by Paul Gilster | Jul 1, 2022 | Outer Solar System |
While we talk often about subsurface oceans in places like Europa, the mechanisms for getting through layers of ice remain problematic. We’ll need a lot of data through missions like Europa Clipper and JUICE just to make the call on how thick Europa’s ice is before determining which ice penetration technology is feasible. But it’s exciting to see how much preliminary work is going into the issue, because the day will come when one or another icy moon yields the secrets of its ocean to a surface lander.
By way of comparison, the thickest ice sheet on Earth is said to reach close to 5,000 meters. This is at the Astrolabe Subglacial Basin, which lies at the southern end of Antarctica’s Adélie Coast. Here we have glacial ice covering continental crust, as opposed to ice atop an ocean (although there appears to be an actively circulating groundwater system, which has been recently mapped in West Antarctica). The deepest bore into this ice has been 2,152 meters, a 63 hour continuous drilling session that will one day be dwarfed by whatever ice-penetrating technologies we take to Europa.
Consider the challenge. We may, on Europa, be dealing with an ice sheet up to 25 kilometers thick – figuring out just how thick it actually is may take decades if the above missions get ambiguous results. In any case, we will need hardware that can operate at cryogenic temperatures in a hard vacuum, with radiation shielding adequate to the Jovian surface environment. The lander, after all, remains on the surface to sustain communications with the Earth.
Moreover, we need a system that is reliable, meaning one that can work its way around problems it finds in the ice as it moves downward. Here again we need ice profiles that can be developed by future missions. We do know the ice we encounter will contain salts, sulfuric acids and other materials whose composition is currently unknown. And we will surely have to cope with liquid water ‘pockets’ on the way down, as well as the fact that the ice may be brittle near the surface and warmer at greater depths.
SESAME Program Targets Europan Ice
NASA’s SESAME program at Glenn Research Center, which coordinates work from a number of researchers, is doing vital early work on all these problems. On its website, the agency has listed a number of assumptions and constraints for a lander/ice penetrator mission, including the ability to reach up to 15 kilometers within three years (assuming we learn that the ice isn’t thicker than this). For preliminary study, a total system mass of less than 200 kg is assumed, and the overall penetration system must be able to survive three years of operations in this hostile environment.
So far this program is Europa-specific, the acronym translating to Scientific Exploration Subsurface Access Mechanism for Europa. The idea is to identify which penetration systems can reach liquid water. It’s early days for thinking about penetrating Europa and other icy moon oceans, but you have to begin somewhere, and SESAME is about figuring out which approach is most likely to work and developing prototype hardware.
SESAME is dealing with proposals from a number of sources. Johns Hopkins, for example, will be testing communication tether designs and analyzing problems with RF communications. Stone Aerospace is studying a closed-cycle hot water drilling technology running on a fission reactor. Georgia Tech is contributing data from projects in Antarctica and studying a subsurface access drill design, hoping to get it up to TRL 4. Honeybee Robotics is focused on a “hybrid, thermomechanical drill system combining thermal (melting) and mechanical (cutting) penetration approaches.”

Image: A preliminary VERNE design from Georgia Tech showing conceptual component layout. Credit: Georgia Tech.
We’re pretty far down on the TRL scale (standing for Technology Readiness Level), which goes from 1 to 9, or from back of the cocktail lounge napkin drawings up to tested flight-ready hardware. Well, I shouldn’t be so cavalier about TRL 1, which NASA defines as “scientific research is beginning and those results are being translated into future research and development.” The real point is that it’s a long haul from TRL 1 to TRL 9, and the nitty gritty work is occurring now for missions we haven’t designed yet, but which will one day take us to an icy moon and drill down into its ocean.
Swarming Technologies at JPL
Let’s home in on work that’s going on at the Jet Propulsion Laboratory, in the form of SWIM (Sensing With Independent Micro-Swimmers), a concept that, in the hands of JPL’s Ethan Schaler, has just moved into Phase II funding from NASA’s Innovative Advanced Concepts program. The $600,000 award should allow Schaler’s team to develop and test 3D printed prototypes over the next two years. The plan is to design miniaturized robots of cellphone size that would swarm through subsurface oceans, released underwater from the ice-melting probe that drilled through the surface.
Schaler, a robotics mechanical engineer, focuses on miniaturization because of the opportunity it offers to widen the search space:
“My idea is, where can we take miniaturized robotics and apply them in interesting new ways for exploring our solar system? With a swarm of small swimming robots, we are able to explore a much larger volume of ocean water and improve our measurements by having multiple robots collecting data in the same area.”
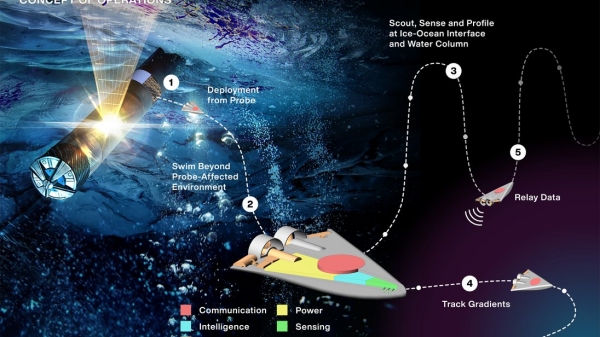
Image: In the Sensing With Independent Micro-Swimmers (SWIM) concept, illustrated here, dozens of small robots would descend through the icy shell of a distant moon via a cryobot – depicted at left – to the ocean below. The project has received funding from the NASA Innovative Advanced Concepts program. Credit: NASA/JPL-Caltech.
