by Paul Gilster | Apr 30, 2015 | Outer Solar System |
One of the more memorable moments from yesterday’s teleconference on the New Horizons mission was Alan Stern’s comment that the latest pixelated images of Pluto/Charon constituted his ‘meet Pluto moment.’ If anyone has an interest in meeting Pluto, it’s Stern (Southwest Research Institute), who serves as principal investigator and whose unflagging efforts made it possible. As for those pixelated views, well, they’re a glimpse of what is to come, but even now, they’re telling us helpful things about the target. The animation below speaks volumes, with the first showing Charon’s rotation with the center of Pluto fixed in the frame. The images were acquired with the Long Range Reconnaissance Imager (LORRI) camera.
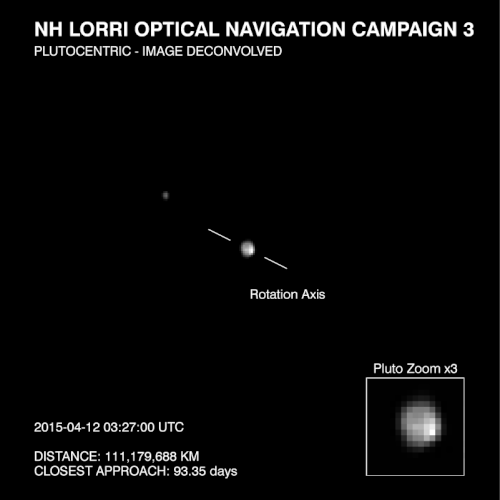
Image: A series of LORRI images of Pluto and Charon taken at 13 different times spanning 6.5 days, from April 12 to April 18, 2015. During that time, the spacecraft’s distance from Pluto decreased from about 111 million kilometers to 104 million kilometers. Pluto and Charon rotate around a center-of-mass (also called the “barycenter”) once every 6.4 Earth days, and these LORRI images capture one complete rotation of the system. The direction of the rotation axis is shown in the figure. In one of these movies, the center of Pluto is kept fixed in the frame, while the other movie is fixed on the center of mass (accounting for the “wobble” in the system as Charon orbits Pluto). Credit: NASA/Johns Hopkins University Applied Physics Laboratory/Southwest Research Institute.
The second image shows the same view with the motion around the barycenter clearly revealed, a spectacular teaching tool for those trying to explain rotation around the center of mass.
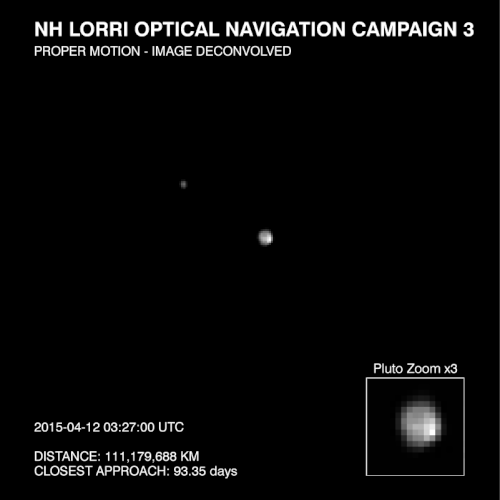
Image: The 3x-magnified view of Pluto highlights the changing brightness across the disk of Pluto as it rotates. Because Pluto is tipped on its side (like Uranus), when observing Pluto from the New Horizons spacecraft, one primarily sees one pole of Pluto, which appears to be brighter than the rest of the disk in all the images. Scientists suggest this brightening in Pluto’s polar region might be caused by a “cap” of highly reflective snow on the surface. The “snow” in this case is likely to be frozen molecular nitrogen ice. New Horizons observations in July will determine definitively whether or not this hypothesis is correct. Credit: NASA/Johns Hopkins University Applied Physics Laboratory/Southwest Research Institute.
The zoomed image is particularly notable because it clearly shows different brightness patterns as Pluto rotates (and notice that Charon, even though much smaller, still stays relatively the same, an indication that the surface is more uniform than Pluto, or as Stern said in the news conference, more ‘muted’). An apparent oddity here actually is not — If you look closely at the view of Pluto in the center, it seems to show an uneven surface. This is only an optical effect caused by the fact that dark areas on the dwarf planet are rotating in and out of view.
