by Paul Gilster | Apr 26, 2019 | Deep Sky Astronomy & Telescopes |
The publication of a paper called “New Features of Parenago’s Discontinuity from Gaia DR1 Data” by V. V. Vityazev and colleagues brought us a new look at an unusual observation. Parenago’s Discontinuity refers to the fact that red, cooler stars move faster in the direction of galactic rotation than blue, hotter stars, based on Hipparcos data. But is the phenomenon just a chance, local observation? Fortunately, a much larger dataset from the Gaia mission has now become available, and it is this that the Vityazev paper addresses in terms of Parenago’s finding. The following dialogue between Greg Matloff and Alex Tolley goes to work on the Vityazev document. Dr. Matloff has pointed to the Discontinuity as a possible marker of consciousness among low temperature stars, where molecular bonds can form.
Could motion be a matter of agency in such stars? Greg explored the idea in his book StarLight, StarBright. Now Alex digs into the Vityazev paper and questions whether Greg is right that his controversial theory can be falsified given our data. Various mechanisms for stellar motion are explored, ranging from coronal mass ejections to possibilities that are downright Stapledonian. How exactly would a civilization go about moving stars? I am preserving the dialogue format of the original correspondence as a case in point of serious differences being discussed in a way that both disputants have found valuable.
By Greg Matloff and Alex Tolley
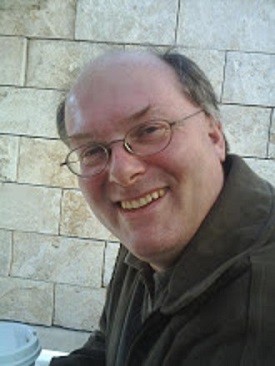
Alex: Greg, in your 2012 Centauri Dreams post Star Consciousness: An Alternative to Dark Matter, you made the claim that there was an alternative explanation to dark matter for the velocity of stars around the galactic center. Your hypothesis was some sort of psychokinesis effect generated by consciousness, even if a primitive one. You used the Parenago Discontinuity (PD), which showed that velocity was related to star type, to suggest that fast stars were cool and that these cool stars could have chemistry, allowing some sort of consciousness. The 2015 Centauri Dreams post Greg Matloff: Conscious Stars Revisited further elaborated on this hypothesis.
The first problem I have with the data you used is that there was no plateau of velocity, but rather a peak, unlike the classic Parenago Discontinuity, which showed a plateau. This would imply that star types reach a peak velocity, then decline. Why would there be such an effect if consciousness was the driver of some psychokinetic effect? A possible explanation could be the amount of material that could be expelled to propel the stars.
The issue was left on whether the Parenago Discontinuity was purely a local, rather than galactic effect. We awaited the Gaia data.
The Vityazev paper using Gaia data confirmed that the Parenago Discontinuity was indeed non-local and that the peak effect around B-V of 0.7 seen with the earlier data you presented was indeed correct. As you had hung the hypothesis on the Parenago Discontinuity, this confirmation with the larger data did not falsify the interpretation that stars might be conscious.
The Hipparcos satellite measured the distances to hundreds of thousands of stars. It also measured the magnitude of each star through two filters:
A blue filter, yielding a B (for “blue”) magnitude
A greenish filter, yielding a V (for “visual”) magnitude (the human eye has highest sensitivity in the green)
Now, astronomers call the difference between the B and V magnitude of a star its (B-V) color, or sometimes just “color” for short. Remember how magnitudes work: large numbers mean “faint”, and small numbers mean “bright”. What is the “(B-V) color” of a hot star?
Figure 5 in the Vityazev paper seems to offer the basis for the more likely explanation of the PD results.
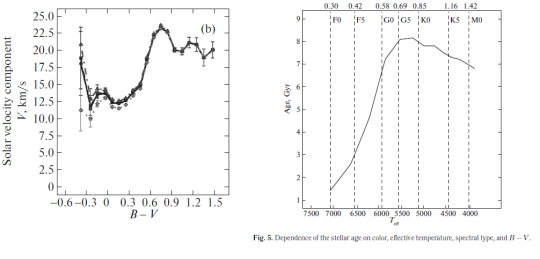
The figure 1b graph (left) shows the PD with the Gaia data. The figure 5 chart (right) shows that the PD effect is correlated with stellar age, rather than stellar temperature. The cooler stars in the sample are actually younger than G stars at the age peak. The shape of the B-V vs age graph matches the B-V vs azimuthal velocity (V) graph. This seems to me to suggest that velocity is therefore related to age, and more likely due to some physical factor related to time.