We are talking about robots described as ‘wedge-shaped,’ each about 12 centimeters long and 60 to 75 cubic centimeters in volume. Space is tight on the cryobot that delivers the package to the Europan surface, but up to 50 of these robots could fit into the envisioned 10-centimeter long (25 centimeters in diameter) delivery package, while leaving enough room for accompanying instruments that will remain stationary under the ice.
I mentioned the Johns Hopkins work on communications tethers, and here the plan would be to connect to the surface lander (obviously ferociously shielded from radiation in this environment), allowing an open channel for data to flow to controllers on Earth. The swarm notion expands the possibilities for what the ice penetrating technology can do, as project scientist Samuel Howell, likewise at JPL, explains:
“What if, after all those years it took to get into an ocean, you come through the ice shell in the wrong place? What if there’s signs of life over there but not where you entered the ocean? By bringing these swarms of robots with us, we’d be able to look ‘over there’ to explore much more of our environment than a single cryobot would allow.”
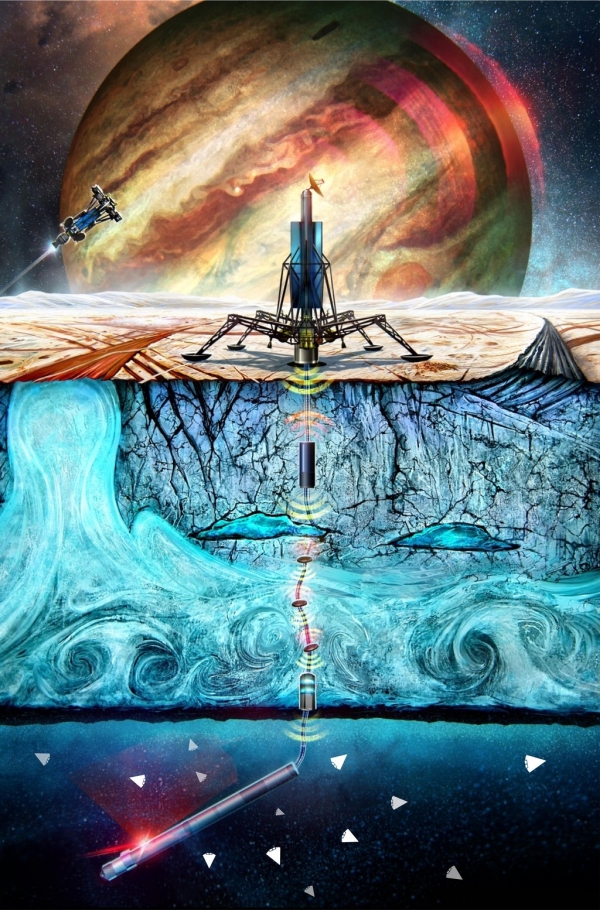
Image: This illustration shows the NASA cryobot concept called Probe using Radioisotopes for Icy Moons Exploration (PRIME) deploying tiny wedge-shaped robots into the ocean miles below a lander on the frozen surface of an ocean world. Credit: NASA/JPL-Caltech.
One of the assumptions built into the SESAME effort is that the surface lander will use one of two nuclear power systems along with whatever technologies are built into the penetration hardware. Thus for the surface cryobot we have the option of a “small fission reactor providing 420 We and 43,000 Wth waste heat” or a “radioisotope power system providing up to 110 We and 2,000 Wth waste heat.” SWIM counts on nuclear waste heat to melt through the ice and also to produce a thermal bubble whose reactions with the ice above could be analyzed in terms of water chemistry.
The robots envisioned here have to be semi-autonomous, each with its own propulsion system, ultrasound communications capability and basic sensors, including chemical sensors to look for biomarkers. Overlapping measurements should allow this ‘flock’ of instrumentation to examine temperature or salinity gradients and more broadly characterize the chemistry of the subsurface water. We’ll follow SWIM with interest not only in the context of Europa but other ocean worlds that may be of astrobiological significance. If life can exist in these conditions, just how much biomass may turn up if we consider all the potential ice-covered oceans on moons and dwarf planets in the Solar System?
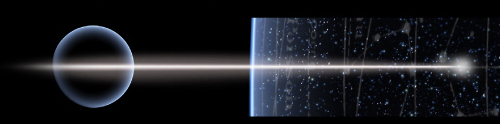
by Paul Gilster | Jun 10, 2022 | Outer Solar System |
I get an eerie feeling when I look at spacecraft before they launch (not that I get many opportunities to do that, at least in person). But seeing the Spirit and Opportunity rovers on the ground at JPL just before their shipment to Florida was an experience that has stayed with me, as I pondered how something built by human hands would soon be exploring another world. I suppose the people who do these things at the Johns Hopkins Applied Physics Laboratory and the Jet Propulsion Laboratory itself get used to the feeling. For me, though, the old-fashioned ‘sense of wonder’ kicks in long and hard, as it did when Europa Clipper arrived recently at JPL.
Not that the spacecraft is by any means complete, but its main body has been delivered to the Pasadena site, where it will see final assembly and testing over a two-year period. Here I fall back on the specs to note that this is the largest NASA spacecraft ever designed for exploration of another planet. It’s about the size of an SUV when stowed for launch, but we know from the James Webb Space Telescope how large these things can become when fully deployed. In Europa Clipper’s case, the recently delivered main body is 3 meters tall and 1.5 meters wide. Extending the solar arrays and other deployable equipment takes it up to basketball court size.