These albedo changes on the Plutonian surface are striking. Stern commented that it was unusual that we should be seeing such marked effects from such a distance. The possibility of a polar cap — probably made of highly reflective frozen molecular nitrogen ice — seems strongly enhanced by the bright area that persists in the zoomed image. JHU/APL has used a mathematical technique called deconvolution to improve the resolution of the raw LORRI images, giving us about the best resolution the camera and detector can provide at this distance.
And speaking of raw images, be aware of the raw image archive being assembled on the LORRI Images from the Pluto Encounter page. The imagery is, of course, going to keep getting better. We’ll get another sequence from an observing run scheduled for May 8 through May 14, producing another ‘movie’ in mid-May, followed by a lengthy pause to downlink the data that lasts until the 27th. The spacecraft will then begin observing Pluto/Charon daily at ever higher resolution. Fasten your seat belt.
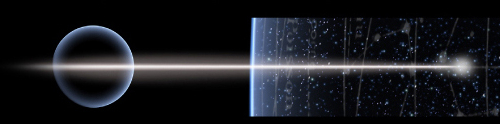
by Paul Gilster | Apr 29, 2015 | Exoplanetary Science |
We’ve found a lot of planets far away from the Sun but know comparatively little about what may be circling nearby stars. The rationale is clear: The Kepler mission’s field of view was carefully chosen to provide a large sample (over 145,000 main sequence stars) that could be studied for transits by the spacecraft’s photometer. Looking out along the Cygnus arm of the Milky Way, far enough from the ecliptic plane to avoid the Sun, the Kepler stars have been providing statistical data to help us understand how common planets actually are in the galaxy.
But as we saw with the announcement of a candidate planet around Alpha Centauri B, the news of planets closer to home excites interest. These are places close enough to us that they could conceivably be the targets of future interstellar probes. As we continue to look at the Kepler inflow, we’re also anticipating missions like TESS (Transiting Exoplanet Survey Satellite), scheduled for a 2017 launch, and PLATO (PLAnetary Transits and Oscillations of stars), scheduled for 2024, both given the charter of finding habitable planets around nearby stars.
But we’re finding nearby worlds even now, as witness the discovery of two planets around the star HD 7924, a K-class main sequence star about 55 light years away in Cassiopeia. A single super-Earth was found here in 2009 using the 10-meter Keck I telescope working in conjunction with the HIRES spectrograph. Now further observations with Keck and a campaign using the Automated Planet Finder (APF) Telescope at Lick Observatory have uncovered two more planets in this system, making for a trio of ‘super-Earths.’
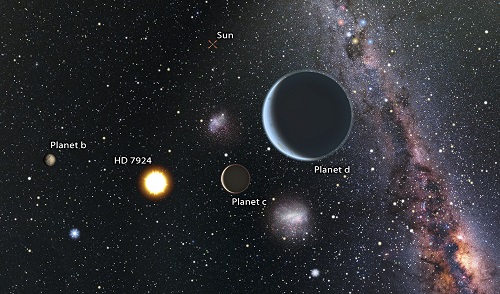
Image: Artist’s impression of a view from the HD 7924 planetary system looking back toward our Sun, which would be easily visible to the naked eye. Since HD 7924 is in our northern sky, an observer looking back at the sun would see objects like the Southern Cross and the Magellanic Clouds close to our sun in their sky. Credit: Karen Teramura & BJ Fulton, UH IfA.
The robotic contribution to this work is a story in itself. We’re looking at a new degree of automation in the exoplanet hunt with Earth-based observatories, one that co-author Andrew Howard (University of Hawaii) likens to “owning a driverless car that goes planet shopping.” The Automated Planet Finder instrument at the Lick Observatory operates robotically every clear night of the year, the installation consisting of a 2.4-meter automated telescope and the high-resolution Levy spectrometer, searching a preprogrammed list of nearby stars using radial velocity methods in the hunt for low-mass rocky worlds.
