse origins, working in their spare time, sharing a common passion. [Editor’s note: Bob Forward, alas, did not see his dream realized. The interstellar theorist died in 2002].
To a physicist or an engineer, the starship is the ultimate challenge. Making it work, at least on paper, is an intense intellectual pleasure. Designers must defeat time and space through the application of human intellect and technology. They work on the largest scales imaginable, speaking of light years, and energies that dwarf our hydrogen bombs. The ships themselves range from the shimmering lattices of Forward’s mini-probes to the colossi of Enzmann, each pushing a 12 million ton iceberg of frozen deuterium.
Interstellar travel forces us to the edge of science, to exotic physical concepts. Some astrophysicists have speculated that black holes, the massive collapsed stars that suck in even their own light, might have exit points elsewhere in the universe, perhaps in white holes that gush out matter and energy. In The Cosmic Connection, Carl Sagan speculated that advanced civilizations might use a black hole transport system, like a subway through the universe. Science writer Adrian Berry, in The Iron Sun, even proposed that humans build their own black holes outside our solar system. Texas physicist John Wheeler suggested that different points in space-time might be connected by “worm-holes”, tiny, short-cut passages.
Recent work has cast doubt on the utility of these concepts for interstellar transportation. But the speculation goes on. Forward wonders if we might someday escape the chains of gravity and inertia, and not be limited to what he calls “the slow crawl of light”.
Ad Astra
Though few starship designers will say so, there is hidden in their work a transcendental idea: that humans should expand outward to explore and colonize the galaxy. Bob Parkinson, a member of the Daedalus team, describes the starship as a philosophical vehicle, the endpoint of a process that began with the physical exploration of the world in the Renaissance.
M.I.T. astrophysicist Philip Morrison criticizes the idea that humanity must expand, naming such a concept “Malthusian” after the grim parson who argued that living things increase until they meet the limits of their food supply. Carl Sagan calls it human chauvinism. But our politically divided species, dangerously armed, may need a grand idea that would define humanity as a whole by contrast with an outside it could explore and settle. Aiming for the stars would force us, constructively, into longer time scales of social endeavor. A star-faring society, writes Parkinson, would have to plan its future not in years and decades, but in centuries and millennia. Such a civilization would have to believe in itself.
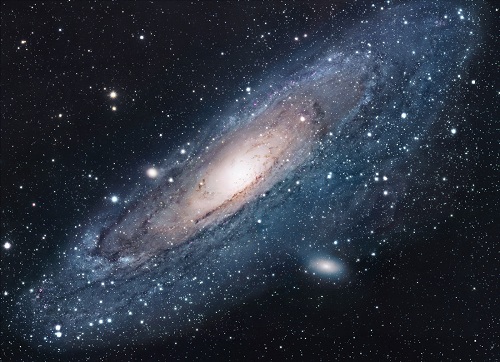
Image: M31, the nearest galaxy to our own. Is it an imperative of intelligence to move outward and explore such vast regions? Credit: Robert Gendler.
There also is an appeal to deep human drives in the extension of human influence and purpose outward into an inanimate universe, the victory of mind over mindlessness. But Dyson, with typically elegant simplicity, says starflight is not a property of our species; it is life’s way of moving around the universe.
The key to expansion will lie in our minds, in our conceptions of what humans can and should be. We may learn to think of our solar system as an inland sea at the edge of the interstellar ocean. We may come to see the stars as lighthouses in the cosmic darkness, welcoming fires in the coldness of the void, furnaces for new civilizations. Eventually, a patient outside observer might see ripples of intelligent activity spreading through the galaxy, from a central disturbance called Earth.
Science fiction publisher Donald Wollheim has noted a widely shared vision of the human future among science fiction writers. This future history begins with journeys to the planets and their colonization, and extends to interstellar travel, star colonies, and the formation of a human galactic empire.
This may be too ambitious for a species of our modest abilities. But if we choose to journey out, however far we go, it will begin with the starships of the mind.
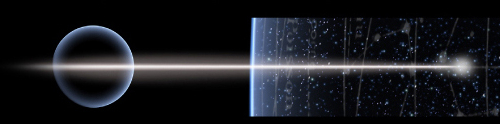