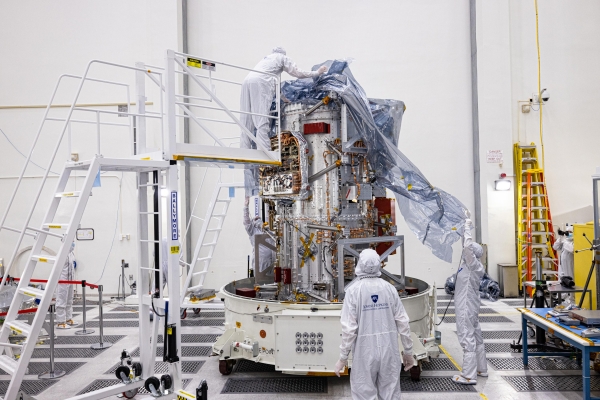
Image: The main body of NASA’s Europa Clipper spacecraft has been delivered to the agency’s Jet Propulsion Laboratory in Southern California, where, over the next two years, engineers and technicians will finish assembling the craft by hand before testing it to make sure it can withstand the journey to Jupiter’s icy moon Europa. Here it is being unwrapped in a main clean room at JPL, as engineers and technicians inspect it just after delivery in early June 2022. Credit: NASA.
Eight antennas are involved, powered by a radio frequency subsystem that will service a high-gain antenna measuring three meters wide, and as JPL notes in a recent update, the electrical wires and connectors collectively called the ‘harness’ themselves weigh 68 kilograms. Stretch all that wiring out and you get 640 meters, taking us twice around a football field. The main body will include a fuel tank and an oxidizer tank connecting to an array of 24 engines. Tim Larson is JPL deputy project manager for Europa Clipper:
“Our engines are dual purpose. We use them for big maneuvers, including when we approach Jupiter and need a large burn to be captured in Jupiter’s orbit. But they’re also designed for smaller maneuvers to manage the attitude of the spacecraft and to fine tune the precision flybys of Europa and other solar system bodies along the way.”
So what is arriving, or has arrived at JPL, is a spacecraft in pieces, its main body now joining key instruments like E-THEMIS, a thermal emission imaging system developed at Arizona State, and Europa-UVS, the mission’s ultraviolet spectrograph. E-THEMIS is an infrared camera that should give us insights into temperatures on the Jovian moon, and hence offer information about its geological activity. Given that we’re interested in finding places where liquid water is close to the surface, the data from this instrument should be extremely valuable during the spacecraft’s nearly fifty close passes.
The theory here is that as Europa’s surface cools after local sunset, the areas of the most solid ice will retain heat longer than areas with a looser, more granular texture. E-THEMIS will be able to map cooling rates across the surface. The infrared camera works in three heat-sensitive bands, and the warmer regions it should see may be the result of liquid water close to the surface, or possible impacts or convection activity. Not surprisingly, E-THEMIS lead project engineer Greg Mehall points to the radiation environment in Jupiter space as one of the team’s biggest issues:
“The extreme radiation environment at Europa gave far more design challenges for the ASU engineering team than on any previous instrument we’ve developed. We had to use dense shielding materials, such as copper-tungsten alloys, to provide the necessary protection from the expected radiation. And to ensure that E-THEMIS will survive during the mission, we also carried out radiation tests on the instrument’s electronic components and materials.”
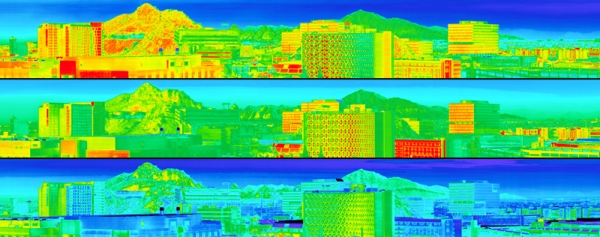
Image: The thermal imager will use infrared light to distinguish warmer regions on Europa’s surface, where liquid water may be near the surface or might have erupted onto the surface. The thermal imager will also characterize surface texture to help scientists understand the small-scale properties of Europa’s surface. In the image above, we’re seeing a diurnal temperature color image from the first light test of Europa Clipper’s thermal imager (called E-THEMIS), taken from the rooftop of the Interdisciplinary Science and Technology Building 4 on the Tempe Campus of Arizona State University (ASU). The top image was acquired at 12:40 PM, the middle at 4:40 PM, and the bottom image at 6:20 PM (after sunset). Temperatures are approximations during this testing phase. Credit: ASU.
As to the Europa-UVS instrument, this ultraviolet spectrograph will search for water vapor plumes and study the composition of both the surface and the tenuous atmosphere as it uses an optical grating to spread and analyze light, identifying basic molecules like hydrogen, oxygen, hydroxide and carbon dioxide.
The spacecraft’s visible light imaging system (EIS) is going to upgrade those well-studied images from the Galileo mission enormously. The plan is to map 90 percent of the moon’s surface at 100 meters per pixel, which is six times more of Europa’s surface than Galileo, and at five times better resolution. And when Europa Clipper swings close to Europa during a flyby, it will produce images with a resolution fully 100 times better than Galileo. The Europa Imaging System includes both wide- and narrow-angle cameras, each with an eight-megapixel sensor. Both of these cameras will produce stereoscopic images and include the needed filters to acquire color images.
All told, the spacecraft’s nine science instruments should be able to extract information about the depth and salinity of the ocean under the ice and, crucially, the thickness of the ice crust (I can imagine wagers on that issue going around in certain quarters). Gathering information about the moon’s surface and interior should further illuminate the issue of plumes from the ocean below that may break through the ice.