Lead author Benjamin Fulton (a University of Hawaii graduate student) notes that robotic searches like these can run all night without human oversight:
“We initially used APF like a regular telescope, staying up all night searching star to star. But the idea of letting a computer take the graveyard shift was more appealing after months of little sleep. So we wrote software to replace ourselves with a robot.”
The Automatic Photometric Telescope (APT) at Fairborn Observatory in Arizona also factored in. Now in operation for fourteen years, APT is one of a cluster of automated instruments at the observatory. Its observations of the brightness of HD 7924 complemented the APF and Keck data to confirm the new planets, both of which are seven to eight times the mass of Earth and, in a configuration we’re now finding common, orbiting close to their host star, with periods of 15.3 and 24.5 days. They join the previously discovered super-Earth with a period of a scant 5 days.
What to make of close-in super-Earths like these? From the paper (internal references omitted for brevity):
The large population of super-Earths orbiting close to their host stars was a surprise. Population synthesis models of planet formation had predicted that such systems would be rare. Planet cores were expected to mostly form beyond the ice line and rarely migrate to close orbits unless they first grew to become gas giants. Nevertheless, close-in, low-mass planets are common and often appear in compact multi-planet systems. Theoretical models are catching up, with refinements to the disk migration and multiplanet dynamics in the population synthesis family of models. A new class of “in situ” formation models have also been proposed in which systems of super-Earths and Neptunes emerge naturally from massive disks.
The first HD 7924 planet discovered (2009) grew out of the Eta-Earth Survey at Keck, in which Howard and colleagues searched for planets from a field of 166 nearby G and K dwarf stars. The team is now observing a subset of the Eta-Earth Survey stars with the Automated Planet Finder, with the two new HD 7924 worlds emerging from this work. With incident irradiation values 114, 28 and 15 times that of the Earth for the three planets, they’re not habitable by our standards of liquid water on the surface. But transits cannot be ruled out here and the paper adds that HD 7924 will be an excellent candidate for the James Webb Space Telescope. The upcoming launch of TESS offers the chance to observe the star closely for transit signatures.
The paper speaks of building a census of small planets in the local stellar neighborhood (within 100 light years) as the Automated Planet Finder continues its operations. There are doubtless many planets to find, considering Kepler’s discovery of so many compact, multi-planet systems.
The paper is Fulton et al., “Three super-Earths orbiting HD 7924,” accepted for publication in the Astrophysical Journal (preprint). But see also Howard and team’s 2010 paper on the Eta-Earth findings. It’s “The Occurrence and Mass Distribution of Close-in Super-Earths, Neptunes, and Jupiters,” Science Vol. 330, No. 6004 (2010), pp. 653-655 (abstract). This University of California Observatories news release is also helpful.
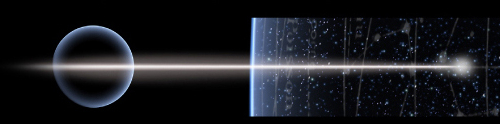
by Paul Gilster | Apr 28, 2015 | Communications and Navigation |
We need to improve the way we handle data tracking and deep space navigation. While the near term is always uncertain because of budgetary issues, we can still take the long view and hope that we’re going to see a steadily increasing number of robotic and human spacecraft in the Solar System. That puts a strain on our existing facilities, and a premium on any methods we can find to make data return more precise and navigation more autonomous.
With these ideas in mind, keep your eye on the Deep Space Atomic Clock (DSAC). It’s a NASA technology demonstrator mission being built to validate a miniaturized, ultra-high precision mercury-ion atomic clock that researchers believe will be 100 times more stable than today’s best navigation clocks. Managed at the Jet Propulsion Laboratory, the DSAC has been tweaked and improved to the point where it allows drift of no more than a single nanosecond in ten days.
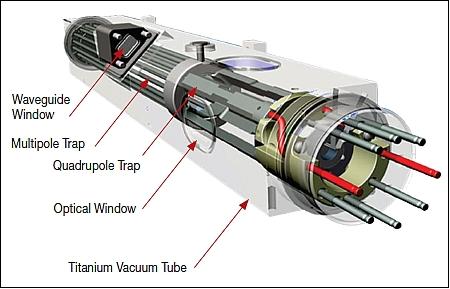
Image: Drawing of the DSAC mercury-ion trap showing the traps and the titanium vacuum tube that confine the ions. Credit: NASA/JPL.