This seems to me to be the most parsimonious relationship and should be chosen by Occam’s razor. The stellar conscious hypothesis has to account for this age relationship and explain why the coolest stars that should have good consciousness and can, for unknown reasons and unknown mechanisms, chase around the galaxy faster than large, hotter stars, nevertheless go more slowly than G-type stars.
Why stars increase velocity with age still needs elucidation and modeling. However, the Vityazev paper has broken down the many components of stellar kinematics. This should provide a nice set of constraints for validating computational models.
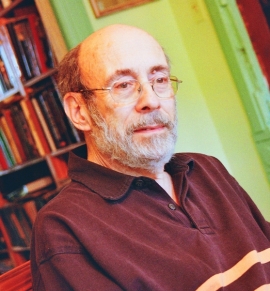
Greg: The fact that Parengo’s Discontinuity is apparently non-local supports my hypothesis and does not support density waves. I have gone through the Messier, Herschel, and NGC Catalogues and reported my results in several publications. No known diffuse nebula in our galaxy or the Clouds of Magellan is large enough to drag along stars over a ~1,000 light-year radius sphere. The fact that stars seem to move faster along the direction of galactic motion as they age is very interesting, but it does not bear upon my original hypothesis that the discontinuity occurs at the point where molecules come in to stellar spectra. It is in my data as well as the data in the paper, so I presume that it is correct. I had originally ignored it because it does not bear on the original hypothesis. I presented in my original letter to Paul and others the causes I could think of to explain this phenomenon. But please, don’t hesitate to present others.
I am pretty happy right now because I predicted a non-local Parenago Discontinuity and this has apparently been verified. No one else, to my knowledge, had made this prediction. The local alternative explanations are now extinct. But science works by constructing alternative explanations for a phenomenon and testing them. Please do so. Try your hand at galaxy-wide possible alternative explanations. I can think of a few that won’t work. I am very interested in what you and others come up with and look forward to a dialogue of competing ideas.
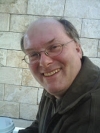
Alex: The chart below is a rough conversion of the B-V values to age (from Figure 5) and the velocity from figure 1b. This seems to show that the Parenago Discontinuity might be a phenomenon based on using a variable (stellar type) that isn’t really related to the star’s velocity. Using age provides an approximately linear trendline with an R-squared of 0.93.
I accept that this velocity might be hard to explain (I am certainly not able to comment on this) but the relationship of age to velocity does seem to point to a simpler explanation for V based on natural forces, rather than requiring agency.
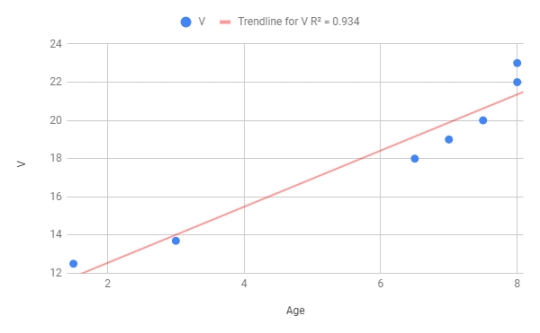
This older reference looked at A and B stars and examined the velocity differences based on age. There were clear differences based on age, rather than stellar type. While this doesn’t invalidate your hypothesis, they do have a physical explanation on why age impacts star velocity. To my mind, such approaches should be examined further before resorting to more exotic explanations. With the Gaia data, astronomers involved in kinematics studies now have a trove of high-quality data to test their models. I would be looking amongst that cadre for cutting-edge modeling.
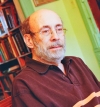
Greg: Nice analysis! What I tried to do is put all the cards, as I see them, on the table. I am not and never have been an advocate for psychokinesis. But science seems willing to accept the Multiverse, which can perhaps not be verified. It accepts dark matter and invokes many properties for this stuff even though it remains hidden. I have discussed PK and the Geller/Randi controversy with people on both sides and find nothing wrong with their arguments. So I believe, with David Kaiser of MIT and others, that the case should be reopened. If a weak PK force can be demonstrated to the satisfaction of the scientific community, it should be considered an option.
I also believe that the stellar age variation has little to do with Parenago’s Discontinuity. Why does the discontinuity appear at the same place where molecules come in to stellar spectra?? And why indeed is it a discontinuity instead of a smooth curve? Invoking strange (and unknown) stellar variability to explain it is certainly an exotic explanation!