Assembly, test and launch is a two year phase that, by the end of this year, should see assembly of most of the flight hardware and the remaining science instruments. Kudos to JHU/APL, which has just delivered a flight system that is the largest ever built by engineers and technicians there. Now we look toward bolting on the radio frequency module, radiation monitors, power converters, the propulsion electronics and those hundreds of meters of wiring. Not to mention the electronics vault that must stand up to hard radiation.
The full instrument package will include an imaging spectrometer, ice-penetrating radar, a magnetometer, a plasma instrument, a mass spectrometer and a dust analyzer. Only two years and four months before launch onto a six-year journey of 2.9 billion kilometers. Europa Clipper isn’t a life-finder, but it does have the capability of detecting whether the moon’s ocean really does allow for the possibility of life to develop. It’s our first reconnaissance of Europa since the 1990s. What surprises will it reveal?
Bear in mind, too, that we still have ESA’s JUICE (JUpiter ICy moons Explorer) in the offing, with launch planned for 2023. I note with interest that on June 19, Europa will occult a distant star, which should be useful in tweaking our knowledge of the moon’s orbit before the arrival of both missions. Destined to end its life as a Ganymede orbiter, JUICE will make only two close passes of Europa, but its period of operations will coincide with part of Europa Clipper’s numerous flybys of the moon.
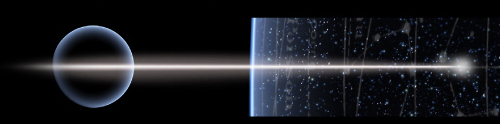
by Paul Gilster | Apr 22, 2022 | Outer Solar System |
I’m always interested in studies that cut across conventional boundaries, capturing new insights by applying data from what had appeared, at first glance, to be unrelated disciplines. Thus the news that the ice shell of Europa may turn out to be far more dynamic than we have previously considered is interesting in itself, given the implications for life in the Jovian moon’s ocean, but also compelling because it draws on a study that focused on Greenland and originally sought to measure climate change.
The background here is that the Galileo mission that gave us our best views of Europa’s surface so far showed us that there are ‘double ridges’ on the moon. In fact, these ridge pairs flanked by a trough running between them are among the most common landforms on a surface packed with troughs, bands and chaos terrain. The researchers, led by Stanford PhD student Riley Culberg, found them oddly familiar. Culberg, whose field is electrical engineering (that multidisciplinary effect again) found an analog in a similar double ridge in Greenland, which had turned up in ice-penetrating radar data.
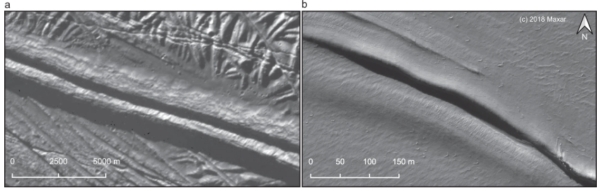
Image: This is Figure 1 from the paper. Caption: a Europan double ridge in a panchromatic image from the Galileo mission (image PIA00589). The ground sample distance is 20?m/pixel. b Greenland double ridge in an orthorectified panchromatic image from the WorldView-3 satellite taken in July 2018 (© 2018, Maxar). The ground sample distance is ~0.31?m/pixel. Signatures of flexure are visible along the ridge flanks, consistent with previous models for double ridges underlain by shallow sills. Credit: Culberg et al.
The feature in Greenland’s northwestern ice sheet has an ‘M’-shaped crest, possibly a version in miniature of the double ridges we see on Europa. The climate change work used airborne instrumentation producing topographical and ice-penetrating radar data via NASA’s Operation IceBridge, which studies the behavior of polar ice sheets over time and their contribution to sea level rise. Where this gets particularly interesting is that flowing ice sheets produce such things as lakes beneath glaciers, drainage conduits and surface melt ponds. Figuring out how and when these occur becomes a necessary part of working with the dynamics of ice sheets.
The mechanism in play, analyzed in the paper, involves ice fracturing around a pocket of pressurized liquid water that was refreezing inside the ice sheet, creating the distinctive twin peak shape. Culberg notes that the link between Greenland and Europa came as a surprise:
“We were working on something totally different related to climate change and its impact on the surface of Greenland when we saw these tiny double ridges – and we were able to see the ridges go from ‘not formed’ to ‘formed… In Greenland, this double ridge formed in a place where water from surface lakes and streams frequently drains into the near-surface and refreezes. One way that similar shallow water pockets could form on Europa might be through water from the subsurface ocean being forced up into the ice shell through fractures – and that would suggest there could be a reasonable amount of exchange happening inside of the ice shell.”
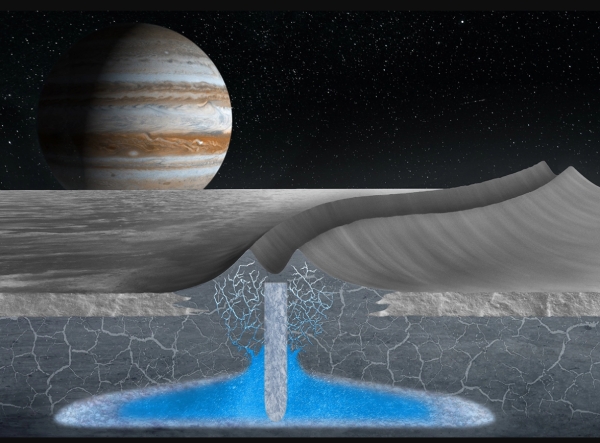
Image: This artist’s conception shows how double ridges on the surface of Jupiter’s moon Europa may form over shallow, refreezing water pockets within the ice shell. This mechanism is based on the study of an analogous double ridge feature found on Earth’s Greenland Ice Sheet. Credit: Justice Blaine Wainwright.