We need improved atomic clocks in space to take spacecraft navigation to the next level and permit the next generation of studies of distant targets like Europa. Assuming Europa does have a subsurface ocean, this body of water will clearly be affected by tidal effects from Jupiter. Atomic clock measurements of DSAC’s caliber will be needed to provide the tracking data we’ll use to estimate Europa’s gravitational tide, helping to confirm the characteristics of its putative ocean. More on this thought and on the background of the DSAC in this JPL news release.
Europa, of course, is but one target whose investigation will be enhanced by projects like DSAC. Beyond this, improving the accuracy and stability of atomic clocks can change the way deep space navigation is done. Right now, we use a two-way paradigm for radiometric tracking, meaning that the same ground-based frequency standard is used as a reference for an uplink signal and a downlink detector. In other words, we track a spacecraft with our network on Earth and a ground-based team performs the necessary navigation. Improving the DSAC to allow it to operate in deep space will create a one-way tracking paradigm and autonomous navigation.
Think of the GPS unit you probably use to navigate with when you drive. GPS offers a one-way signal requiring no return signal from your car. The goal is to create the same one-way capability in deep space navigation. The smaller clock error (and DSAC is expected to be stable to less than 3 X 10-15 at one day, as measured by its Allan Deviation, a measure of frequency stability) enables one-way tracking with accuracies equal to or better than the two-way methods we currently use, a more flexible and efficient space navigation system.
The benefits of such a system will be striking, particularly in scenarios where we have several spacecraft either in orbit around or on the surface of a planet (Mars is the obvious reference for now). Let me pull a quote from Thornton and Border’s Radiometric Tracking Techniques for Deep-Space Navigation (Wiley, 2003):
…a single deep space antenna can acquire one-way Doppler and telemetry simultaneously from all spacecraft. Multiple uplink signals are not required. Consequently, this configuration results in more efficient use of ground-based resources and enhances orbit solutions and lander position estimates through the use of differential measurements.
Moreover, we get better signal-to-noise ratios for receiving spacecraft telemetry. Thornton and Border go on to explain the two reasons for this:
…one-way transmissions provide better short-term (< 1 s) stability, resulting in less signal loss in the detection process. This is because the short-term stability of two-way transmissions is degraded by solar plasma scintillations of the uplink signal and, for more distant spacecraft, by thermal noise in the spacecraft receiver. Second, the ground antennas are configured in a listen-only mode for one-way tracking, whereas the more complicated diplexer mode, required for simultaneous uplinking and downlinking, increases the effective system noise temperature of the ground receiver.
So DSAC technology can be a game-changer for deep space navigation, assuming the system checks out in flight. The plan is for the demonstration unit to be launched in 2016 aboard a SpaceX Falcon 9 Heavy booster, hosted on a spacecraft provided by Surrey Satellite Technologies. The equipment will be operated for at least one year, making use of GPS satellite signals to demonstrate precision orbital determination. Todd Ely (JPL), principal technologist for the DSAC Technology Demonstration Mission, describes the testing:
“Our in-orbit investigation has several phases beginning with commissioning, where we start up the clock and bring it to its normal operating state. After that we’ll spend the first few months confirming and updating our modeling assumptions, which we will use to validate the clock’s space-based performance. With these updates and our observation data, we’ll spend the next few months determining DSAC’s performance over many time scales…from seconds to days.”
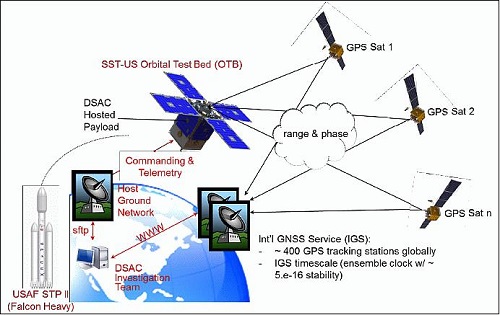
Image: Overview of the mission architecture. Credit: NASA/JPL.