One of the problems with what we are discussing comes from the fact that I was checking the metaphysics of Olaf Stapledon, a sci-fi author and philosopher, and attempting to see if there is something scientific behind his discussion in Star Maker. If I were starting afresh, I would title my work (as I have done in subsequent papers) “A Test of Self-Organization on the Galactic Scale?”, since astrophysicist Erich Jantsch uses “self-organization” as a possible explanation of consciousness. Alternatively, I might go with the terminology of philosophers such as David Chalmer and title my papers “Is Panpsychism a Science?”
I think that the work that you have done on (B-V) vs. star age from the Gaia Release 1 sample is invaluable and should be included in our published dialogue. I hope very much that our debate leads to further work with future Gaia data releases.
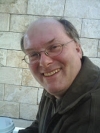
Alex: Let me give you a perspective from a biologist’s point of view. The Parenago Discontinuity might have an analogy to living vs non-living, where living things can be observed to grow, move (or have tropisms), reproduce, etc. Let us assume that cooler stars have some sort of motility which is driven by a “need”, perhaps acting something like a bacterium. Bacteria can be observed to move faster in their medium if they have flagella. A biologist would ask “what is the evolutionary advantage of movement given the cost” and might hypothesize that this leads to faster food discovery, or a phototropism, or evasion of larger predators. In the case of cool stars, I would be asking similar questions. Why is V (azimuthal velocity) the key variable, rather than a different direction (U, W)?
Given that V is affected by star type, what is the advantage of moving faster in one direction? One might hypothesize that this facilitates sweeping up of neutral gas clouds more effectively, but this would be even better if the stars moved in the opposite direction. Are the stars perhaps self-organizing into “flocks” and what we are seeing is the movement of the flock which is currently going in the V direction? With bacteria, we know that it is flagella that allow movement through the medium. With stars, one mechanism is with a Shkadov drive to asymmetrically create thrust. Another might be directed coronal mass ejections (CMEs). In the former case, it is not the star that is sentient, but more likely a separate intelligence, a more Stapledonian idea.
Therefore another hypothesis is that stars are being driven by a galactic wide intelligence[s] to reach a certain unified velocity for some reason. The age relation to velocity then makes sense if the Shkadov drives need billions of years to reach the needed velocity. For what purpose?
One idea might be to facilitate more frequent stellar encounters allowing biological civilizations to disperse more easily among the stars. On the other hand, if the galaxy is an organism or superorganism, perhaps the Parenago Discontinuity is some process to organize older stars for some equivalent of a cellular process.
An obvious question suggests itself. If cooler stars are somehow able to direct their velocity, shouldn’t very cool stars, i.e. red dwarfs and brown dwarfs, also have that behavior? We don’t have enough examples of BDs to determine this, but it would provide another datum. One might extend this to gas giant planets too. While the ones we know about are bound to their stars, is it possible that planetary migration is a related phenomenon? Are “hot Jupiters” exhibiting similar behaviors?
Clearly one can speculate endlessly. If the relevant variable is time rather than stellar type, then this constrains mechanisms to those that require both time and a reason for the more uniform direction. With such a constraint, looking for a natural process that requires billions of years would seem to be the most fruitful direction. That velocity W (fig 1c) is fairly constant, but U (fig 1a) declines with stellar type suggests to me that physical forces are somehow directing the movement into the W direction, whether those forces are natural or artificial. The direction is apparently translating from U, towards the galactic center, to V, a transverse direction, rather like the Coriolis forces operating on weather patterns that “shape” the velocity profile.
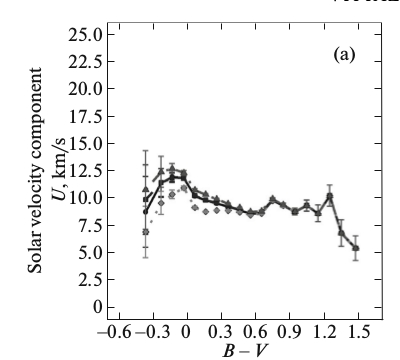
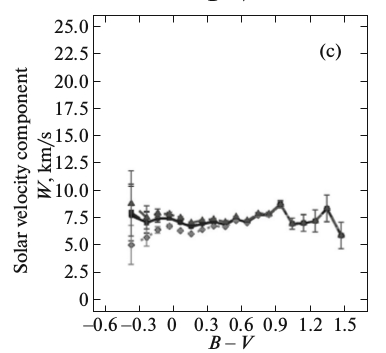
If time is the relevant variable, then looking for young, cool stars should be the best way to test the hypothesis. If they are fast, then stellar type is the key variable, but if slow, then time. The Vityazev paper also has limited data for red giant stars. If anything, the G, K and M red giant stars have more uniform V than main sequence stars, i.e. they seem to conform more closely to the classic PD plateau. Whatever the mechanism, size is clearly not a variable as they have velocities comparable to the oldest G stars. Creating an age chart for these stars might also be instructive.