The double ridges on Europa can be dramatic, reaching nearly 300 meters at their crests, with valleys a kilometer wide between them. The idea of a dynamic ice shell is supported by evidence of water plumes erupting to the surface. Thinking about the shell as a place where geological and hydrological processes are regular events, we can see that exchanges between the subsurface ocean and the possible nutrients accumulating on the surface may occur. The mechanism, say the researchers, is complex, but the Greenland example provides the model, an analog that illuminates what may be happening far from home. It also provides a radar signature that future spacecraft should be able to search for.
From the paper:
Altogether, our observations provide a mechanism for subsurface water control of double ridge formation that is broadly consistent with the current understanding of Europa’s ice-shell dynamics and double ridge morphology. If this mechanism controls double ridge formation at Europa, the ubiquity of double ridges on the surface implies that liquid water is and has been a pervasive feature within the brittle lid of the ice shell, suggesting that shallow water processes may be even more dominant in shaping Europa’s dynamics, surface morphology, and habitability than previously thought.
So we have a terrestrial analog of a pervasive Europan feature, providing us with a hypothesis we can investigate with instruments aboard both Europa Clipper and the ESA’s JUICE mission (Jupiter Icy Moons Explorer), launching in 2024 and 2023 respectively. Confirming this mechanism on Europa would go a long way toward moving the Jovian moon still further up our list of potential life-bearing worlds.
The paper is Culberg et al., “Double ridge formation over shallow water sills on Jupiter’s moon Europa,” Nature Communications 13, 2007 (2022). Full text.
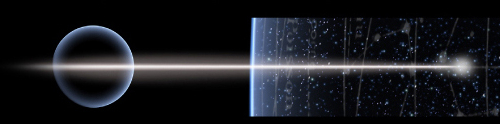
by Paul Gilster | Dec 9, 2021 | Outer Solar System |
A spray of organic molecules and ice particles bursting out of an outer system moon is an unforgettable sight, as Cassini showed us at Enceladus. Finding something similar at Europa would be a major help for future missions there, given the opportunity to sample a subsurface ocean that is perhaps as deep as 160 kilometers. But Lynnae Quick (NASA GSFC), who works on the science team that produced the Europa Imaging System cameras that will fly on the Europa Clipper mission, offers a cautionary note:
“A lot of people think Europa is going to be Enceladus 2.0, with plumes constantly spraying from the surface. But we can’t look at it that way; Europa is a totally different beast.”
A good thing that Europa Clipper can produce evidence of conditions beneath the ice without the need for plumes when it begins its explorations in 2031. In fact, adds Quick, every instrument aboard the spacecraft has its own role to play in the study of that global ocean. Still, potential plumes are too important to ignore, even if finding an active, erupting Europa would by no means be as straightforward as discovering the plumes of Enceladus. The Europa evidence we have indicates faint plume activity through Galileo and Hubble data and some Earth-based telescopes.
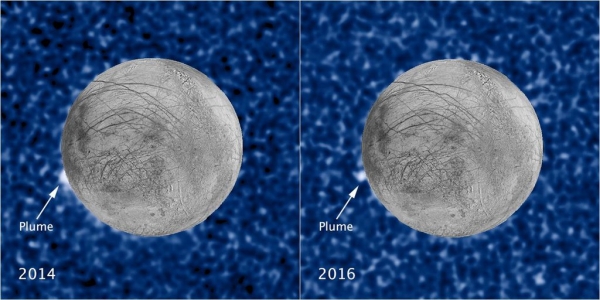
Image: These composite images show a suspected plume of material erupting two years apart from the same location on Jupiter’s icy moon Europa. The images bolster evidence that the plumes are a real phenomenon, flaring up intermittently in the same region on the satellite. Both plumes, photographed in ultraviolet light by NASA’s Hubble’s Space Telescope Imaging Spectrograph, were seen in silhouette as the moon passed in front of Jupiter. Credit: NASA/JPL.
In the image above, notice the possible plume activity. At left is a 2014 event that appears in Hubble data, a plume estimated to be 50 kilometers high. At the right, and in the same location, is an image taken two years later by the same Hubble Imaging Spectrograph, both events seen in silhouette as the moon passed in front of Jupiter. It’s noteworthy that this activity occurs at the same location as an unusually warm spot in the ice crust that turned up in Galileo mission data from the 1990s.
Let’s now cut to a second image, showing that Galileo find. Below we see the surface of Europa, focusing on what NASA calls a ‘region of interest.’
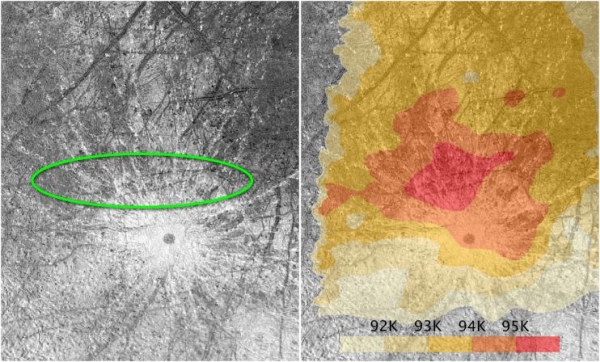
Image: The image at left traces the location of the erupting plumes of material, observed by NASA’s Hubble Space Telescope in 2014 and again in 2016. The plumes are located inside the area surrounded by the green oval. The green oval also corresponds to a warm region on Europa’s surface, as identified by the temperature map at right. The map is based on observations by the Galileo spacecraft. The warmest area is colored bright red. Researchers speculate these data offer circumstantial evidence for unusual activity that may be related to a subsurface ocean on Europa. The dark circle just below center in both images is a crater and is not thought to be related to the warm spot or the plume activity. Credit:
NASA/ESA/W. Sparks (STScI)/USGS Astrogeology Science Center.