Following that period, the team will monitor clock telemetry to characterize its potential for long-term operations. The initial DSAC flight aims at producing the data that will help to make future units smaller and more efficient, readying them for the lengthy mission times that exploring deep space will demand. The kind of tracking data such a refined atomic clock will make available will improve spacecraft navigation and allow the precise tracking data we’ll need as we explore the moons of the gas giants and prepare for future targets even further out.
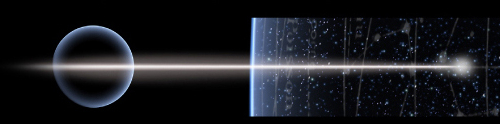
by Paul Gilster | Apr 27, 2015 | Exoplanetary Science |
It’s the twentieth anniversary of the discovery of 51 Pegasi b, a ‘hot Jupiter’ that was the first planet to be discovered around a normal star. I always have to throw in that ‘normal’ qualifier because it was in 1992 that Aleksander Wolszczan and Dale Frail announced their discovery of planets around the pulsar PSR 1257+12, the first extrasolar planets ever found, and an extraordinary discovery in themselves. Michel Mayor and Didier Queloz announced the 51 Pegasi b discovery in 1995, and it was quickly confirmed by Geoff Marcy and Paul Butler.
51 Pegasi b, some 50 light years from Earth in the constellation Pegasus, is the prototypical ‘hot Jupiter,’ a gas giant orbiting in tight proximity to its star. A new paper from Jorge Martins (Universidade do Porto, Portugal) and team announces another first for this world, the first detection of the spectrum of visible light reflected off an exoplanet. The detection was made by painstakingly removing the host star’s spectrum to reveal the spectrum of the (by comparison) extremely faint planet.
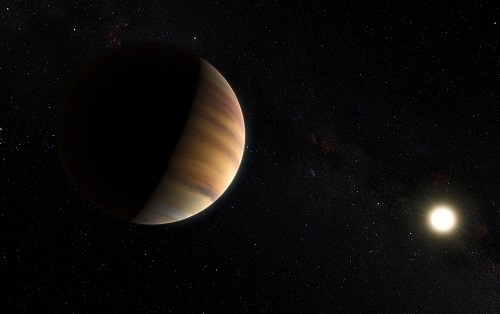
Image: This artist’s view shows the hot Jupiter exoplanet 51 Pegasi b, sometimes referred to as Bellerophon, which orbits a star about 50 light-years from Earth in the northern constellation of Pegasus (the Winged Horse). This was the first exoplanet around a normal star to be found in 1995. Twenty years later this object was also the first exoplanet to be be directly detected spectroscopically in visible light. Credit: ESO/M. Kornmesser/Nick Risinger (skysurvey.org).
This is intriguing work because until now we’ve been largely confined to studying exoplanet atmospheres through a method called transmission spectroscopy, which allows scientists to study starlight filtered through the atmosphere during a transit. Also in common use is occultation photometry and spectroscopy, which measures the depth of the secondary eclipse as the planet passes behind the star (again, we’re talking about a transiting planet). Occultation spectroscopy is particularly useful in gauging the heat signature of the exoplanet.
The method deployed by Martins and team uses what is known as the cross-correlation function to extract meaning from the combined spectra of star and planet. Correlation is a mathematical operation that uses two signals to produce a third signal — this third signal is the cross-correlation of the two inputs. Using their spectroscopic data, Martins and team could amplify the signal of the planet and then remove the stellar signal to reveal the planet’s spectrum. The paper describes the basic principle at work:
…the cross-correlation function (hereafter CCF) can be used to mathematically enhance the S/N [signal-to-noise ratio] of our observations to a level where the extremely low S/N planetary signal can be recovered. The CCF of a spectrum with a binary mask (Baranne et al. 1996) has been extensively tested in detecting exoplanets with the radial velocities method. Briefly, this technique corresponds to mapping the degree of similarity between the stellar spectrum and a binary mask (representing the stellar type), which increases the S/N of the data by a factor proportional to the square root of the number of spectral lines identified in the mask.