Unfortunately, even if age is the relevant variable, it doesn’t falsify your hypothesis. The mechanism of acquiring a high V might simply require time, whether a natural, external force or an inner, self-directed one. Just as with determining which animals are conscious (e.g. using the mirror test), we need a better test to be able to falsify your hypothesis. We may be in the position of trying to determine sentience of a herd of buffalo from a still photograph when what we need is video or many photographs over time.
PS: A back-of-the-envelope calculation shows that a star could attain the necessary velocity by directing its radiant energy (e.g. Shkadov drive), but not by directed coronal mass ejections. (calculation for our sun).
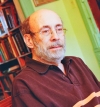
Greg: Wonderful! My feeling has always been that stars need not have a high level of consciousness–they could be like slime-mold amoeba or developing cells in embryos.
I suspect that Vityazev et al could not use the red giant Gaia data for the same reasons the Burnham’s validation of Parenago for giants looks so fluffy. Since most of these stars are very distant, astrometric parallax is impossible with Hipparchus and the early Gaia data.
Spectroscopic distance estimates using the HR diagram are accurate to 10% or less. Also, what is the local reference standard when the subject stars occupy a sphere thousands of parsecs in radius? Hopefully, future Gaia data releases will offer the possibility of greater accuracy.
Also, I have been thinking about your suggestion regarding unknown forms of stellar variability. My suggestions in the earlier emails–EM radiation anisotropy and unidirectional jets in mature stars–are two of these. Perhaps there are others!
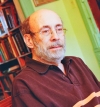
Greg: The Shkadov thruster could of course be a form of anisotropic EM emission from the star. But could such a thruster develop in a purely materialistic setting not requiring a Kardashev Type 2 civilization in that star’s system or volition on the part of the star?
It is so strange that we are encountering so many fascinating intellectual insights at the same time that national governments are failing. Last Sunday we attended a talk by Tyler Volk, a biologist at NYU. He has constructed a geometric/architectural model of self-organization from the quark to universal levels. Tyler suspects that we are moving towards the planetary phase of our civilization and this is the use of apparent political dysfunction. I hope he is correct.
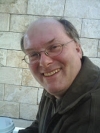
Alex: Greg, you write: “The Shkadov thruster could of course be a form of anisotropic EM emission from the star. But could such a thruster develop in a purely materialistic setting not requiring a Kardashev Type 2 civilization in that star’s system or volition on the part of the star?”
I would be skeptical about EM emission asymmetry as I would have thought this would be detectable. A Shkadov drive with mirrors would definitely have some observable effects. Even just some “magical” control over EM emissions should be detectable.
While CMEs are not energetic enough, a small star with large CMEs that are accelerated to c might just work. But again, I would think such a beam would be detectable. I agree with you that such EM or particle control would need K2 civilizations to manage. With so many stars that would be exhibiting such high V’s, just by chance we would detect some anomalies, and solve the SETI question too.
It also occurs to me that since our galaxy has experienced a collision with another galaxy or satellite in the remote past, with a corkscrew effect on velocities, is there any chance this might in some way be responsible for the Parenago Discontinuity?
Let me refer to a post here on Centauri Dreams titled Gaia Data Hint at Galactic Encounter. I could imagine that an old collision with another galaxy or similar massive object accelerated stars which retained their higher velocities while younger stars maintain the velocities of birth in a more stable galaxy post-collision. To get the age-related relationship, it suggests that the collision must have had its impact on velocity over billions of years. Perhaps the values of U, W and V can be used to determine the likely form of the collision?