Getting access to the realm below the surface would obviate the need to drill through kilometers of ice in some future mission, giving us a better understanding of possible habitability. An ocean churned by activity from heated rock below the seafloor could spawn the kind of life we find around hydrothermal vents here on Earth, circulating carbon, hydrogen, oxygen, nitrogen, phosphorus, and sulfur deep within. Moreover, Europa is in an elliptical orbit that generates internal heat and likely drives geology.
Does an icy plate tectonics also exist on this moon? The Europan surface is laced with cracks and ridgelines, with surface blocks having apparently shifted. Bands that show up in Galileo imagery delineate zones where fresh material from the underlying shell appears to have moved up to fill gaps as soon as they appear. A 2014 paper (citation below) by Simon Kattenhorn (University of Idaho – Moscow) and Louise Prockter (JHU/APL) found evidence of subduction in Galileo imagery, where one icy plate seems to have moved beneath another, forcing surface material into the interior.
That paper is, in fact, worth a quote. The italics are mine:
…we produce a tectonic reconstruction of geologic features across a 134,000 km2 region of Europa and find, in addition to dilational band spreading, evidence for transform motions along prominent strike-slip faults, as well as the removal of approximately 20,000 km2 of the surface along a discrete tabular zone. We interpret this zone as a subduction-like convergent boundary that abruptly truncates older geological features and is flanked by potential cryolavas on the overriding ice. We propose that Europa’s ice shell has a brittle, mobile, plate-like system above convecting warmer ice. Hence, Europa may be the only Solar System body other than Earth to exhibit a system of plate tectonics.
This is an encouraging scenario in which surface nutrients produced through interactions with radiation from Jupiter are driven into pockets in the ice shell and perhaps into the ocean below, even as chemical activity continues at the seafloor. If we find plumes, their chemical makeup could put these scenarios to the test. But as opposed to the highly visible plumes of Enceladus, any Europan plumes would be harder to detect, bound more tightly to the surface because of the higher Europan gravity, and certainly lacking the spectacular visual effects at Enceladus.
Key to the search for plumes will be Clipper’s EIS camera suite, which can scout for activity at the surface by scanning the limb of the moon as it passes in front of Jupiter. Moreover, a plume should leave a deposit on surface ice that EIS may see. Clipper’s Europa Ultraviolet Spectrograph (Europa-UVS) will look for plumes at the UV end of the spectrum, tracking the chemical makeup of any that are detected. The Europa Thermal Emission Imaging System (E-THEMIS) will be able to track hotspots that may indicate recent eruptions. A complete description of Clipper’s instrument suite is available here.
We’ve been using Galileo data for a long time now. It’s a refreshing thought that we’ll have two spacecraft – Europa Clipper and Jupiter Icy Moons Explorer (JUICE) – in place in ten years to produce what will surely be a flood of new discoveries.
The paper on Europan plate tectonics is Kattenhorn & Prockter, “Evidence for subduction in the ice shell of Europa,” Nature Geoscience 7 (2014), 762-767 (abstract).
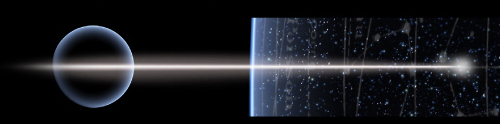
by Paul Gilster | Oct 20, 2021 | Outer Solar System |
Juno has worked wonders for our knowledge of Jupiter, but we continue to rely on Hubble observations and still helpful imagery from Galileo as we study the giant planet’s intriguing moon Europa, anticipating the arrival of Europa Clipper and the European Space Agency’s Jupiter Icy Moons Explorer (JUICE) at the end of the decade. The great question is whether life can exist beneath the ice, and the evidence of plume activity, first found in 2013 in Hubble data, is encouraging in some ways more than others. A mission to Saturn’s moon Enceladus can rely on geysers in a particular place — the Tiger Stripes at the south pole — and on geysers that are frequent. At Europa, predicting where and when a plume will burst forth is all but a black art. How do we sample a Europan plume?
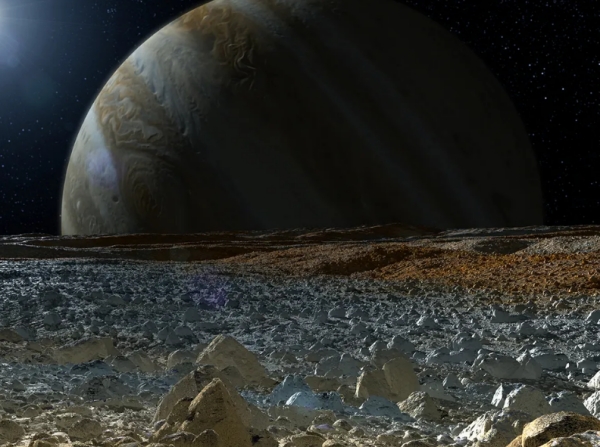
Image: Will we one day see Europa through the eyes of a (well-shielded) lander? Credit: NASA.