The work was performed using the European Southern Observatory 3.6 meter telescope in conjunction with the HARPS spectrograph at La Silla (Chile). The results reveal that 51 Pegasi b is about half as massive as Jupiter although somewhat larger than Jupiter in diameter, with an orbital inclination of about nine degrees to the direction of the Earth. That means that the planet’s orbit is close to edge-on as seen from Earth, although not close enough to allow a transit. Remember, 51 Pagasi b was a radial velocity discovery, and the method used by Martins and his colleagues is not dependent on transits, unlike transmission and occultation spectroscopy.
Exposed to the intense starlight of its host star in such a tight orbit, the planet is highly reflective. And the fact that these properties can be deduced from the spectroscopic technique he has developed is promising for the future, as Martins explains:
“This type of detection technique is of great scientific importance, as it allows us to measure the planet’s real mass and orbital inclination, which is essential to more fully understand the system. It also allows us to estimate the planet’s reflectivity, or albedo, which can be used to infer the composition of both the planet’s surface and atmosphere.”
Moreover, the fact that existing equipment could produce these results points to the potential of future instruments on larger telescopes, such as the Very Large Telescope and the planned European Extremely Large Telescope. The ESPRESSO spectrograph (Echelle SPectrograph for Rocky Exoplanet and Stable Spectroscopic Observations) will greatly exceed the HARPS instrument to produce radial velocity measurements that can reveal Earth-like planets. Now being manufactured, the goal for first light of ESPRESSO on the VLT is 2016. The paper notes what the combination of advanced instruments and this new spectroscopic method means:
The sheer increase in precision and collecting power will allow for the detection of reflected light from smaller planets, planets on orbits with longer periods, or an increase in detail for larger planets like 51 Peg b.
The paper is Martins et al., “Evidence for a spectroscopic direct detection of reflected light from 51 Peg b,” 576 (2015), A134 (abstract / preprint). Martins’ previous paper describing the use of the cross-correlation function is Martins et al., “Spectroscopic direct detection of reflected light from extrasolar planets,” Monthly Notices of the Royal Astronomical Society, Vol. 436, Issue 2 (2013). Abstract / preprint.
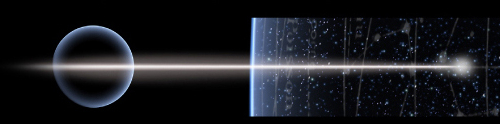
by Paul Gilster | Apr 24, 2015 | Exoplanetary Science |
Yesterday’s look at NExSS (the Nexus for Exoplanet System Science), NASA’s new ‘virtual institute,’ focused on the multidisciplinary nature of the effort. The work I’m looking at today, an analysis of the planets around Tau Ceti performed at Arizona State University, only emphasizes the same point. To get a read on whether two planets that are possibly in Tau Ceti’s habitable zone are likely to be terrestrial worlds like Earth, the ASU team brought the tools of Earth science into play, in particular the work of Sang-heon Shim.
Shim is a mineral physicist who worked with astrophysicists Michael Pagano, Patrick Young and Amanda Truitt in the Tau Ceti analysis. His perspective was vital because early work had already suggested that Tau Ceti has an unusual balance between the rock-forming minerals magnesium and silicon. In fact, the ratio of magnesium to silicon here is 1.78, about 70% more than we find in the Sun. That casts long-standing views of Tau Ceti as Sol’s twin into doubt, and raises questions about the nature of the planets that formed around it.
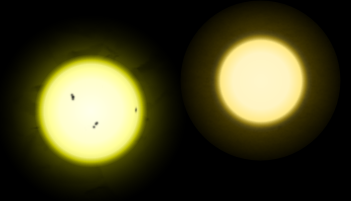
There is evidence for five of these, with two — Tau Ceti e and f — thought to be in the habitable zone. That’s an attractive possibility, for Tau Ceti is nearby at 12 light years, a solitary G-class star like the Sun, and a relatively stable one at that. No wonder it figures prominently in science fiction, its very proximity made a significant plot issue by Larry Niven in his 1968 novel A Gift from Earth, which depicts an isolated Tau Ceti colony that can still receive the occasional cargo from Earth. Isaac Asimov made a Tau Ceti planet the home of the first human extrasolar settlement in The Caves of Steel (1954).