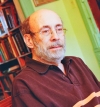
Greg: Regarding the Shkadov thruster discussion, have you read the Benford/Niven novels Bowl of Heaven and Shipstar? These discuss an advanced version of this concept. I have given some thought to a galactic collision producing Parenago’s Discontinuity. According to the computer simulations done at Cornell (and likely other places), when the Milky Way collides with Andromeda to form Milkimedia (what a horrible name!), there will be widespread disruption of diffuse nebulae. This might result in a Spiral Arms Density Wave effect drawing lower-mass stars along faster than their more massive colleagues.
But the same simulations indicate that the combined galaxies lose their spiral shapes after merger. The ultimate result is a giant elliptical with few nebulae and a very low rate of star formation. Since this has clearly not occurred for the Milky Way galaxy (which is a nice barred spiral), it is reasonable to conclude that such a galactic merger did not happen. From what I understand, galactic cannibalism (where a big galaxy gobbles a smaller one) has less disruptive effects–the big guy retains its shape (which might be an argument for self-organization on the galactic scale.
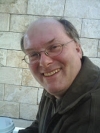
Alex: From an engineering point of view, I think controlling the solar wind might be a lot easier than deflecting the EM with a solid mirror. I would envisage surrounding the star in a charged, superconducting “mesh” to trap the protons. These would be funneled to electromagnetic accelerators to emit the protons in a unified direction at near c velocity. That might be enough to accelerate the star over the needed timescales. The advantage is lower mass requirements and no impact on the star’s light emissions or spectrum. However, I would expect that the proton beam emitted would be detectable. Think of this drive as a giant ion engine coupled with a ramscoop that captures the protons from the star, rather than from the interstellar medium as in a Bussard ramjet.
The Centauri Dreams link I provided was for a proposed “impact” with a dwarf galaxy (the Sagittarius dwarf galaxy). Much smaller than M-31, and if there was an impact, it certainly hasn’t disrupted the shape of our galaxy. What is interesting is the analysis technique that teased out the spiral shape of the velocity-position phase space that was too subtle to visualize with pre-Gaia data. This sort of analysis might offer a better way to understand the stellar type/age vs velocity relationship. Figure 2 in the paper referenced in the article shows the structure in the azimuthal (V) direction vs the radius (I cannot interpret if this has any possible bearing at all on the Parenago Discontinuity, but the same analysis teasing out stellar type might well do. It might be worth contacting the principal author regarding this approach. Paper attached)
[The paper Alex is referring to is Antoja et al., “A Dynamically Young and Perturbed Milky Way Disk,” Nature 561 (2018), 360-362 (abstract / preprint).]
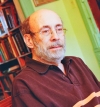
Greg: Nice paper! Thanks for it. But can this effect bear on an apparent near-linear relationship between galactic revolution velocity and star age? I think that such dwarf galaxy absorptions by the Milky Way are rare events. Regarding the Shakdov thruster, you are (I think) correct. But could we detect a proton flow necessary to increase V by ~1 km/s over a 10 billion year time interval? Certainly fuel for thought.
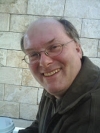
Alex: Given the large numbers of stars in the sample, I was thinking that the stellar type vs velocity relationships could be plotted for locations on the galactic disc. If they are all the same, that would tell a different story than if they differed in shape. There is a hint of this in the Vityazev paper where they plot thin vs thick disk stars that show differences. In other words, given the large sample size, it should be possible to show more granularity based on location than with the averaging that Vityazev computes.
Incidentally, I came to the same conclusion with regards to CMEs. However, even for our sun, the CMEs are within a single order of magnitude if they are accelerated to c. As the solar wind produces more protons overall than CME, the same approach might work. However, this approach has to work for all stars, so the mass loss of protons/star mass has to increase for cooler stars for this mechanism to describe the PD observation.
I am not aware of the idea of moving stars by trapping and accelerating the solar wind and flares, so possibly it is novel. I also wonder if it is possible to direct solar flares by manipulating the surface magnetic fields of the star so that they break and cause a flare in desired positions. As the star rotates, the magnetic field is broken when the alignment is correct, releasing the flare. The flare material still needs to be accelerated to have the desired thrust, so electric fields could do that, or something more like a linear particle accelerator. Definitely K2 or even K3 level engineering…or just maybe some phenomenon than a “living” star could manage.
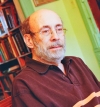
Greg: The differences between disc star velocities and others in the Antoja et al paper is fascinating. I don’t think it bears on Parenago unless somehow large diffuse nebula were created in the galactic merger. These could have perhaps caused differential velocities between massive and less massive stars. But such nebula would have to be perhaps an order of magnitude larger in radius than those in today’s galaxy to encompass a stellar sphere with a radius in excess of 1,000 light years. A further problem is that these structures would have to dissipate in less than 1 billion years, leaving no trace.