While contemplating that question, we push ahead with other analyses that help us characterize this unusual place. A team led by Lorenz Roth (KTH Royal Institute of Technology, Space and Plasma Physics, Sweden) has produced evidence from Hubble that a persistent fraction of water vapor exists within Europa’s atmosphere, what little of it there is (this is an atmosphere with one-billionth the surface pressure of the atmosphere on our planet). Here we are talking not about plumes but processes including sublimation, where surface ices transition directly to vapor. It’s a finding that feeds into planning for those upcoming Jupiter missions and also informs us more deeply about how icy moons react to this high radiation environment.
The paper on this work, which appeared in Geophysical Research Letters (citation below), reinforces the contrast between plume activity and persistent vapor:
A key finding of this study is the consistency in the detection of the reduced oxygen emission ratio on the trailing hemisphere disk center and the overall stability of the ratio profiles in all images with similar geometry. In particular, the oxygen emission ratios in center and limb regions in the four trailing side visits, which were obtained in 1999, 2012, and 2015 and are all consistent within uncertainties. This means, they are diagnostic for persistent atmospheric properties, in stark contrast to the apparent transient nature of detected features that were interpreted to relate to H2O plumes (Paganini et al., 2019; Roth et al., 2014b).
The transition of ice to water vapor raises questions. Water vapor on Europa seems to be limited to the moon’s trailing hemisphere, the part of the moon that remains opposite to its direction of motion along its orbit. The cause of the asymmetry between leading and trailing hemispheres is intriguing given that we find the same phenomenon on Ganymede as well.
The paper also notes that the ratio of H2O relative to O2 is similar on the trailing hemispheres of both Europa and Ganymede. I should note before going further that the detection of water vapor on Ganymede came from Roth’s use of the technique he here uses on Europa, as discussed in Nature Astronomy earlier this year.
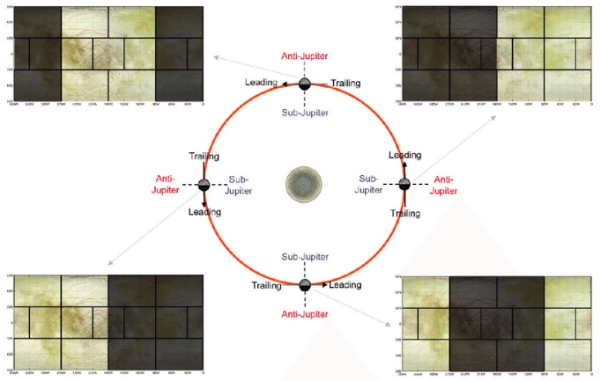
Image: Since Europa is tidally locked, Europa’s terrain maintains the same orientation relative to Jupiter (center image). The notional Jupiter centered plot viewed from Jupiter’s north pole in the rotating frame with the y-axis points to the Sun, z-axis normal to the Jupiter orbit plane, and x-axis completing the right rule. Also shown are maps of Europa showing the fourteen roughly equal-area regions delineated by the Europa Science Definition Team (SDT) and the surface illumination around Jupiter. Credit: Lam et al., from “A Robust Mission Tour for NASA’s Planned Europa Clipper Mission,” 7 January 2018 / AIAA Space Flight Mechanics Meeting 2018.
How fully do we understand the production of water vapor in the Europan environment? Consider this snip from the paper. It points not only to sublimation, but also to the phenomenon known as ‘sputtering,’ in which surface particles are bombarded by energetic plasma. Sputtering is considered an alternative source for at least some of the H2O found on Europa’s trailing hemisphere, especially given the modeling of plasma discussed in this paper:
The trailing hemisphere [of Europa] also coincides with the plasma upstream hemisphere, where most of the thermal plasma impinges on Europa’s surface according to modeling (Cassidy et al., 2013; Pospieszalska & Johnson, 1989). In addition, the sputtering yield (amount of neutrals ejected per incident charged particle flux) also increases with surface temperature (Famá et al., 2008), further favoring the trailing hemisphere independent of the distribution of the incident flux…. For both sources [Europa and Ganymede], however, modeled H2O abundances are often significantly lower than our derived value on the trailing side.
Roth’s datasets were produced in Hubble ultraviolet observations using the instrument’s Space Telescope Imaging Spectrograph (STIS). There is still much for the next two spacecraft to investigate in terms of water vapor at Europa as we examine the difference between leading and trailing day-side hemispheres, but the exact mechanism remains unresolved:
Putting the main results in a nutshell, oxygen emission ratios found in HST observations suggest a persistent H2O atmosphere above Europa’s trailing hemisphere, but the source of the water vapor cannot [be] unambiguously identified.
The paper is Roth, “A Stable H2O Atmosphere on Europa’s Trailing Hemisphere From HST Images,” Geophysical Research Letters Vol. 48, Issue 20 (28 October 2021). Published online 13 September 2021. Full text. The paper on Ganymede is Roth et al., “A sublimated water atmosphere on Ganymede detected from Hubble Space Telescope observations,” Nature Astronomy 5 (26 July 2021), 1043-1051 (abstract),
by Paul Gilster | Sep 20, 2021 | Outer Solar System |
Something interesting always comes out of the Europlanet Science Congress (probably better known to Centauri Dreams readers under its former name, the European Planetary Science Congress), and this year is no exception. This is the largest planetary science meeting in Europe, normally drawing about 1000 participants, though last year and this year as well have been virtual meetings, the latter ongoing as I write this and running until September 24.