Image: The Sun is at the left in this comparison with Tau Ceti. Credit: R.J. Hall via Wikimedia Commons.
In fact, I can think of few stars that have received so much attention from writers. Might some of the planets there really be habitable? The two planets we are looking at are ‘super-Earths,’ with masses of 4.29±2.00 and 6.67±3.50 times that of Earth respectively. The new work makes the prospect of Earth-like conditions unlikely. In fact, Shim’s mineralogical study indicates that the high magnesium/silicon ratio of the parent star could produce planets unlike any we’re familiar with, as the scientist explains:
“With such a high magnesium and silicon ratio it is possible that the mineralogical make-up of planets around Tau Ceti could be significantly different from that of Earth. Tau Ceti’s planets could very well be dominated by the mineral olivine at shallow parts of the mantle and have lower mantles dominated by ferropericlase.”
Ferropericlase is a magnesium/iron oxide that is thought to be a major constituent of the Earth’s lower mantle, along with silicate perovskite, which is a magnesium/iron silicate. Because ferropericlase is viscous, an abundance of it in the mantle would make mantle rock flow more readily, possibly affecting plate tectonics and volcanism at the planetary surface. The resulting world would pose challenges for the development of life, and certainly for its detection. “Faster geochemical cycling,” the paper notes, “could impede the buildup of biologically produced non-equilibrium chemical species in the planet’s atmosphere.”
The paper describes what it calls a detectability index, or DI, that gauges the ability of a planet to house life and to maintain biosignatures of the kind we hope to detect with new space telescope missions. Tau Ceti planets in the habitable zone might, in other words, be habitable, but unlikely to produce detectable life signs in their atmospheres. Life would not necessarily be absent, but detecting it would require a thorough study of planetary evolution.
Another issue is the length of time a planet spends in the habitable zone. Tau Ceti e’s position is deeply problematic. The authors believe the world is reaching the end of its habitable lifetime and is at best on the extreme inner edge of the habitable zone. Tau Ceti f, meanwhile, appears to be near the outer edge of the habitable zone, but evidently moved into it within the past 1.5 billion years, and probably in much less time than this.
Assume even a billion years in the habitable zone and bear in mind that Earth’s biosphere took roughly two billion year to produce biosignatures that would be theoretically detectable. The DI for this world — our ability to find life if it does exist on Tau Ceti f — would be low indeed. A long habitable lifetime may be in this planet’s future, but that doesn’t help us now:
Even in the most pessimistic case, the planet will have about 7 Gy of habitable lifetime until the end of the main sequence, plus additional time while the star traverses the subgiant branch. From a detectability standpoint, however, f is a poor candidate. At best, the planet has been in the HZ for < <1Gy under these assumptions.
So we have in Tau Ceti f a world where life could exist but would be, at least according to what we know of life here on Earth, probably in an early state and unlikely to be detectable. There is a significant lesson for us in this conclusion, as the paper goes on to note:
The rate of change of L [luminosity] and Teff [stellar effective temperature] as a function of time means that cases similar to f where a planet enters the HZ in the latter part of the star’s life are more common than planets that have been in the HZ since early times. This serves as a reminder that the present “habitability” of a planet does not necessarily indicate that it is a good candidate for detecting biosignatures. The temporal evolution of the system must be taken into account.
So much, then, for the notion that if we detect a planet in the habitable zone, we are safe in assuming life has had time enough to emerge there. Here we have one case of basic mineralogy casting doubt over whether surface conditions are habitable or whether it could be detected by our instruments if present, and another where the movement of a planet into the habitable zone means that biology may flourish there but only in our distant future.
The paper is Pagano et al., “The Chemical Composition of τ Ceti and Possible Effects on Terrestrial Planets,” Astrophysical Journal Vol. 803, No. 2 (2015), Art. 90 (abstract / preprint).
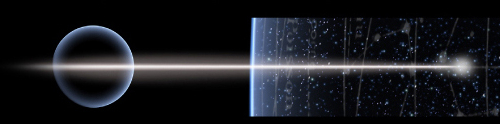