But your suggestion of using future Gaia data releases to dig deeper in stellar kinematics is excellent. I hope that someone will check if Parenago’s Discontinuity works for a star sample at a distance, say, of 10,000 light years.
I have done some rough calculations to see if a directional stellar wind or directional coronal mass ejections (CMEs) could accelerate a Sun-mass star by about 1,000 m/s in 1 billion years. Both seem to fail by a few orders of magnitude. But it is most intriguing that stellar flares (and presumably CMEs) are more common in less massive red dwarfs than in Sunlike stars.
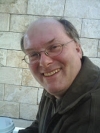
Alex: BoE calculation for thrust of solar wind:
For our sun, redirecting the solar wind to a single direction would only result in the sun traveling ~3 m/s after 1 billion years. However, if the particles were accelerated by 3 orders of magnitude to c, that increases the velocity to 3 km/s. Over 5 billion years, that gets you to 15 km/s, which is in the range of the V needed for the Parenago Discontinuity graph. So physically possible. My first thought is that this would be an engineering solution, rather than a natural one – that is K2 – K3 civilizations. The bias in my thinking is that only human engineering can create craft that can travel at supersonic and hypersonic speeds. Birds cannot even manage 0.1 km/s, while our engineering can propel craft in air 1-2 orders of magnitude faster.
I do have a question about the relative emissions of EM and particles from different stellar types. Isn’t the mass loss vs initial star mass going to be the basically the same for all stellar types? If so, wouldn’t all stellar types reach the same velocity by the time they reach the end of their main sequence period? The Parenago Discontinuity relationship suggests mass or age is important, which implies that the acceleration must be approximately constant for all stars. This would imply, naively perhaps, a force more like gravity, that is exerted over the age of the star. It cannot be a point source outside the galaxy; otherwise the rotation of the galaxy would keep changing the direction of the force. Could it possibly be invoked by the [dark] matter in or surrounding the galaxy? Can this force be computed to suggest a possible cause?
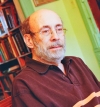
Greg: Very interesting speculation. It’s so funny–we needed Gaia to get beyond a few hundred light years. And this early paper of Gaia results (and I am sure many others) will inspire people to generate designs for even more capable space observatories. It seems that every question we investigate and answer results in hundreds of new questions.
I would hesitate to invoke dark matter. It seems to be a catch-all for everything not understood in the universe. I will check out mass loss rates for distant star types. But I don’t know if we have reliable data.
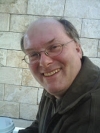
Alex: What is relevant is whether the acceleration is related to stellar type or not. A large, hot star may lose a lot of mass, but it has to accelerate a more massive object. If a star loses X% of its mass over its lifetime, and if the wind speed is fairly uniform across such stars then the final velocity should be much the same for all star types over their lifetimes. The PD requires that this is not the case.
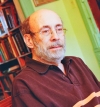
Greg: I just did a Google for “Mass Loss from Main Sequence Stars”. Apparently, this quantity directly depends upon star surface temperature.
I think our dialog has been very fruitful. When I submitted my first paper on the subject to the Journal of the British Interplanetary Society, I had to contend with 4 reviewers. Some of these favored the concept of stellar volition but argued about the inclusion of psychokinetics as a possible explanation. I am neutral regarding this topic, as I have discussed but I am still impressed by the vehemence of the arguments pro and con decades after the Geller/Randi affair. So the fact that accelerated CMEs can provide acceleration of G-type stars (as I agree after a few calculations) indicates that there is an alternative option. I will at some point write up my work on accelerated CMEs and send you the results.
Late this afternoon, weather permitting, we will journey to the Hayden Planetarium, which is hosting a Dutch astronomer who will talk about the latest Gaia results on galactic stellar kinematics. I suspect that Parenago’s Discontinuity is not on the agenda, but I will let you know.
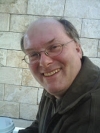
Alex: I note that the Gaia data include all the information needed to create samples of stars within a radius of a position. This could be used to explore the type/mass vs V graphs at the higher granularity that I suggested, particularly radius from the galactic center. This would help confirm that the Parenago Discontinuity is truly global within the dataset and not some averaging, or whether there is spatial structure to the distributions. It may take some computational effort, but it might shed light on possible natural explanations of the PD observation. Sample sets would be easy to crunch on a PC, and the results compiled as a map. For example, the slope of V vs type or mass as a color-coded map.