As the conference proceeds, my eye was drawn to a study by Tristan Guillot on the ice giants Uranus and Neptune, targets of (let’s hope) future space missions that can help us resolve the differences between this class of world and gas giants like Jupiter and Saturn. Guillot (CNRS, Laboratoire Lagrange, Nice) targets the odd fact that both of these planets have recently been found to be deficient in ammonia in their atmospheres as compared to the gas giants. Astronomers are puzzled because other compounds such as methane, found in the primordial cloud as the young system coalesced, are clearly there in abundance.
Let’s pause briefly on this point. We’ve talked often about the ‘snowline,’ beyond which materials like methane, ammonia and other trace compounds — ‘ices’ in astronomical parlance — transition from being gases to solids during system formation. The ice giants differ from the primarily hydrogen and helium-based gas giants in being mostly composed of ices beyond the snowline during formation. Recent work at infrared and radio wavelengths indicating the lack of ammonia at Uranus and Neptune thus needs an explanation. The current formation model suggests it should be there.
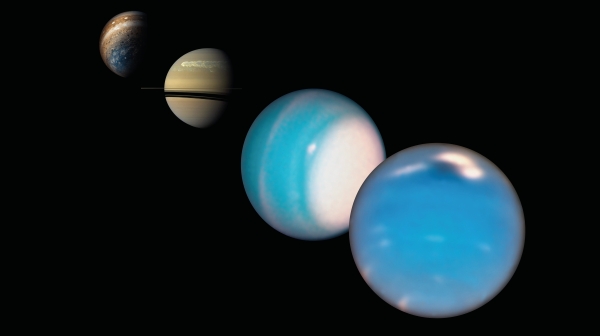
Image: Composite image of Neptune, Uranus, Saturn and Jupiter. Credits: Jupiter from Juno: NASA/SwRI/MSSS/Gerald Eichsta?dt/Sea?n Doran; Saturn from Cassini: NASA/JPL-Caltech/Space Science Institute; Uranus and Neptune from HST: NASA/ESA/A. Simon (NASA Goddard Space Flight Center), and M.H. Wong and A. Hsu (University of California, Berkeley).
Guillot proposes that the ammonia is actually present at both planets in the form of slushy hailstones of ammonia and water, which would carry it far below the limits of detection within the ice giant atmospheres. The scientist is drawing on data from the highly productive Juno mission to Jupiter, where ammonia turns up at deeper levels than expected because of similar ‘hailstone’ formation.
Is the process common in the outer planets? The Juno data have revealed ammonia-water hailstones forming rapidly in Jupiter’s atmosphere during storms, a fact Guillot attributes to ammonia’s ability to liquefy water ice crystals at low temperatures. Models drawing on the Juno findings make these hailstones out to be up to a kilogram or more in weight, larger than we find on Earth, and they act as a transport mechanism for moving ammonia well below the cloud base. However, at Jupiter the effect is more confined. Says Guillot:
“Thermodynamic chemistry implies that this process is even more efficient in Uranus and Neptune, and the mushball seed region is extended and occurs at greater depths. Thus, ammonia is probably simply hidden in the deep atmospheres of these planets, beyond the reach of present-day instruments.”
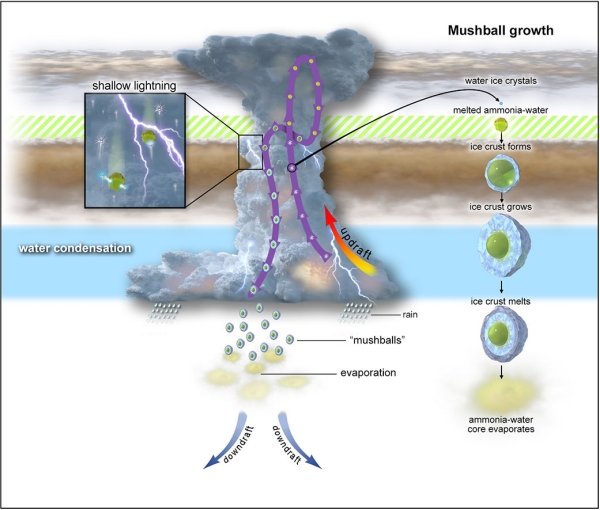
Image: Artist’s impression showing how mushballs form in giant planets’ atmospheres. Credit: NASA/JPL-Caltech/SwRI/CNRS.
The Juno findings become a plausible solution to the ammonia deficit at Uranus and Neptune, but here we run into the limits of our instrumentation. Just how deep is the ammonia taken by this process? Guillot is hardly the first to point to the need for a dedicated mission, preferably two, in the form of orbiters for each of the ice giants. Only then will we begin to get a handle on mixing in hydrogen atmospheres in the interesting realm of ice giants, which are turning up in many other stellar systems as well.
Guillot adds: “Neptune and Uranus are a critical link between giant planets, like Jupiter and Saturn, and ice giant exoplanets that we are discovering in the galaxy. We really need to go there!”
Dr. Guillot’s paper is still in preparation. The reference for today is Guillot, “Mushballs and the lack of Ammonia in Uranus and Neptune,” Europlanet Science Congress 2021, online, 13–24 Sep 2021, EPSC2021-422. https://meetingorganizer.copernicus.org/EPSC2021/session/41678#Oral_and_Poster_presentations_and_abstracts
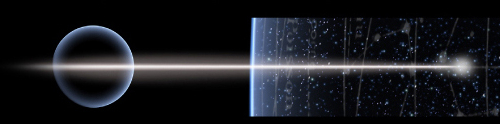