I was also thinking about the gravity solution. It may work if a point object makes continual passes in the galactic plane. Older stars would experience more passes and if V is due to gravity dragging the stars along during a pass, then this would account for their higher V. A prediction would be that the slope of V would depend on how far from the source the stars are. Dare I say a denser clump of dark matter in a highly elliptical orbit about the galactic center? Data and some modeling would be needed to test this idea.
Out of this discussion, Greg Matloff incorporated many of Alex Tolley’s contributions and produced the short essay below looking at how stars could be accelerated to account for the Parenago Discontinuity.
Physical Methods of Effecting Main Sequence Star Acceleration
Greg Matloff, Nov. 16 2018
Using the Gaia DR1 dataset, Vityazev et al. have investigated Parenago’s Discontinuity for a sample of 1,260,071 main sequence stars [1]. The minimum mean distance for O-k stars in this data release is estimated as 0.15 kiloparsecs, which indicates that the diameter of the sphere containing the subject stars equals or exceeds 1,000 light years. Local explanations for Parenago’s Discontinuity clearly fail.
The analysis reveals that Parenago’s Discontinuity is real. Stars redder than (B-V) about equal to 0.6, which corresponds to spectral class F9 or G0 move faster in their orbits around the center of the Milky Way galaxy than hotter, more massive, bluer stars. Their results are in substantial agreement with the results from Allen’s Astrophysical Quantities and Hipparcos data for a much smaller stellar sample (with a diameter of about 500 light years) presented by Matloff [2].
But Vityazev et al. discuss for the first time a feature present in both data sets. Main Sequence stars apparently speed up in their galactic trajectory as they age. This velocity increment amounts to an increase of about 2 km/s in 8 billion years. The acceleration is approximately equal to 8 X 10-15 m/s2. For a solar-mass star (2 X 1030 kg), the average force exerted on or by the star during the 8 billion year time interval is 1.6 X 1016 N.
Reference 2 presents a number of possible causes for the discontinuity in galactic stellar orbital velocity around (B-V) = 0.6. The analysis presented here considers possible mechanisms that a star might employ to maintain a constant acceleration over a multi-billion year time interval.
1. Acceleration by Nonisotropic Stellar Electromagnetic Emissions
Consider here the possibility that a minded star can control the direction of its electromagnetic emissions. The solar luminosity (Lsun) is 3.9 X 1026 W [3]. Modifying Eq. ((7.2) of Ref. 4, the maximum stellar-radiation pressure acceleration for a Sun-mass star (Msun) is Lsun/ (Msun c) for a unidirectional stellar wind, where c is the speed of light in vacuum (3 X 108 m/s). Substituting in this equation, the maximum solar acceleration from this process is about 7 X 10-13 m/s2.
This acceleration is about 100 times the required stellar acceleration to effect the 1 km/s during a 1-billion year time interval. But if the Sun accelerates using this process, the Solar Constant might vary on an annual basis by up to 1%. Solar Luminosity has been measured to vary by a much smaller amount during the Sun’s 11-year activity cycle.
But the Sun and other main sequence stars generate neutrinos as well as photons as they convert hydrogen into helium deep in the solar (stellar) interiors [3]. Neutrinos have linear momentum as well as very small mass. The solar momentum flux is hard to study because of the very low cross-section of neutrino interactions with detectors. Perhaps stellar neutrino fluxes are not isotropic.
2. Acceleration by Nonisotropic Solar Wind
From Ref. 3, p. 427, the average solar wind velocity is 500 km/s (5 X 105 m/s) and the solar wind carries about 2 million tons of solar matter per second (2 X 109 kg). If all of this material were concentrated in a unidirectional jet, the force exerted on the Sun or Sunlike star is 1015 Newtons. This force is 1/16 the force required for stellar acceleration. This proposed method of stellar acceleration therefore fails.
3. Acceleration by Coronal Mass Ejections
Coronal mass ejections (CMEs) are generally associated with solar flares. Their frequency varies with the solar activity cycle. On average, the mass ejected by CMEs in a Sunlike star amounts to a few percent of the mass ejected in the solar wind and the velocity of a typical CME varies from a few hundred km/s to a few thousand km/s [5]. Unidirectional flares therefore fail as a stellar accelerating mechanism.
4. Acceleration by an Accelerated Solar Wind
Consider next the possibility that a minded Sunlike star can apply its magnetic field to accelerate a unidirectional solar wind to 0.1c (3 X 107 m/s). For the solar wind mass listed above, the kinetic energy of the jet is approximately 1024 W, which is less than 1% of the solar constant. If all of the solar wind is in the jet, the approximate force on the star is 6 X 1016 N. This is about 4X greater than the average force required to accelerate the star.
5. Might the Gravitational Constant of Galactic Mass Vary With time?
Elementary physics students learn that the velocity of an object orbiting a central body varies directly with the square root of the product of the central body’s mass and the Universal Gravitational Constant G. It might be argued that increases in galactic mass or G over billion-year time scales might account for the higher galactic-orbital velocities of older stars.
Surprisingly, as reviewed in an on-line essay by Rupert Sheldrake, experimental measurements of G show some variation [6]. But according to Lorenzo Iorio, such variations are likely caused by experimental errors because the orbits of solar system planets seem relatively constant [7].
A recently published study based upon the Gaia data set demonstrates that within the last 900 million years, at least one dwarf galaxy has passed through and possibly merged with the Milky Way [8]. So it is safe to conclude that the mass of our galaxy may not be constant over multi-billion-year time scales.
But it seems unlikely that either of these two variations can account for the reported increase in stellar galaxy-orbiting velocity with star age. After all, stellar birth nebulae orbit the Milky Way galaxy’s center as do the stars. Infant stars will therefore be accelerated in the same fashion as mature stars by such variations.
6. Conclusions
We see that several of the above suggestions succeed as possible methods of main sequence star acceleration. Perhaps the most intriguing, and the one that might inspire future research, is the possibility that stellar neutrino emissions need not be isotropic.
Psychokinetic (PK) effects have not been considered in the above treatment. Although it is unwise to eliminate PK from consideration for this application and others, it is wise to keep a distance until/unless it can be demonstrated in the laboratory in experiments that can be replicated by other researchers, including skeptics.
Acknowledgement
I greatly appreciate communications and interactions with Alex Tolley. His spirited comments and criticisms were instrumental in preparation of the above discussion.
References
1. V. V. Vityazev, A. V. Popov, A. S. Tsvetkov, S. D. Petrov, D. A. Trofimov and V. I. Kiyaev, “New Features of Parenago’s Discontinuity from Gaia DR1 Data”, Astronomy Letters, 44, 629-644 (2018).
2. G. L. Matloff, “Olaf Stapledon and Conscious Stars: Philosophy or Science?”, JBIS, 65, 5-6 (2012).
3. E. Chaisson and S. McMillan, Astronomy Today, 6th ed., Pearson/Addison-Wesley, San Francisco, CA (2008).
4. G. L. Matloff, Deep Space Probes, 2bd. ed., Springer-Praxis, Chichester, UK (2005).
5. P. Odert, M. Leitzinger, A. Hanslmeier, and H. Lammer, “Stellar Coronal Mass Ejections I. Estimating Occurrence Frequencies and Mass-Loss Rates”, arXiv:1707.02165v2 [astro-ph.SR] 31 Jul 2017.
6. R. Sheldrake, “How the Universal Gravitational Constant Varies.”, www.sheldrake.org. Also in R. Sheldrake, Science Set Free, Deepak Chopra Books, NY (2012).
7. L. Iorio, “Does the Newton’s Gravitational Constant Vary Sinusoidally with Time? Orbital Motions Say No”, arXiv:1504.07233v2 [gr-qc] 16 Dec 2015.
8. T. Antoja, A. Helm, M. Romero-Gomez, D. Katz, C. Babuslaux, R. Drimmel, D. W. Evans, F. Figueras, E. Poggio, C. Reyle, A. C. Robin, G. Seabroke, and C. Soubiran, “A Dynamically Young and Perturbed Milky Way Disk”, arXiv:1804.10196v2 [astro-ph.GA] 24 Sep 2018.
———
The Vityazev et al. paper is “New Features of Parenago’s Discontinuity from Gaia DR1 Data,” Astronomy Letters, Volume 44, Issue 10 (October 2018), pp 629-644 (abstract).
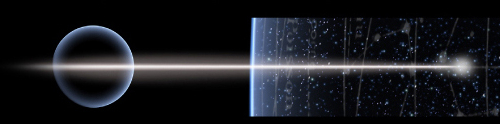